Tricuspid Valve Disorders: Atresia, Dysplasia, and Ebstein Anomaly
Frank Cetta
Joseph A. Dearani
Patrick W. O’Leary
David J. Driscoll
Tricuspid Valve Atresia
Tricuspid valve atresia is one of the more common form of cyanotic congenital heart disease and constitutes 2.7% of all congenital heart disease (1). The prevalence in autopsy series is 2.9% and it occurs in approximately 1:15,000 live births (2,3,4). It consists of complete agenesis of the tricuspid valve and absence of a direct communication between the right atrium (RA) and the right ventricle (RV). The survival of newborns with tricuspid atresia depends upon the presence of an atrial septal defect (ASD) to allow egress of blood from the RA to the left atrium (LA).
Pathology
Development of the tricuspid valve is related to the sinus (inlet) portion of the RV. In tricuspid valve atresia the inlet portion of the RV is absent and the infundibular portion is developed (except with coexistent pulmonary valve atresia). The development/size of the trabecular portion of the RV is variable (depends on presence/size of a VSD). The pathogenesis of tricuspid valve atresia is unknown. In most hearts with tricuspid atresia, the tricuspid inlet is a dimple in the floor of the RA and appears as a fibromuscular ledge (Fig. 38.1). More rare forms of tricuspid atresia have partially delaminated but fused leaflets, and appear as membranes or resemble some features of Ebstein anomaly.
In 1906, Kuhne initially categorized tricuspid valve atresia (5). The classification scheme was later modified into three types based on the great artery relationship: Type I, normally related great arteries (70% to 80% of patients); Type II, transposed great arteries (12% to 25%); and Type III, congenitally corrected transposition of the great arteries (3% to 6%). The three subtypes are further classified according to the presence and size of the ventricular septal defect (VSD) and the presence of pulmonary atresia or stenosis (6,7) (Table 38.1).
Since there is no flow from the RA to the RV, an ASD allows egress of blood from the RA to the LA. Rarely is the ASD restrictive or absent. Additional cardiovascular abnormalities occur in 18% of patients with normally related great arteries and in 63% of patients with transposed great arteries. These include coarctation of the aorta, patent ductus arteriosus (PDA), and right aortic arch. Extracardiac anomalies occur in 20% of cases.
TABLE 38.1 Classification of Tricuspid Atresia | ||||||||||||||||||||
---|---|---|---|---|---|---|---|---|---|---|---|---|---|---|---|---|---|---|---|---|
|
Genetics
Tricuspid atresia has been associated with microdeletions of 22q11, and trisomies 13, 18, and 21 (8,9,10). The likelihood of a microdeletion of 22q11 in patients with tricuspid atresia is about 7%. Cases of tricuspid atresia have been associated with mutations on chromosomes 3, 4, and 8 (11). In mice, inactivation of the Zfpm2 gene causes cardiac defects that include tricuspid atresia (12). In addition to Zfpm2 inactivation, tricuspid atresia has been associated with mutations of NKX2 and HEY2. Two growth factor pathways that act as upstream regulators of epithelial–mesenchymal transformation are the TGF-β/BMP and Notch pathways. Downstream targets of these pathways include transcription factors such as NKX2–5, TBX5, NFATC1, GATA4, and SOX9 and these are associated with tricuspid atresia in animal models. The TGF-β/BMP pathway with its GATA4/ZFPM2 downstream target and the Notch pathway with its Hey2 downstream target may be important in the development of tricuspid atresia (13,14,15).
Hemodynamics
In tricuspid atresia, hemodynamics are determined by the presence or absence of pulmonary valve atresia, the severity of subpulmonary/pulmonary stenosis, relationship of the great arteries, and presence of subaortic obstruction. Because of mixing in the LA, the blood oxygen saturation reaching the left ventricle (LV), and subsequently the aorta, depends on the relative volumes of systemic and pulmonary venous return. Patients with tricuspid atresia and increased pulmonary blood flow (no pulmonary/subpulmonary stenosis) have a larger volume of pulmonary venous return than systemic venous return and relatively high systemic arterial oxygen saturation. In contrast, patients with low pulmonary blood flow (due to pulmonary/subpulmonary stenosis or pulmonary atresia) may have marked systemic arterial hypoxemia. The volume of pulmonary blood flow and the clinical characteristics may change as the child grows. Patients with pulmonary stenosis may depend on the PDA for adequate pulmonary blood flow; as the PDA closes, pulmonary blood flow may decrease.
In general, those with normally related great arteries will demonstrate pulmonary or subpulmonary (at the VSD) obstruction that is progressive. In contrast, most patients with tricuspid atresia and transposed great arteries (type II-C) have unobstructed pulmonary blood flow. Patients in the former group usually have more marked hypoxemia than those with transposed great arteries. However, those with transposed great arteries are more likely to have pulmonary edema, congestive heart failure, and can develop pulmonary vascular obstructive disease. The VSD associated with tricuspid atresia has a completely muscular circumference and tends to become smaller over time. A restrictive VSD associated with normally related great arteries produces progressive subpulmonary stenosis and increasing hypoxemia. A restrictive VSD associated with transposed great arteries produces subaortic obstruction. When subaortic obstruction coexists with a pulmonary artery band, LV hypertension and hypertrophy may occur leading to fibrosis and myocardial dysfunction.
Clinical Findings
History
Historically, patients with tricuspid valve atresia came to attention because of cyanosis, signs/symptoms of congestive heart failure, or growth failure. Diagnosis in the current era frequently is made in utero with fetal echocardiography. PDA closure in those with pulmonary atresia or critical pulmonary stenosis produces profound cyanosis, arterial hypoxemia, and death. Cyanosis is more variable in those with lesser degrees of pulmonary stenosis but hypercyanotic spells can occur as subpulmonary stenosis progresses. For patients with unobstructed pulmonary blood flow, signs and symptoms of congestive heart failure and pulmonary edema develop as pulmonary vascular resistance decreases. Without surgical intervention, pulmonary vascular obstructive disease occurs in patients with unrestricted pulmonary blood flow (types I-C and II-C). Pulmonary vascular obstructive disease is much less common in patients with normally related great arteries than in patients with transposed great arteries.
Bacterial endocarditis and brain abscess are relatively common complications of tricuspid atresia. Neurologic complications also result from intravascular thrombosis and paradoxical embolic phenomena, especially prior to Fontan palliation.
Physical Examination
Cyanosis is the most common clinical feature of tricuspid atresia. Infants with tricuspid atresia and normally related great arteries may have excessive pulmonary blood flow and little cyanosis. Their degree of cyanosis may increase as the VSD becomes more restrictive, causing subpulmonary stenosis and decreased pulmonary blood flow.
Newborns with tricuspid atresia usually have normal birth weight. The RV impulse may be diminished. In an older infant, a thrill may be present if the VSD is restrictive or there is severe pulmonary/subpulmonary stenosis. The first heart sound is single and may be accentuated. The intensity of the second heart sound usually is normal if the great arteries are normally related and pulmonary artery pressure is normal. But when the great arteries are transposed, the aorta is closer to the anterior chest wall and the second heart sound may be single or louder despite normal pulmonary artery pressure.
Cardiac murmurs are present in 80% of patients with tricuspid atresia. A low-frequency holosystolic murmur is present in those with a small VSD. Sometimes, a crescendo–decrescendo murmur is produced by the flow of blood through the VSD. A systolic midfrequency crescendo–decrescendo murmur is present in patients with pulmonary stenosis. Patients with pulmonary atresia and collateral blood supply and patients who had a surgical systemic-to-pulmonary arterial shunt have continuous murmurs. A third heart sound or a diastolic mitral inflow murmur may be audible in patients with excessive pulmonary blood flow.
Hepatomegaly may occur in the rare instance of a restrictive ASD. In older children with persistent cyanosis, clubbing will be present. Pulses are expected to be normal and symmetrical except with coexistent coarctation of the aorta.
Electrocardiography
First-degree atrioventricular (AV) block occurs in 15% of cases and presumably is due to prolonged atrial conduction, since AV node function usually is normal. Early origin of the left bundle from the common bundle causes the frontal plane QRS axis to shift leftward or superior. The frontal plane electrocardiographic (ECG) loop is counterclockwise. Rarely, the frontal plane QRS axis is normal, and this suggests the presence of transposed great arteries. The RV forces are diminished, and there is evidence of LV hypertrophy and discordant QRS and T waves. A short PR interval with slurred initial portion of the QRS is common and may be misinterpreted as pre-excitation (16,17) (Fig 38.2).
Chest Radiography
The cardiac silhouette usually is normal in neonates and infants with tricuspid atresia. The right heart border may be prominent, reflecting enlargement of the RA. Heart size increases in those with unrestricted pulmonary blood flow. Pulmonary vascular markings
are prominent when blood flow is excessive. In 80% of patients with tricuspid atresia, pulmonary blood flow is diminished and vascular markings are decreased.
are prominent when blood flow is excessive. In 80% of patients with tricuspid atresia, pulmonary blood flow is diminished and vascular markings are decreased.
Echocardiography
The echocardiographic assessment of tricuspid valve atresia begins in utero (18). The two-dimensional four-chamber view readily demonstrates the absence of a tricuspid valve and discordant ventricular cavity sizes (LV > RV). Incremental information is obtained from pulse wave and color Doppler imaging that fail to demonstrate flow across the tricuspid inlet. The RV will be small, although the outlet portion can reach nearly normal size if there is a large VSD. It is important during the fetal assessment to completely understand the orientation of the great arteries (normal or transposed) the degree of outflow obstruction (if any) and the presence of a VSD. Pulmonary valve atresia must be excluded. The presence of retrograde aortic arch or ductal flow indicates inadequate perfusion from the corresponding outflow tract and should lead to “prophylactic” institution of a prostaglandin infusion shortly after delivery. The fetus with disproportionate great artery sizes should also receive early prostaglandin therapy. Interrogation of the atrial septum will demonstrate an obligatory right-to-left shunt. In the rare cases of a restrictive ASD, the RA and hepatic veins are severely dilated. Patients with tricuspid valve disease frequently have a redundant Eustachian valve. It is important not to confuse the redundant Eustachian valve with the interatrial septum, this leads to misidentification of ASDs.
In the neonate, a comprehensive echocardiographic assessment with attention to the absent right AV connection, orientation of the great arteries, presence/absence of a VSD, size of the RV, atrial shunt, and presence of pulmonary/subpulmonary/subaortic obstruction are important features (Fig. 38.3). Subcostal scans/sweeps that are perpendicular to the plane of the atrial septum should be performed in the four-chamber (coronal) and bicaval sagittal views. These views offer the best visualization of the atrial septum and sizing of the ASD. An RA to LA gradient may be obtained with Doppler interrogation of the flow across the atrial septum.
Evaluation of the great arteries should be performed from multiple imaging planes. The ventricular septum should also be assessed in orthogonal views. Left juxtaposition of the RA appendage may occur in tricuspid atresia and it can be visualized in both the subcostal coronal plane as well as the parasternal long axis window. It is important to differentiate the orifice of a juxtaposed RA appendage from an ASD. The atrial septum will lie in a more horizontal plane due to the distortion from the abnormal position of the RA appendage.
As an infant grows it is important to serially evaluate flow through the VSD since it may become restrictive. In addition, muscular ridges or membranes can obstruct flow through the VSD. A restrictive VSD will have implications for either pulmonary or systemic flow depending on whether the great arteries are normally related or transposed. Aortic arch abnormalities must be excluded especially in the situation of transposed great arteries with a restrictive VSD. The PDA should be evaluated from the suprasternal and high left parasternal short axis views.
Cardiac Catheterization
In the current era, the diagnosis of tricuspid atresia and the delineation of associated anomalies can be accomplished noninvasively with echocardiography. Cardiac catheterization may be necessary in infants to measure pulmonary artery pressure and resistance; as a guide to the need for pulmonary artery banding to prevent development of pulmonary vascular obstructive disease. Very rarely, cardiac catheterization is necessary in order to perform a balloon atrial septostomy in a newborn with a restrictive ASD. Later in childhood, cardiac catheterization may be needed to assess pulmonary pressure/resistance and ventricular end-diastolic pressure in preparation for a Fontan operation. Some centers do not routinely perform cardiac catheterization in uncomplicated patients with tricuspid atresia. These patients
are evaluated with MRI or CT in addition to echocardiography prior to Fontan.
are evaluated with MRI or CT in addition to echocardiography prior to Fontan.
In 2010, the Boston Children’s Hospital group demonstrated that in 51% of patients who had cardiac catheterization prior to Fontan, no incremental clinical information was added by the procedure (19). In their study, cardiac catheterization hemodynamic data were not associated with early postoperative outcomes. However, important interventions were performed in 37% of patients. Minor complications occurred in 29% of patients and major complications occurred in 2%. Preoperative surface echocardiography alone did not demonstrate relevant anatomy in 2/3 of patients (usually because of inadequate imaging of the pulmonary arteries). However, this study included many patients with more complex anatomy than tricuspid atresia.
Clinical Management
Infants
Four considerations should guide the management of infants with tricuspid atresia:
In patients with cyanosis: decrease hypoxemia by increasing pulmonary flow with shunts (systemic-to-pulmonary artery in a neonate, bidirectional cavopulmonary anastomosis (BDCPA) in an older infant).
In patients with unrestricted pulmonary blood flow: procedures that will ensure adequate low pressure pulmonary flow (pulmonary artery band in select cases, BDCPA).
Preservation of myocardial function by eliminating systemic outflow obstruction and providing adequate low pressure pulmonary flow that preserves the pulmonary vascular integrity to optimize conditions for later Fontan operation. In cases with transposed great arteries this may require VSD enlargement or a Damus–Kaye–Stansel (DKS) anastomosis with a systemic-to-pulmonary artery shunt.
Reduce risks of bacterial endocarditis and thromboembolism.
Newborns with prenatal evidence of severe outflow obstruction and those demonstrating severe hypoxemia and acidosis should be treated promptly with infusion of prostaglandin E1 to maintain patency of the PDA. Infants with transposed great arteries and unrestricted pulmonary blood flow may have signs and symptoms of pulmonary edema and congestive heart failure. These patients will benefit from treatment with diuretics. Historically, these patients had a pulmonary artery band placed to decrease pulmonary flow. However, pulmonary artery banding may accelerate VSD closure. In tricuspid atresia with transposed great arteries, subaortic obstruction occurs and leads to marked LV hypertrophy and fibrosis. Ventricular hypertrophy is an adverse risk factor for subsequent Fontan operation. Therefore, alternative surgical procedures, other than pulmonary artery banding, that limit pulmonary flow and bypass potential areas of subaortic obstruction have been recommended (DKS operation).
Children and Adolescents
Before 1971, palliative procedures to control pulmonary blood flow (pulmonary artery band, systemic-to-pulmonary artery shunt, or superior vena cava to pulmonary artery anastomosis)
were the mainstay of surgical treatment for patients with tricuspid valve atresia. Unoperated patients with tricuspid atresia and cyanosis had a 1-year mortality of 90% (20). In 1971, Fontan and associates described a unique procedure to separate the systemic and pulmonary venous returns, eliminate the right-to-left intracardiac shunt and reduce ventricular volume overload (21,22,23). The components of the original Fontan operation consisted of the following: (1) classic Glenn anastomosis to direct superior vena cava flow to the right lung, (2) redirection of inferior vena cava flow to the pulmonary artery with a valved connection of the RA to the pulmonary artery, (3) insertion of a valve into the inferior vena cava, (4) closure of the ASD, (5) obliteration of the connection between the pulmonary artery and RV. Since its original description, the Fontan procedure has been modified many times. The first major change was elimination of the valves in the IVC and RA-PA connection. It also became apparent that the construction of a classic Glenn anastomosis prior to the Fontan operation was unnecessary and contributed to the development of pulmonary arteriovenous fistulae.
were the mainstay of surgical treatment for patients with tricuspid valve atresia. Unoperated patients with tricuspid atresia and cyanosis had a 1-year mortality of 90% (20). In 1971, Fontan and associates described a unique procedure to separate the systemic and pulmonary venous returns, eliminate the right-to-left intracardiac shunt and reduce ventricular volume overload (21,22,23). The components of the original Fontan operation consisted of the following: (1) classic Glenn anastomosis to direct superior vena cava flow to the right lung, (2) redirection of inferior vena cava flow to the pulmonary artery with a valved connection of the RA to the pulmonary artery, (3) insertion of a valve into the inferior vena cava, (4) closure of the ASD, (5) obliteration of the connection between the pulmonary artery and RV. Since its original description, the Fontan procedure has been modified many times. The first major change was elimination of the valves in the IVC and RA-PA connection. It also became apparent that the construction of a classic Glenn anastomosis prior to the Fontan operation was unnecessary and contributed to the development of pulmonary arteriovenous fistulae.
In the current era, the BDCPA became an integral part of the Fontan operation. Eventually, as clinicians noted the marked enlargement of the RA and high incidence of atrial arrhythmias associated with the atriopulmonary (AP) connection it was abandoned in favor of a lateral tunnel operation. This modification further evolved into the extracardiac conduit technique that is now the preferred approach by many for patients with tricuspid atresia.
In 1990, Bridges et al. (23) described the concept and use of a fenestration as part of the Fontan operation. It became clear that in high-risk patients, the use of a fenestration reduced postoperative morbidity and improved survival. Fontan fenestration reduced the duration of hospital stay and the duration and volume of chest tube drainage. Some investigators have suggested that the risk of stroke is increased for patients who had a fenestration, but other investigators have not confirmed this observation. Despite many technical modifications, the concept of directing systemic venous return directly to the pulmonary arteries without passing through a ventricle retained the eponym “modified Fontan procedure.” The Fontan operation remains as “definitive palliation” for most patients with single-ventricle physiology.
Echocardiographic Assessment of Fontan Physiology
The operative report is the most important tool for the echocardiographer when evaluating a patient after Fontan palliation. Reading the operative report is the mandatory initial step in the study because it defines the patient’s original anatomy and the connections between the systemic veins and the pulmonary arteries. It will outline previous surgical procedures that may require systematic evaluation. Finally, it will summarize the immediate postoperative hemodynamics giving the echocardiographer a clue to possible residual hemodynamic issues (Fig. 38.4).
Standard techniques should be applied to define the status of the aortic arch and ventricular and valvular performance in all patients with Fontan palliation. Venous and pulmonary arterial flow patterns are unique in the Fontan circulation and require additional consideration. It is important to use multiple imaging planes to assess the pulmonary and systemic venous flows. Convenient imaging planes include the parasternal and suprasternal sagittal planes to visualize the pulmonary artery confluence as it passes posterior to the aorta. The BDCPA and Fontan connection to the pulmonary artery can also be assessed from these windows. In addition, subcostal imaging is important to evaluate connection of the inferior vena cava and hepatic veins to the Fontan conduit.
Once the anatomic assessment of the Fontan connections has been completed and no abnormalities are identified, it then becomes important to evaluate flow through the Fontan circuit. The understanding of pulmonary artery flow dynamics has evolved over time. It is now considered that pulmonary flow occurs after Fontan operation for the following reasons:
Residual kinetic energy from the previous ventricular contraction
Negative intrathoracic pressure due to spontaneous breathing
Low pulmonary arterial resistance
Normal atrial and ventricular relaxation
Several of these factors can be evaluated with Doppler echocardiography. In 1991, Penny and Redington (24) demonstrated with simultaneous use of a respirometer, electrocardiogram, and Doppler echocardiography that forward flow in the pulmonary arteries was augmented by 35% during spontaneous inspiration compared to expiration. The conclusion of their study was that the “act of breathing” spontaneously provided additional energy to promote flow through the Fontan circuit.
![]() Figure 38.6 Pulsed-wave Doppler recording obtained from the right pulmonary artery of the patient with hypoplastic left heart syndrome and an extracardiac Fontan connection. This tracing also demonstrates phasic flows, but there are important differences to note relative to the flow patterns seen in Figure 41.7. Ventricular diastole and atrioventricular valve opening result in augmented forward flow in both types of Fontan connections. In this recording, this is reflected by the increased forward flow seen in early diastole (TVO). There is no atrial tissue in the extracardiac Fontan pathway, therefore the to-and-fro signal seen in Figure 41.7 is not evident. However, left atrial activity can still influence the pulmonary arterial flow pattern. When the “left” (pulmonary venous) atrium contracts (AC) pulmonary venous pressures rise slightly. This reduces forward flow velocity in the pulmonary artery somewhat. In an extracardiac Fontan, left atrial relaxation (AR) promotes forward flow by drawing blood out of the pulmonary veins and into the atrium. Although pulmonary arterial flow is still phasic in an extracardiac Fontan, the normal flow velocity should rarely decrease to near zero. This is unlike the flow seen in an atriopulmonary Fontan connection, where even flow reversals are common (Fig. 41.7). Intrathoracic pressure changes will produce the same alterations in these flow patterns that were described for the atriopulmonary Fontan connection in Figure 41.7. RPA, right pulmonary artery; TVO, tricuspid valve opening. (From Eidem BW, O’Leary PW, Cetta F. Echocardiography in Pediatric and Adult Congenital Heart Disease. 2nd ed. Philadelphia, PA: Wolters Kluwer; 2015.) |
Pulse wave Doppler evaluation permits evaluation of the variety of Fontan connections and the corresponding physiology of pulmonary arterial flow patterns. Figure 38.5 demonstrates the typical pulmonary artery flow of a direct AP connection (25). Figure 38.6 demonstrates typical pulmonary artery flow in a total caval-pulmonary artery connection. In the older AP connection, inspiration and ventricular relaxation augment flow when the systemic AV valve opens. Atrial contraction (systemic venous atrium) also augments flow. However, relaxation of the atrium will permit flow reversal. This to-and-fro flow pattern is not efficient and contributes to RA dilation.
In a tubular structure such as the extracardiac conduit, RA mechanics have less effect on flow. Flow reversals in the pulmonary arteries are not expected in an extracardiac conduit. Ventricular relaxation and opening of the systemic AV valve augment forward flow. One would not expect to see to-and-fro flow in an extracardiac conduit. Relaxation of the pulmonary venous (left) atrium draws blood flow from the pulmonary veins. In essence, there is suction through the lung bed by relaxation of the LA and the systemic ventricle.
Another important feature of the echocardiographic assessment of the patient after Fontan is flow through the fenestration. The mean pressure in the entire Fontan circuit should be equivalent: the mean SVC, IVC, Fontan conduit, and pulmonary artery pressures should all be equal. The mean pressure in the Fontan circuit should be greater than the mean pressure of the pulmonary venous atrium and flow through the fenestration is necessarily right to left. Flow through the fenestration can be assessed from several different imaging planes. Continuous wave Doppler assessment during several consecutive cardiac cycles generates a signal from which the mean gradient through the fenestration can be calculated (Fig. 38.7). The mean fenestration gradient is equivalent to the transpulmonary gradient. The normal gradient should be 5 to 8 mm Hg and is primarily determined by pulmonary vascular resistance. A low fenestration gradient is usually the result of hypovolemia. An elevated gradient should raise concern about Fontan pathway stenosis, intrinsic pulmonary resistance issues, lung disease, or pulmonary venous obstruction. However, elevation of the LA pressure or the ventricular end-diastolic pressure will not increase the transpulmonary (fenestration) gradient. Elevation of LA or end-diastolic pressure will cause a similar elevation in mean pulmonary artery and Fontan conduit pressures but will not change the transpulmonary gradient if cardiac output is normal.
Lastly, cardiac rhythm abnormalities may affect Fontan flow. Figure 38.8 demonstrates a patient after Fontan operation with AV dissociation. Large flow reversals that are “cannon A waves” are present in the pulmonary arteries and hepatic veins. These are reflections of the pulmonary venous atrium contracting against the closed systemic AV valve. The wave is transmitted back through the Fontan circuit. In patients with AV dissociation after Fontan operation there will be a reduction in forward flow with an occasional flow reversal.
Obstruction in the Fontan pathway and branch pulmonary arteries can be identified with two-dimensional and Doppler echocardiography. The importance of defining the size of the pulmonary artery/Fontan lumen cannot be overstated. Given the low pressure, nonpulsatile nature of “Fontan flow” very narrow stenoses may generate relatively small pressure differences. Therefore, a comparison of the minimum proximal vascular diameter (conduit/anastomosis or PA) to the maximum distal PA diameter is often the most useful measure of obstruction. If these cannot be clearly defined by echocardiography, and obstruction is clinically suspected, alternative imaging with CT, MRI, or angiography should be pursued. Doppler assessments need to be interpreted differently than in the normal heart. Evaluation of pulmonary artery flow with color Doppler requires reducing the Nyquist limit to 30 to 40 cm/s or less. Important stenoses may produce relatively low velocity flows (>0.8 m/s). In order to define a Doppler derived pressure gradient in these patients, one must account for respiratory variation. Tracing multiple sequential cardiac/respiratory
cycles in order to generate an “average” mean gradient is helpful when Fontan pathway stenosis is suspected (Fig. 38.9).
cycles in order to generate an “average” mean gradient is helpful when Fontan pathway stenosis is suspected (Fig. 38.9).
Diastolic dysfunction can also be identified after Fontan operation. Figure 38.10 demonstrates L waves in the systemic AV valve inflow signal and other evidence of elevated ventricular end-diastolic pressure in the pulmonary vein signals. Elevated systemic venous pressure in the Fontan circuit will be identified by reduced expiratory forward flow in the hepatic veins. Pulmonary venous obstruction is important to evaluate especially in the patient with an AP connection. Figure 38.11 shows a CT image with massive RA dilation causing compression of the right pulmonary veins. Complete imaging of the pulmonary circulation after Fontan operation requires more than echocardiography especially in the older child, adolescent, and adult. Incremental information from MRI directly impacts management.
Echocardiographic evaluation of the patient after Fontan is challenging. However, even when image quality is suboptimal, there are clues to Fontan failure that can be assessed:
Inferior vena cava dilation with presence of spontaneous echo contrast
Reduction in ventricular contractility and/or worsening diastolic function
Volumetric analysis, fractional area change, tissue Doppler, and myocardial strain data have been utilized in a quantitative manner
Assessment of anatomic pathway stenosis
Small gradients in the Fontan circuit and branch pulmonary arteries are important
Altered flow patterns can reflect diastolic dysfunction or dysrhythmia
Calculation of the transpulmonary gradient by measuring the “average” mean fenestration gradient over several cardiac cycles
Reduced gradients suggest hypovolemia or reduced cardiac output
TABLE 38.2 Choussat and Fontan Original Operative Criteria | ||||||||||
---|---|---|---|---|---|---|---|---|---|---|
|
Outcomes after Fontan Operation
Survival
Reports of outcomes after Fontan operation include all patients with functional single ventricle and may not be representative of only patients with tricuspid atresia. In general, results of the Fontan operation for patients with tricuspid atresia are superior to those for other forms of functional single ventricle.
Operative survival (<30 days) was 80% to 85% in early series and has increased to 95% in more recent studies (Fig. 38.12). For patients with tricuspid atresia operated between 1988 and 1997, the operative mortality was only 2%. Survival at 10 years ranges from 50% to 90% and at 15 years from 50% to 80%. Early and late mortality were higher for patients operated in the 1970s and 1980s than for patients operated more recently (24,25,26,27,28,29,30,31,32,33,34,35). Also, some of the risk factors for poor outcome, such as hypoplastic left heart syndrome (26) are now apparent.
Choussat and Fontan (21) recommended 10 criteria for a successful low-risk Fontan operation (Table 38.2). These criteria
imply that the operation was intended for patients with tricuspid atresia. These are relative criteria and it is clear that some are more important than others. For example many investigators have studied the effect of young age (<2 years or <4 years) upon survival. It is clear that age less than 4 years but greater than 2 years probably does not have a detrimental effect upon survival. However, age less than 2 years may have a negative effect. It has been demonstrated that patients lacking one or more of these criteria can survive operation, but the length of survival and quality of life are related to many of these criteria. Over the years, other investigators have identified additional important relative risk factors (Table 38.3). In 2015, Mayo Clinic reported a large follow-up study of 1,052 patients after Fontan operation (273 patients with tricuspid atresia). The 10-, 20-, and 30-year survival was 79%, 62%, and 45% respectively for the patients with tricuspid atresia.
imply that the operation was intended for patients with tricuspid atresia. These are relative criteria and it is clear that some are more important than others. For example many investigators have studied the effect of young age (<2 years or <4 years) upon survival. It is clear that age less than 4 years but greater than 2 years probably does not have a detrimental effect upon survival. However, age less than 2 years may have a negative effect. It has been demonstrated that patients lacking one or more of these criteria can survive operation, but the length of survival and quality of life are related to many of these criteria. Over the years, other investigators have identified additional important relative risk factors (Table 38.3). In 2015, Mayo Clinic reported a large follow-up study of 1,052 patients after Fontan operation (273 patients with tricuspid atresia). The 10-, 20-, and 30-year survival was 79%, 62%, and 45% respectively for the patients with tricuspid atresia.
TABLE 38.3 Additional Risk Factors for Fontan Operation | ||||||||||||||||||
---|---|---|---|---|---|---|---|---|---|---|---|---|---|---|---|---|---|---|
|
Problems after Fontan Operation
Arrhythmia
Gelatt et al. (36) reported the incidence of atrial arrhythmia 5 years after Fontan operation for patients who had an AP connection, a total caval pulmonary connection, or RA to RV connection as 33%, 18%, and 11% respectively. Durongpisitkul et al. (37) reported incidence of atrial arrhythmia of 6%, 17%, and 30% at 1, 5, and 10 years after operation. The occurrence of arrhythmias may be lower after the extracardiac Fontan (Fig. 38.13).
Protein Losing Enteropathy
Protein losing enteropathy (PLE) is a lethal complication of the Fontan operation. It was first described in 1980 by Crupi et al. (38) in a patient with single-ventricle physiology after a Fontan operation.
PLE is characterized by fatigue, peripheral edema, ascites, pleural effusions, growth delay, and/or diarrhea. These patients may exhibit low serum albumin, low serum protein, hypocalcemia, acquired hypogammaglobulinemia, and elevated fecal α-1-antitrypsin (38,39,40,41,42,43). In some cases, onset of symptoms may be related to a recent nonspecific illness. PLE usually begins 3.5 years following Fontan operation (42). However, it can occur immediately after operation and has been reported 16 years postoperatively. Data from Mayo Clinic (43) indicate that PLE occurs in up to 10% of cases and 5-year survival after the onset of PLE was 50%. That study included patients from the early surgical experience and none of those patients had a fenestration. In the current era John et al. (44), in a noncohort study, reported less mortality (22%) at 5 years after onset of PLE. In 2015 Pundi et al. (45) reported an 8% incidence of PLE in a cohort study of 1,052 patients from Mayo Clinic. In that study, 5-year survival after diagnosis of PLE was only 45%.
PLE is characterized by fatigue, peripheral edema, ascites, pleural effusions, growth delay, and/or diarrhea. These patients may exhibit low serum albumin, low serum protein, hypocalcemia, acquired hypogammaglobulinemia, and elevated fecal α-1-antitrypsin (38,39,40,41,42,43). In some cases, onset of symptoms may be related to a recent nonspecific illness. PLE usually begins 3.5 years following Fontan operation (42). However, it can occur immediately after operation and has been reported 16 years postoperatively. Data from Mayo Clinic (43) indicate that PLE occurs in up to 10% of cases and 5-year survival after the onset of PLE was 50%. That study included patients from the early surgical experience and none of those patients had a fenestration. In the current era John et al. (44), in a noncohort study, reported less mortality (22%) at 5 years after onset of PLE. In 2015 Pundi et al. (45) reported an 8% incidence of PLE in a cohort study of 1,052 patients from Mayo Clinic. In that study, 5-year survival after diagnosis of PLE was only 45%.
Thromboembolism
Monagle, et al. (46) summarized a number of studies and reported that the occurrence of thrombosis ranged from 2.2% to 19% after Fontan operation. Routine anticoagulation regimes after Fontan operation have been institution specific. Some centers utilize warfarin therapy only in patients after fenestration, others utilize antiplatelet therapy (usually daily aspirin). Warfarin therapy has not proved superior to aspirin therapy in thromboembolism prevention in children after Fontan operation (47). The 2012 American Chest Physician evidence-based guidelines recommended aspirin therapy or unfractionated heparin therapy followed by vitamin K antagonists over no therapy after Fontan operation (Grade 1C: strongly recommended but low/very low quality evidence) (48). Anticoagulation regimes may change as experience with novel oral anticoagulants increases and as patients enter their second and third decades. Many adults after Fontan receive warfarin therapy due to recurrent atrial arrhythmia, poor ventricular function, residual shunt, and/or thromboembolic events. Anticoagulation after Fontan needs to be tailored to the individual patient and the clinical situation (49).
Exercise Performance
Patients with single-ventricle physiology have reduced aerobic capacity (VO2max = 20.5 to 23.1 mL/kg/min). The major factor contributing to reduced aerobic capacity for these patients is the presence of a right-to-left shunt. Because of the right-to-left shunt, overventilation occurs in order to maintain appropriate arterial pCO2 and pH levels. Overventilation is manifest by an elevated ventilatory equivalent for oxygen (VE/VO2) at rest and a markedly increased VE/VO2 at peak exercise. Indeed the need to increase VE is such that these patients would, theoretically, have to exceed their resting maximum voluntary ventilation (MVV) in order to achieve predicted normal VO2max; this is not possible.
There have been numerous studies of aerobic capacity after fenestrated and nonfenestrated Fontan operation. The values for VO2max range from 15.9 to 28.5 mL/kg/min (50,51,52). Studies in which postoperative values were compared to preoperative values have shown that there is a significant increase in postoperative VO2max (50,51,52,53,54). However, postoperative VO2max remains significantly below normal subjects. Durongpisitkul et al. (51) demonstrated that the determinants of VO2max included age at exercise, preoperative confluent pulmonary arteries, and resting VO2max (50). Ohuchi et al. (53) reported that LV morphology was associated with higher VO2max than for patients with a morphologic RV. Fredriksen et al. (54) found that higher VO2max was associated with earlier age of operation and male gender. These investigators also reported the presence of restrictive chest wall mechanics after Fontan operation. Most of the studies of aerobic capacity after Fontan operation have been performed for patients without a fenestration. Patients with a fenestration, because of the right-to-left shunt, likely, will have lower VO2max. Numerous investigators have shown that cardiac output and stroke volume are subnormal after Fontan operation. Sports participation for patients after Fontan operation is addressed in another chapter in this textbook and usually is recommended to be low-intensity and self-limited by the patient.
Effects of Fontan Physiology on the Liver
Unfortunately, one of the long-term consequences of Fontan physiology is the detrimental effect on the liver. There have been case reports of hepatocellular carcinoma after Fontan operation (53). Liver biopsy has been suggested for all patients 10 years after Fontan operation (56). Others advocate a less invasive approach using MR elastography (MRE) to determine liver stiffness, differentiate fibrosis from cirrhosis, and select patients and sites for biopsy. MRE quantitatively assesses the mechanical properties of liver tissue (57). The “stiffness index” has been developed in patients with hepatitis and other liver disease its application to the patient after Fontan operation is promising (Fig. 38.14).
Ebstein Anomaly
Introduction
In 1866, Wilhelm Ebstein described an autopsy that revealed a strikingly abnormal tricuspid valve (58) (Fig. 38.15). The patient
was a 19-year-old laborer who had presented with dyspnea a few days earlier. The anterior leaflet of the tricuspid valve was described as normal and arose from the tricuspid annulus. However, the septal and inferior leaflets were redundant and arose from RV myocardium below the annulus. Subsequently, others described similar findings (59). Yet, it was not until 1950 that “Ebstein anomaly,” also referred to as “Ebstein malformation,” was clinically recognized (60).
was a 19-year-old laborer who had presented with dyspnea a few days earlier. The anterior leaflet of the tricuspid valve was described as normal and arose from the tricuspid annulus. However, the septal and inferior leaflets were redundant and arose from RV myocardium below the annulus. Subsequently, others described similar findings (59). Yet, it was not until 1950 that “Ebstein anomaly,” also referred to as “Ebstein malformation,” was clinically recognized (60).
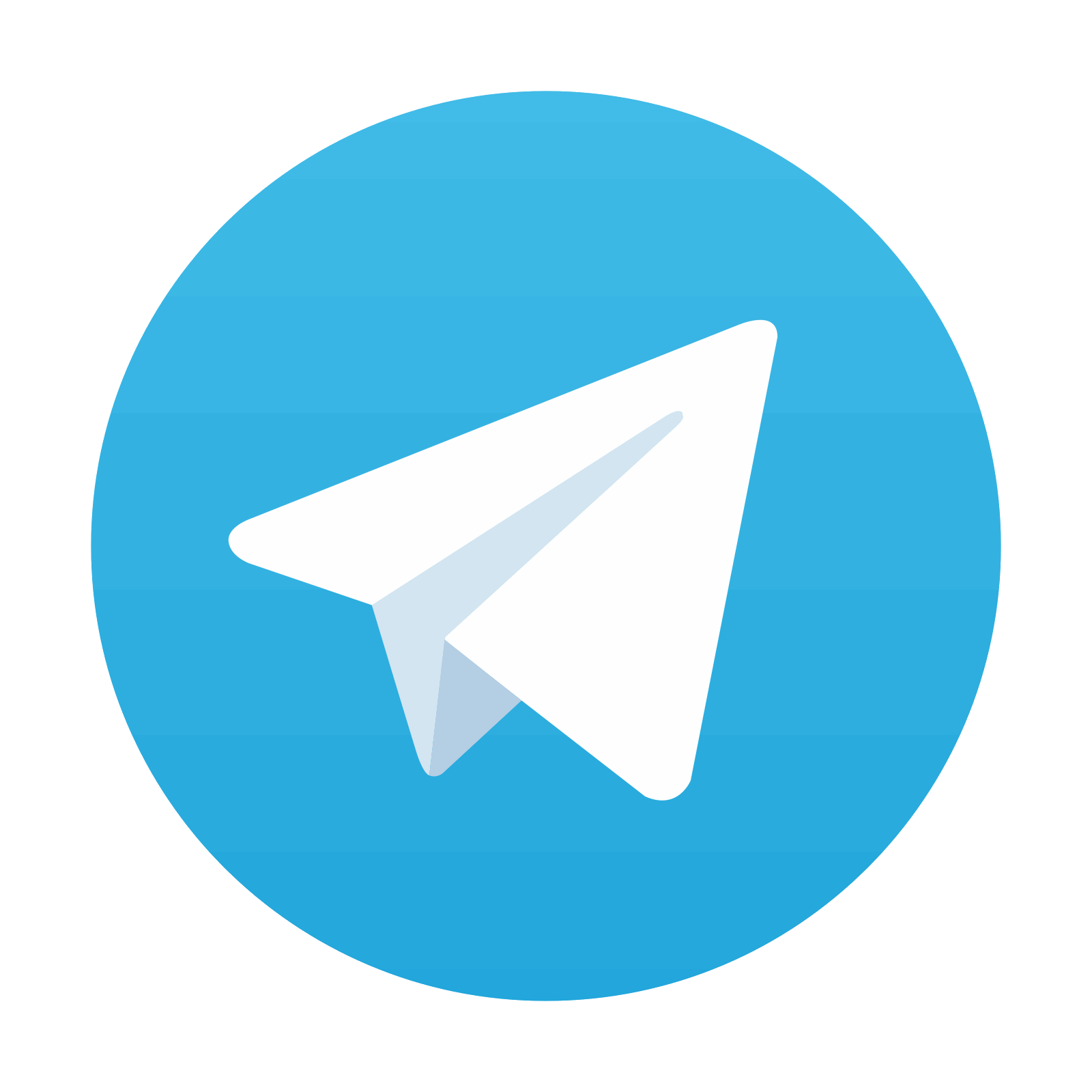
Stay updated, free articles. Join our Telegram channel

Full access? Get Clinical Tree
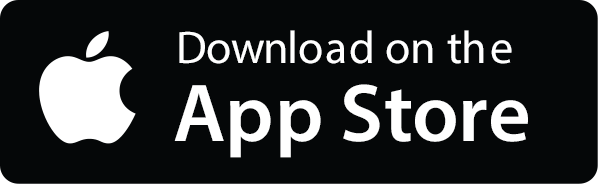
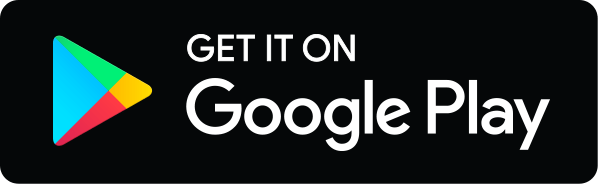