Transposition of the Great Arteries
Athar M. Qureshi
Henri Justino
Jeffrey S. Heinle
Introduction
First described by the Scottish pathologist Dr. Matthew Baillie in 1797 (1), transposition of the great arteries was for decades merely known as a fascinating disease that was universally fatal. Many innovations in therapy along the way, including the introduction of the balloon atrial septostomy by Rashkind and Miller (2) and Jatene’s report of the arterial switch operation (3) revolutionized the way that we now care for these infants. Patients who were initially felt to have a fatal disease can now be expected not only to survive, but also to have an excellent quality of life. Indeed, the innovations in therapy over the years for patients with transposition of the great arteries became the basis for many advances in technology in the disciplines of interventional cardiology and cardiac surgery.
In this chapter, we will discuss lesions with concordant atrioventricular connections and discordant ventriculoarterial connections, or “simple transposition.” This form of transposition is also known as D-TGA (dextro transposition of the great arteries) or D-looped transposition. These lesions encompass transposition of the great arteries with intact ventricular septum, transposition of the great arteries with ventricular septal defect, and transposition of the great arteries with ventricular septal defect and left ventricular outflow tract obstruction (Fig. 47.1). Other forms of transposition of the great arteries, for example, congenitally corrected transposition of the great arteries or transposition of the great arteries in association with other lesions, such as double outlet right ventricle, are discussed elsewhere in this text.
Epidemiology (Incidence, Prevalence)
Transposition of the great arteries is the most common form of cyanotic congenital heart disease presenting in the newborn period and comprises approximately 5% of all congenital heart defects (4). Early estimates from the Report of the New England Regional Infant Program (5) reported the incidence of transposition of the great arteries to be 218 per million live births. A more recent meta-analysis from 41 studies estimated the incidence to be a median of 303 and mean of 315 per million live births (6). Investigators from the National Birth Defects Prevention Network (NBDPN) found the prevalence of transposition of the great arteries to be 4.73 per 10,000 live births during 1999–2001 (7). These numbers were revised in an updated report (8) to 3 per 10,000 live births during the time period of 2004–2006, largely due to more accurate coding for inclusion criteria.
Etiology
Genetic
Transposition of the great arteries is predominately seen in males (particularly large-term males). As opposed to other conotruncal abnormalities, transposition of the great arteries is rarely associated with chromosomal abnormalities (9), although infrequent reports of associations with chromosomal genetic syndromes exist (10). Moreover, unlike other conotruncal abnormalities, extracardiac associations are less frequent (10%) than with other conotruncal outflow tract abnormalities (33%) (11). It is however, associated with dextrocardia (10) and lateralization defects, for example, heterotaxy syndrome, particularly asplenia or right isomerism (10,12). Transposition of the great arteries has been associated with laterality genes associated with heterotaxy syndrome (10,12,13,14,15) and evidence of isolated associations with mutations of the laterality genes CFC1 (13), Nodal (14), and ZIC3 (15) have been identified.
Familial recurrence was previously not felt to occur in patients with transposition of the great arteries. However, in a multicenter study from Italy, the recurrence rate was found to be 1.7% in siblings of patients with transposition of the great arteries (16).
Noninherited and Environmental Risk Factors
Diabetes and Vitamin A have been the most studied modifiable maternal risk factors contributing to transposition of the great arteries. Pregestational diabetes is positively associated with transposition of the great arteries (17,18,19,20). Hyperglycemia has been found to be a major teratogen with regard to transposition of the great arteries (21), and some of these effects can be reversed with treatment with Vitamin E (21,22). High maternal intake of supplemental Vitamin A may significantly increase the risk of having offspring with transposition of the great arteries (23). Maternal exposure to ibuprofen (20), influenza (20), organic solvents (20), and pesticides (24) have all been reported to increase the risk specifically for transposition of the great arteries, in addition to other congenial heart defects.
Morphogenesis
The embryologic basis of transposition of the great arteries is less well understood than other conotruncal abnormalities. Unlike other conotruncal abnormalities, neural crest ablation does not result in transposition of the great arteries (25). In embryonic development, normally, the aorticopulmonary septum develops a spiral-like configuration resulting in the pulmonary trunk twisting around the ascending aorta. There are two main proposed theories for the embryologic basis for transposition of the great arteries. One theory, the “straight conal septal” theory (26) proposes that there is lack of spiral rotation of the aorticopulmonary septum. The other theory, first proposed by Goor and Edwards (27) and substantiated by Anderson et al. (28) and Van Praagh and Van Praagh (29), is the “differential conal development” theory, which we favor. Normally in embryonic development, the subpulmonary conus grows while the subaortic conus undergoes resorption. In embryos that develop transposition of the great arteries, the subaortic conus enlarges, while the subpulmonary conus is resorbed (27,28,29). The aorta is thus positioned anterior and rightward with the pulmonary artery being posterior and leftward.
High-dose retinoic acid, by inducing hypoplasia of the conus swellings, may be a primary event with resultant lack of counterclockwise rotation of the distal outflow tract, that leads to transposition of the great arteries (30,31,32). Perlecan-deficient embryos have been shown to exhibit transposition of the great arteries and it is hypothesized that this is due to hyperplastic conotruncal endocardial cushions from excess mesenchymal cells causing disorganized spiral endocardial ridges (33).
Morphology
Pathologic Anatomy, Variants and Conduction Anatomy
The morphology in transposition of the great arteries can be most aptly described as an abnormality of ventriculoarterial connection. Almost invariably, patients with transposition of the great arteries have situs solitus, levocardia, concordant atrioventricular connections, and right-hand ventricular topology (D-TGA or D-looped). The fundamental abnormality involves the presence of a discordant ventriculoarterial connection. As such, the arterial trunks arise from the morphologically inappropriate ventricle, that is, the right ventricle is predominantly aligned with the aorta, while the left ventricle is predominantly aligned with the pulmonary artery. While this arrangement most typically results in the aorta being anterior and rightward, while the pulmonary artery is posterior and leftward, it is important to note that it is not the arrangement of the great arteries in space that defines transposition of the great arteries, but rather the connection of the great arteries to their respective ventricles. Historically, the term transposition had been loosely applied to a wide variety of congenital anomalies in which the great artery arrangement in space was abnormal regardless of connection to the ventricular mass (34). In addition, rare forms of transposition of the great arteries can have a great artery arrangement that resembles the normal arrangement in space (i.e., an aorta that is posterior and rightward with a pulmonary artery that is anterior and leftward), albeit with the arterial trunks arising from the morphologically inappropriate ventricles. It is also important to note that the term transposition of the great arteries should not be applied to patients with additional abnormalities of atrioventricular connection (e.g., patients with discordant atrioventricular connection, patients with double inlet connection, etc.). It is worthy of mention that the same fundamental abnormality of discordant ventriculoarterial connection can be encountered in the mirror image arrangement of normal, that is, with situs inversus.
The conduction system in “simple” transposition of the great arteries discussed in this chapter is generally normal. In transposition of the great arteries with a ventricular septal defect with fibrous continuity between the tricuspid and pulmonary valves leaflets, the conduction system is posterior and inferior to the defect (35). The conduction system is posterior and lateral to the tricuspid valve orifice if straddling or overriding of the tricuspid valve is present (35).
Associated Lesions
The most common associated lesions in transposition of the great arteries are the presence of a ventricular septal defect, with or without additional presence of left ventricular outflow tract obstruction. In the newborn, the presence of a foramen ovale and ductus arteriosus is expected, and as such these two are not considered associated lesions.
The morphology of ventricular septal defects in transposition of the great arteries is variable, and essentially all types of ventricular septal defects found in patients with otherwise normal connections can also be found in patients with transposition of the great arteries (35). A common type of ventricular septal defect encountered in transposition of the great arteries includes direct fibrous continuity between the tricuspid and pulmonary valve, that is, a perimembranous ventricular septal defect. In the setting of transposition of the great arteries, this type of ventricular septal defect is often also referred to as conoventricular. Another common type of ventricular septal defect encountered in transposition of the great arteries is the so-called malalignment type of ventricular septal defect, characterized by the malalignment between the outlet septum and the remainder of the muscular ventricular septum. Malalignment of the outlet septum can occur posteriorly toward the pulmonary outflow tract, and in this manner is responsible for one of the common mechanisms of left ventricular outflow tract obstruction. However, anterior deviation of the outlet septum can also occur, resulting in some degree of override of the pulmonary valve over the right ventricle. In its extreme form, anterior deviation of the outlet septum results in the double outlet right ventricle with a subpulmonary ventricular septal defect, the so-called Taussig–Bing anomaly. A less common type of ventricular septal defect in transposition of the great arteries is the muscular defect, which can be located in the inlet, outlet, apical, or mid-muscular septum, and can sometimes be multiple. Less common types still include those so-called “canal type” of inlet ventricular septal defects included within the spectrum of atrioventricular septal defects with the associated abnormalities of the atrioventricular junction and atrioventricular valves, and sometimes associated with straddling of the tricuspid valve in transposition of the great arteries. The least common type is the so-called doubly committed subarterial ventricular septal defect, in which both semilunar valves are in direct fibrous continuity with each other, and characterized by the absence of muscular outlet septum in this area. Of the types of ventricular septal defects encountered in transposition of the great arteries, only muscular ventricular septal defects and perimembranous ventricular septal defects have the potential for becoming smaller over time or even spontaneously closing.
The mechanisms responsible for left ventricular outflow tract obstruction in transposition of the great arteries are numerous, and include obstruction at the level of the pulmonary valve itself, as well as several causes of obstruction at the subpulmonary region, which include posterior malalignment of the outlet septum, tissue tags, muscular ridges, and anomalous insertion of the mitral valve apparatus (35). Precise characterization of the mechanism responsible for subpulmonary obstruction is paramount, because the ability to resect the cause of obstruction is central to the ability to perform an arterial switch operation.
The independent occurrence of ventricular septal defect and left ventricular outflow tract obstruction results in four possible combinations of lesions in transposition of the great arteries: transposition of the great arteries with intact ventricular septum, transposition of the great arteries with ventricular septal defect, transposition of the great arteries with ventricular septal defect and left ventricular outflow tract obstruction, and transposition of the great arteries with intact ventricular septum and left ventricular outflow tract obstruction (rarest form). The frequency of these variants occurs in that order, with transposition of the great arteries with intact ventricular septum by far the most common, comprising more than 50% of these combinations (36).
Additional lesions that may be encountered in transposition of the great arteries include coarctation of the aorta or interruption of the aortic arch, most often encountered in anterior deviation of the outlet septum (Taussig–Bing anomaly). Atrioventricular septal defects are uncommon. One rare associated abnormality that deserves special mention is juxtaposition of the atrial appendages, which in transposition of the great arteries most often consists of left juxtaposition of the right atrial appendage (37). Although this anomaly, in and of itself, is inconsequential to the physiology of transposition of the great arteries and does not impact directly on the surgical repair options (although it may affect a Senning operation), it is important that this anomaly be recognized using echocardiography (see “Echocardiography” section) at the time of diagnosis of transposition of the great arteries and may have implications when performing a balloon atrial septostomy (see “Balloon Atrial Septostomy” section).
Lastly, coronary anomalies are so common in transposition of the great arteries that they may not typically be considered to constitute associated lesions as such. The highly variable origins of the coronary arteries as well as their variable epicardial course is the subject of much study. Various classifications of coronary branching patterns have been described, of which the Leiden convention (38) and Yacoub and Radley-Smith classification (Fig. 47.2) (39) are
the most common. The “type A” or normal coronary distribution is the most common branching pattern, but abnormal coronary branching patterns can be seen in up to one-third of patients (40).
the most common. The “type A” or normal coronary distribution is the most common branching pattern, but abnormal coronary branching patterns can be seen in up to one-third of patients (40).
Physiology of Transposition of the Great Arteries
The physiology in transposition of the great arteries is significantly more complex than that of simple left-to-right or right-to-left shunts, and can vary widely according to associated lesions and according to the state of the patient’s pulmonary vascular resistance during the normal neonatal evolution from fetal, to transitional, to adult-type circulation.
The essence of the circulatory derangement in transposition of the great arteries is that the systemic and pulmonary circulations are arranged in parallel rather than in series. The left ventricle, which receives fully oxygenated blood from the pulmonary veins, pumps this oxygenated blood through the pulmonary valve into the lungs, where it cannot possibly pick up any more oxygen. Similarly, the right ventricle, which receives deoxygenated blood from the systemic veins, pumps this deoxygenated blood across the aortic valve back to the body, where it cannot deliver much oxygen to the body. After being pumped to the body, the even more deoxygenated blood returns to the right ventricle, and is once again pumped to the body. According to this construct, one can see that within just a few heartbeats, the right ventricle would be pumping blood that is essentially devoid of oxygen, while the left ventricle keeps recirculating fully oxygenated blood to, and from, the lungs. Clearly survival with the systemic and pulmonary circulations arranged in parallel is not possible, and requires some blood to exit the pulmonary circuit in order to enter the systemic circuit, and for blood to similarly exit the systemic circuit in order to enter the pulmonary circuit. Any blood exiting one circuit to enter the other would result in one circulation emptying itself into the other; there must therefore be an equal flow returning blood back to the original circuit. The levels of communication that allow blood to cross from one circuit to the other can occur either centrally at the atrial (atrial septal defect or patent foramen ovale), ventricular (ventricular septal defect), or great artery level (patent ductus arteriosus), or can occur peripherally at the naturally existing shunts at the level of the bronchial circulation and thebesian veins.
In order to correctly describe the intricacies of this highly variable physiology, it is important to recall some basic definitions, which will first be illustrated using simpler forms of congenital heart disease, and then be applied to the understanding of transposition of the great arteries. First, pulmonary blood flow (QP) refers to the entire flow through the pulmonary vascular bed, while systemic blood flow (QS) refers to the entire flow through the systemic vascular bed. Effective pulmonary blood flow (QEP) refers to the flow of systemic venous blood into the pulmonary circulation, that is, the deoxygenated blood that is being sent to the lungs to undergo oxygenation. Similarly, effective systemic blood flow (QES) refers to the flow of pulmonary venous blood into the systemic circulation, that is, the oxygenated blood that is being sent to the body to deliver oxygen. The amount of oxygen absorbed by the blood circulating in the lungs must equal the amount of oxygen delivered by the blood circulating to the body, hence QEP must always equal QES. In a completely normal heart, QP = QEP, QS = QES, and QP = QS. A left-to-right shunt is defined as the flow of blood which has already completed its circulation to the lungs, and yet is being sent to the lungs once again without having seen the systemic circulation, while a right-to-left shunt represents the flow of blood that has already completed its course through the systemic circulation, and yet is being circulated once more to the systemic circulation without seeing the pulmonary circulation. In hearts with simple left-to-right shunts (e.g., atrial or ventricular septal defects with pure left-to-right shunting), the flow being shunted into the pulmonary circulation across the defect (QL→R) is additive to the flow coming in normally from the systemic veins to enter the lungs (QEP), hence QP = QEP + QL→R. Similarly, in hearts with simple right-to-left shunts (e.g., an atrial septal defect shunting purely right-to-left), the flow shunted right to left across the atrial septal defect (QR→L) is being added to the normal blood flow from the pulmonary veins being pumped out to the aorta (QES), hence the total amount being sent to the body is the sum of these two blood flows, that is, QS = QES + QR→L.
In transposition of the great arteries specifically, the terms “left-to-right shunt” or “right-to-left shunt” can be confusing, and some authors have attempted to avoid confusion by distinguishing the terms “physiologic left-to-right shunt” from “anatomic left-to-right shunt.” We prefer to use the term “left-to-right shunt” to mean what it intends in any other cardiac lesion, that is, the “physiologic left-to-right shunt.” Rather than utilizing the term “anatomic shunt” we prefer to describe the direction of flow from one chamber or vessel to the other chamber or vessel. This will become clear as we discuss what precisely constitutes the “shunted” blood in transposition of the great arteries. First, it is important to recognize that the deoxygenated blood pumped by the right ventricle to the aorta in transposition of the great arteries constitutes, in fact, a large right-to-left shunt (QR→L) because it fulfills the definition described above (systemic venous blood being pumped back to the body without having seen the pulmonary circulation). Similarly, the oxygenated blood pumped by the left ventricle to the pulmonary artery is, in fact, the left-to-right shunt (QL→R) because it fulfills the definition of a left-to-right shunt (pulmonary venous blood being pumped back to the lungs without having circulated through the body). It is therefore important to note that the small amount of blood typically crossing the foramen ovale from the left atrium to the right atrium in a newborn with TGA is not a left-to-right shunt, but is rather the only portion of the oxygenated blood that is flowing “correctly” to the body (hence, it is actually the QES). It is therefore inaccurate in the setting of transposition of the great arteries to describe flow occurring from the left atrium to the right atrium across a foramen ovale as a left-to-right shunt, although some authors might use the term “anatomic left-to-right shunt” because it is occurring from a left-sided anatomical structure to a right-sided anatomical structure (certainly, all would agree that this does not constitute a physiologic left-to-right shunt). Similarly, any blood flowing from the aorta to the pulmonary artery across the ductus arteriosus actually represents deoxygenated blood being sent to the lungs to pick up oxygen, hence it constitutes the QEP, and it most certainly is not a physiologic shunt. In addition, it can be seen that the terminology “anatomic shunt” breaks down when describing a shunt between the aorta and the pulmonary artery: Normally the aorta is considered a left-heart structure and the pulmonary artery a right-heart structure, but in transposition of the great arteries, the aorta is connected to the right ventricle and the remainder of the right heart. Does that make the aorta an anatomic right-heart structure, while the pulmonary artery becomes a left-heart structure? This becomes confusing, and it is therefore best to avoid the terms “anatomic left-to-right shunt” and “anatomic right-to-left shunt,” in favor of describing the actual chambers or vessels that allow communication between them and the manner in which the flow occurs between these structures (e.g., “flow from the aorta to the pulmonary artery,” “flow from the pulmonary artery to the aorta,” “flow from the left atrium to right atrium,” etc.). Similarly when interpreting hemodynamic cardiac catheterization data in these patients, it is important to understand the limitations in this situation and to interpret the data in the context of “transposition physiology” discussed in this section.
When considering a newborn baby with transposition of the great arteries, the foramen ovale is expected to be available for shunting, as is the ductus arteriosus in the first few minutes or hours of life. Hence, the two parallel circuits consisting of the pulmonary and systemic circulations are expected to have two possible areas of communication between them in almost every newborn with transposition of the great arteries. Superimposed
on this congenital anomaly is the near-normal evolution of pulmonary vascular resistance in the newborn period. Hence, immediately after birth the pulmonary vascular resistance is elevated, and accordingly it is possible for the ductus arteriosus to have flow occur bidirectionally, typically from pulmonary artery to aorta in systole, and from aorta to pulmonary artery in diastole. This phenomenon is responsible for the classic “reversed differential cyanosis” seen in newborns with transposition of the great arteries (i.e., the lower body will be relatively pink while the upper body will be relatively cyanotic). It is important to note that this phenomenon is a fleeting one, typically lasting only the first few hours of life. The two conditions necessary for the presence of this phenomenon are patency of the ductus arteriosus, and elevation of the pulmonary vascular resistance. The systolic flow of blood from pulmonary artery to aorta brings highly oxygenated blood from the pulmonary artery to the descending aorta to result in relatively higher saturations in the lower extremities compared to the upper extremities. However, within the first few hours of life as the pulmonary vascular resistance drops precipitously, there will cease to be systolic flow from pulmonary artery to aorta across the ductus, and eventually the flow across the ductus will entirely be from aorta to pulmonary artery throughout the cardiac cycle. When this occurs, differential cyanosis disappears, and with it an important bedside clinical clue to the presence of transposition physiology. The increased flow across the ductus arteriosus from aorta to pulmonary artery caused by the drop in pulmonary vascular resistance also results in increased pulmonary venous return to the left atrium. The relative increase in left atrial pressure causes the foramen ovale to flow from the left atrium to right atrium. During this phase of the physiology, any net amount of blood flow occurring from aorta to pulmonary artery across the ductus (QEP) is matched by an equal flow occurring from left atrium to right atrium (QES), and these two flows are keeping the newborn alive, as these are the only flows participating in actual oxygen exchange. It is therefore evident that restriction at the level of the foramen ovale and/or ductus arteriosus limits the ability of deoxygenated blood to enter the lungs (QEP) and oxygenated blood to enter the body (QES). This physiologic set up is the justification of two of the most important strategies for treatment of newborns with transposition of the great arteries: the institution of a prostaglandin infusion to maintain patency of the ductus, and the performance of a balloon atrial septostomy to enlarge the foramen ovale. It is a common misconception that the ductus and foramen are necessary to improve “mixing” in transposition of the great arteries. In fact, it is not mixing back-and-forth across these structures, but rather net flow across these structures (from aortic to pulmonary artery, and from left atrium to right atrium) that is occurring during this physiologic state in transposition of the great arteries (41). After a septostomy has been performed, the systemic arterial saturation typically increases tremendously, such that prostaglandin can frequently be discontinued. In the setting of a closed ductus, the patient then relies essentially on the atrial defect that has been created via balloon atrial septostomy to maintain adequate oxygen saturation. In this physiologic state, the flow of blood across the atrial septal defect occurs bidirectionally, thus it is perfectly accurate to describe the atrial septal defect as being responsible for improving “mixing.” Whether prostaglandin can be discontinued after a balloon atrial septostomy is very much institution dependent, with some institutions preferring to discontinue prostaglandin infusions due to their softening effect on the cardiac tissues at the time of the operation, while other institutions prefer that their patients have higher oxygen saturations preoperatively, to which ductal patency certainly contributes.
on this congenital anomaly is the near-normal evolution of pulmonary vascular resistance in the newborn period. Hence, immediately after birth the pulmonary vascular resistance is elevated, and accordingly it is possible for the ductus arteriosus to have flow occur bidirectionally, typically from pulmonary artery to aorta in systole, and from aorta to pulmonary artery in diastole. This phenomenon is responsible for the classic “reversed differential cyanosis” seen in newborns with transposition of the great arteries (i.e., the lower body will be relatively pink while the upper body will be relatively cyanotic). It is important to note that this phenomenon is a fleeting one, typically lasting only the first few hours of life. The two conditions necessary for the presence of this phenomenon are patency of the ductus arteriosus, and elevation of the pulmonary vascular resistance. The systolic flow of blood from pulmonary artery to aorta brings highly oxygenated blood from the pulmonary artery to the descending aorta to result in relatively higher saturations in the lower extremities compared to the upper extremities. However, within the first few hours of life as the pulmonary vascular resistance drops precipitously, there will cease to be systolic flow from pulmonary artery to aorta across the ductus, and eventually the flow across the ductus will entirely be from aorta to pulmonary artery throughout the cardiac cycle. When this occurs, differential cyanosis disappears, and with it an important bedside clinical clue to the presence of transposition physiology. The increased flow across the ductus arteriosus from aorta to pulmonary artery caused by the drop in pulmonary vascular resistance also results in increased pulmonary venous return to the left atrium. The relative increase in left atrial pressure causes the foramen ovale to flow from the left atrium to right atrium. During this phase of the physiology, any net amount of blood flow occurring from aorta to pulmonary artery across the ductus (QEP) is matched by an equal flow occurring from left atrium to right atrium (QES), and these two flows are keeping the newborn alive, as these are the only flows participating in actual oxygen exchange. It is therefore evident that restriction at the level of the foramen ovale and/or ductus arteriosus limits the ability of deoxygenated blood to enter the lungs (QEP) and oxygenated blood to enter the body (QES). This physiologic set up is the justification of two of the most important strategies for treatment of newborns with transposition of the great arteries: the institution of a prostaglandin infusion to maintain patency of the ductus, and the performance of a balloon atrial septostomy to enlarge the foramen ovale. It is a common misconception that the ductus and foramen are necessary to improve “mixing” in transposition of the great arteries. In fact, it is not mixing back-and-forth across these structures, but rather net flow across these structures (from aortic to pulmonary artery, and from left atrium to right atrium) that is occurring during this physiologic state in transposition of the great arteries (41). After a septostomy has been performed, the systemic arterial saturation typically increases tremendously, such that prostaglandin can frequently be discontinued. In the setting of a closed ductus, the patient then relies essentially on the atrial defect that has been created via balloon atrial septostomy to maintain adequate oxygen saturation. In this physiologic state, the flow of blood across the atrial septal defect occurs bidirectionally, thus it is perfectly accurate to describe the atrial septal defect as being responsible for improving “mixing.” Whether prostaglandin can be discontinued after a balloon atrial septostomy is very much institution dependent, with some institutions preferring to discontinue prostaglandin infusions due to their softening effect on the cardiac tissues at the time of the operation, while other institutions prefer that their patients have higher oxygen saturations preoperatively, to which ductal patency certainly contributes.
Associated lesions such as ventricular septal defects, pulmonary stenosis, or both, add additional complexity to the physiology of transposition of the great arteries. When present, a ventricular septal defect predominantly will shunt from right ventricle to left ventricle, thus allowing deoxygenated blood from the right ventricle to enter the pulmonary arteries, contributing to QEP. Accordingly, the saturations will generally be higher in patients with transposition of the great arteries with ventricular septal defect. In patients with transposition of the great arteries, ventricular septal defect, and pulmonary stenosis, the pulmonary stenosis, especially if severe, will significantly limit pulmonary blood flow and contribute to lower oxygen saturation.
Clinical Features and Investigation
Presentation and Symptoms
The clinical features of children with transposition of the great arteries depends on the anatomic details of the lesion (e.g., presence of an intact ventricular septum, a ventricular septal defect, or left ventricular outflow tract obstruction). In addition, the clinical features depend on the age of presentation, which in most cases is the newborn period, but in developing countries, late presentation can occur.
Newborns with transposition of the great arteries and intact ventricular septum present with cyanosis. Inadequate atrial and ductal communications result in profound cyanosis and lethargy. In the presence of a large VSD, “pulmonary overcirculation” or heart failure may ensue as the pulmonary vascular resistance drops, and is manifested by tachypnea. Patients with left ventricular outflow tract obstruction and a ventricular septal defect may present with profound cyanosis and lethargy if severe left ventricular outflow tract obstruction is present, or heart failure symptoms if little obstruction is present. Mild cyanosis with no symptoms may be present in infants with a “well-balanced” degree of left ventricular outflow tract obstruction.
Physical Findings
Cyanosis is evident on general examination. Differential cyanosis is usually not present. However, “reverse” differential cyanosis, for example, oxygen saturation higher in the lower extremities than upper extremities, may be present in newborns with transposition of the great arteries and a patent ductus arteriosus with pulmonary hypertension or coarctation of the aorta/interrupted aortic arch. Compromise in systemic perfusion, manifested by cool extremities and hypotension may be present if little mixing is present. In neonates with an intact ventricular septum, there is usually little or no murmur heard as the pulmonary vascular resistance is relatively high in the newborn period and the PDA is usually large. In the presence of a large VSD, retractions and hepatomegaly are evident as the pulmonary vascular resistance drops in the first few weeks of life. A harsh holosystolic murmur is heard at the left lower sternal border and is more evident as the pulmonary vascular resistance drops, along with a diastolic rumble at the apex. In the presence of left ventricular outflow tract obstruction, a harsh systolic ejection murmur of varying intensity depending on the degree of obstruction is heard at the left sternal border.
ECG (and Vector)
The electrocardiogram (ECG) demonstrates right atrial enlargement and right ventricular hypertrophy. Biventricular hypertrophy may be seen particularly in cases of left ventricular outflow tract obstruction or significant left ventricular volume overload. Patients with an intact ventricular septum have clockwise rotation of the horizontal QRS vector loop (42), usually directed rightward and anterior. Patients with a large VSD and/or left ventricular outflow tract obstruction may have counterclockwise horizontal plane QRS vector loops (42).
Radiography
The pulmonary vascular markings are normal to increased in patients with transposition of the great arteries and intact ventricular septum. In cases where the aorta and pulmonary trunk exhibit a direct (or somewhat direct) anterior–posterior relationship, the mediastinal shadow is narrow and not as prominent as usual, leading to the classic “egg on a string appearance” of the cardiac silhouette (Fig. 47.3). The pulmonary vascular markings are increased in patients with a large VSD and pulmonary overcirculation. Cardiomegaly is evident as well in these infants. Pulmonary vascular markings correlate inversely to the severity of left ventricular outflow tract narrowing in patients with transposition of the great arteries, ventricular septal defect and left ventricular outflow tract obstruction.
Echocardiography
In the current era, the diagnosis is confirmed by transthoracic echocardiography. Two-dimensional (2-D) echocardiography in the parasternal long-axis view demonstrates the pulmonary artery originating from the left ventricle coursing posteriorly (and bifurcating into branch pulmonary arteries) and the aorta originating from the right ventricle in the same plane coursing anteriorly (Fig. 47.4). Doppler interrogation shows flow into the posterior vessel (pulmonary artery) via the arterial duct. This is not seen in a normal posterior aorta. The origin of the pulmonary trunk from the left ventricle and the aorta from the right ventricle is also seen in other views, for example, in sweeps in the subcostal and parasternal apical chamber views. The parasternal short-axis view is helpful in determining the exact relationships of the aorta (identified as the great vessel from which the coronary arteries are seen originating) and pulmonary artery (identified as the great vessel that bifurcates) (Fig. 47.5), which can be variable.
A number of pertinent anatomic details should be obtained from transthoracic echocardiography that are relevant to the medical, interventional catheterization and surgical management of these patients. The size of the patent ductus arteriosus and direction of flow is important for medical management, prior to and while the patient is receiving prostaglandin infusion. Atrial level shunts, for example, a patent foramen ovale or secundum atrial septal defect, should be delineated, particularly with reference to overall anatomic adequacy of the size of the shunt/shunts and velocity of flow. Juxtaposition of the atrial appendages can be seen in transposition of the great arteries (37), with left-sided juxtaposition of the right atrial appendage being more common than right-sided juxtaposition of the left atrial appendage. The orifice of the juxtaposed right atrial appendage can be mistaken for large atrial septal defect (which, in a profoundly
cyanotic newborn with transposition of the great arteries, may be confusing and result in inappropriate delay in performing a balloon atrial septostomy). This may have important bearing on an eventual balloon atrial septostomy (detailed in “Balloon Atrial Septostomy” section). A number of important anatomic and presurgical details should be determined, including the relative size of the semilunar valve annuli and detection of abnormal semilunar valve morphology. The origins of the coronary arteries and their branching (and presence or absence of an intramural course) is an important finding that is accurately diagnosed by echocardiography (Fig. 47.6) (43,44).
In patients with transposition of the great arteries and a ventricular septal defect, the ventricular septal defect is usually a perimembranous one, but other defects in the ventricular septum can occur and should be sought. In patients with transposition of the great arteries, ventricular septal defect and left ventricular outflow tract obstruction, the precise mechanism of obstruction (e.g., valvar or subvalvar) must be delineated. Subvalvar left ventricular outflow tract obstruction may occur from posterior malalignment of the muscular outlet septum, ventricular septal hypertrophy, accessory AV valve tissue or from fibrous tissue/accessory tissue tags (35), and has bearing on the surgical procedure and timing of the surgical procedure that will eventually be performed.
cyanotic newborn with transposition of the great arteries, may be confusing and result in inappropriate delay in performing a balloon atrial septostomy). This may have important bearing on an eventual balloon atrial septostomy (detailed in “Balloon Atrial Septostomy” section). A number of important anatomic and presurgical details should be determined, including the relative size of the semilunar valve annuli and detection of abnormal semilunar valve morphology. The origins of the coronary arteries and their branching (and presence or absence of an intramural course) is an important finding that is accurately diagnosed by echocardiography (Fig. 47.6) (43,44).
In patients with transposition of the great arteries and a ventricular septal defect, the ventricular septal defect is usually a perimembranous one, but other defects in the ventricular septum can occur and should be sought. In patients with transposition of the great arteries, ventricular septal defect and left ventricular outflow tract obstruction, the precise mechanism of obstruction (e.g., valvar or subvalvar) must be delineated. Subvalvar left ventricular outflow tract obstruction may occur from posterior malalignment of the muscular outlet septum, ventricular septal hypertrophy, accessory AV valve tissue or from fibrous tissue/accessory tissue tags (35), and has bearing on the surgical procedure and timing of the surgical procedure that will eventually be performed.
Prenatal fetal echocardiography can readily identify fetuses with transposition of the great arteries, by identifying the origin of the great vessels from the ventricular chambers and by assessing the relationships of the aorta and pulmonary artery. This is advantageous in preparing and counseling families of the implications of the disease and its prognosis. Additionally, delivery at, or transport to a facility equipped to handle these newborns can be planned, along with appropriate postnatal management.
Magnetic Resonance Imaging and Computerized Tomography
Cardiac magnetic resonance imaging (MRI) and computed tomography (CT) scans are not needed for the diagnosis, but play an important role in the follow-up of these patients, particularly with regard to coronary issues (Fig. 47.7), branch pulmonary arteries (Figs. 47.7 and 47.8), the supravalavar neo-pulmonary valve region, and function of the semilunar valves (Fig. 47.8) in patients who underwent an arterial switch operation. Cardiac MRI and CT scans are also important in the follow-up of patients after atrial redirection procedures (discussed below). Brain MRI (preoperative and postoperative) may have a role in detection and follow-up of neurologic findings.
Cardiac Catheterization/Angiography
These days, cardiac catheterization is not needed for diagnosis. In a previous era, the “laid-back” angiogram was performed to evaluate the coronary arteries (45). This can also be performed if the patient undergoes a preoperative catheterization for other purposes, or the coronary artery pattern cannot be readily discerned by echocardiography (although rare), and the information is felt mandatory by the surgical team (not usually necessary). This is performed by angulating the frontal camera in an extreme caudal projection. A balloon occlusion angiogram in the ascending aorta with a catheter that has side holes proximal to the balloon, for example, a Berman angiographic catheter (Arrow International, Inc., Reading, PA) is performed from a transvenous approach (Fig. 47.9), which obviates the need to perform this retrograde from the femoral artery. This same angulation may be used from the femoral arterial approach after an arterial switch operation to examine the coronary arteries if needed. Selective coronary angiography can be safely performed even in very small infants.
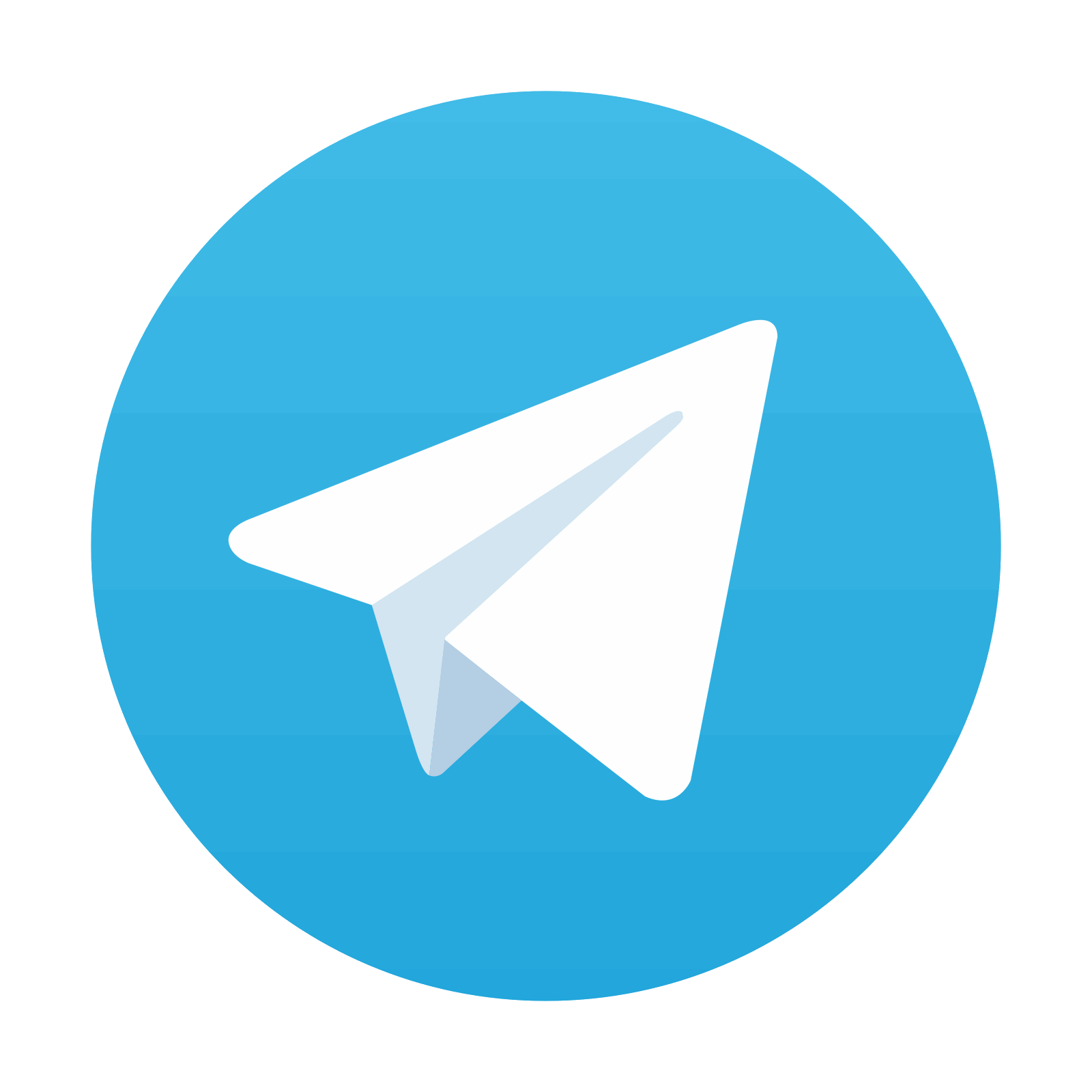
Stay updated, free articles. Join our Telegram channel

Full access? Get Clinical Tree
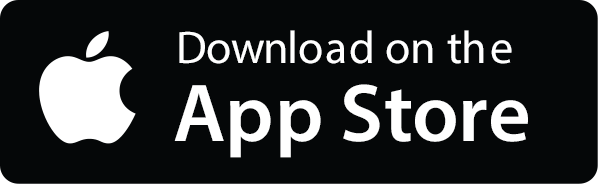
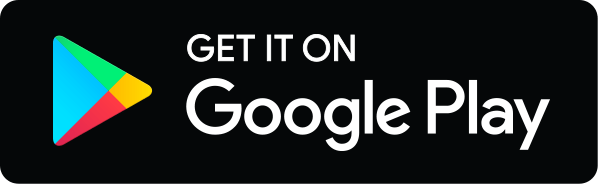