Preoperative Transcranial Doppler
Preoperatively, TCD can be combined with duplex ultrasonography to obtain valuable information for planning CEA. Duplex scanning of the extracranial carotid arteries is discussed in Chapter 8, and the basic principles of TCD are covered in Chapter 9. The TCD assessment prior to CEA includes the following.
a. Establishing the presence of adequate temporal acoustic windows for insonation of the middle cerebral artery (MCA) during surgery: In 8% to 10% of the general population, bone density does not allow the ultrasound beam to penetrate the skull, leading to failure of insonation with TCD. Inadequate acoustic windows are more common in women and in older subjects,12 and the failure rate has been reported to be higher in some series of CEA, most likely due to the relatively older age of the patient population.8,13
b. Evaluating the degree of stenosis and collateralization, including the status of the circle of Willis: A complete circle of Willis is present in about 30% to 40% of the general population, and the anterior communicating or posterior communicating arteries may be hypoplastic or nonfunctional. The degree of extracranial carotid stenosis and resulting collateralization can be qualitatively assessed by the “reversal of flow” phenomenon seen on TCD. The ophthalmic artery is one of the first branches from the intracranial ICA and forms collateral connections with the internal maxillary branch of the ECA. Flow in the ophthalmic artery is normally directed toward the eye; a reversal in the flow direction of the ophthalmic artery represents ECA to ICA collateralization.14
Preoperative TCD is a reliable tool for evaluation of the collateral supply through the circle of Willis in patients with ICA occlusion.15 A patent anterior communicating artery is indicated on TCD by a reversal of blood flow in the A1-segment of the anterior cerebral artery (ACA) or by a prompt fall in blood velocity in the middle cerebral artery (MCA) after compression of the nonoccluded contralateral carotid artery.15 Importantly, such carotid compressions must not be performed without first excluding plaques in the carotid system using duplex ultrasonography. In a study evaluating the collateral pathways through the circle of Willis with TCD and cerebral angiography in 40 patients with ICA occlusion, the sensitivity and specificity of TCD in detecting anterior communicating artery collateral supply were 95% and 100%, respectively.15 Collateral supply through the basilar artery is usually indicated by (i) a basilar artery blood velocity of more than 70 cm/s; (ii) a marked increase of basilar artery blood velocity after compression of the nonoccluded carotid artery; and (iii) an evident side-to-side asymmetry of the blood velocity in the posterior cerebral arteries with high velocity ipsilateral to the ICA occlusion.15 For evaluating collateralization via the basilar artery, the sensitivity and specificity of TCD have been reported to be 87% and 95%, respectively.15
c. Preoperative embolus detection: TCD is also valuable for preoperative detection of cerebral embolization from the carotid lesion and for monitoring therapeutic response using the embolus counts.16
d. Evaluation of hemodynamic significance of carotid stenosis and vasomotor reactivity: A reversal in the flow direction of the ophthalmic artery represents ECA to ICA collateralization and usually indicates that the lesion in the extracranial carotid artery is long standing and hemodynamically significant.14 A decrease of mean blood flow velocity more than 70% of the basal value during digital common carotid compression and a critical reduction of vasomotor reactivity (no significant increase of mean blood flow velocity in the MCA during the breath-holding test) also indicate a hemodynamically significant carotid stenosis.10
e. Detecting other intracranial vascular abnormalities: Preoperative TCD evaluation can also be useful for demonstrating stenosis of the MCA, carotid siphon, and other intracranial arteries.10
Although preoperative TCD may provide potentially useful information, some have questioned its utility, arguing that it is not a reliable enough method to modify operative strategy during carotid surgery.13 However, it is generally agreed that, coupled with arteriography, TCD is a good way to study cerebral hemodynamics.13
Intraoperative Transcranial Doppler
Intraoperative monitoring with TCD is aimed at detecting the vascular events leading to cerebral ischemia or hyperemia as they occur, so that appropriate interventions can be made to avoid permanent brain damage.
a. Probe placement and positioning: Typically, a 2-MHz pulsed Doppler ultrasound probe is used to insonate the ipsilateral MCA using standard vessel identification criteria. Some surgeons prefer to monitor the MCAs bilaterally. With either approach, it is important to maintain a constant angle of insonation throughout the operation in order to be sure that any observed velocity changes are related to the surgical procedure, because a change in the angle can affect the flow velocity. Hence, it is crucial that a fixation device be used to stabilize the probe position on the patient’s head and maintain a constant insonation angle during surgery. Figure 11.1 depicts application of the TCD probe with a head frame for a patient undergoing CEA.
b. Anesthetic considerations: Carotid surgery can be performed under regional or general anesthesia. It is important for the person interpreting TCD findings to be aware of the anesthetic technique being used for CEA because this may affect the cerebral blood flow velocities. In general, compared to the awake state, cerebral blood flow velocity decreases with induction of anesthesia, indicating decreased cerebral metabolic activity and flow-metabolism coupling.17,18 Thereafter, it remains stable unless the anesthetic depth or concentration of the anesthetic agent is changed. Increasing the concentration of volatile anesthetic agent to greater than 1.0 minimum alveolar concentration may increase the MCA velocity if the blood pressure is constant, indicating cerebral vasodilatation.19 On the other hand, intravenous anesthesia with propofol causes cerebral vasoconstriction and may decrease the MCA velocity.17
While there are no data supporting the relative superiority of one anesthetic agent over the other, it is important for the anesthesia provider to ensure that the anesthetic agent and concentration are not changed during crucial periods of the CEA to avoid interference with interpretation of TCD findings. Another physiologic parameter that may affect the interpretation of intraoperative TCD monitoring is the partial pressure of carbon dioxide.20 Since carbon dioxide is a powerful modulator of cerebrovascular tone, an increase in the partial pressure of carbon dioxide leading to cerebral vasodilatation may cause an increase in the MCA blood flow velocity and vice versa. Therefore, it is important for the anesthesiologist to ensure stable ventilation parameters and avoid inadvertent hypercapnia or hypocapnia during CEA.
c. Intraoperative monitoring for cerebral ischemia: Carotid cross-clamping during CEA can cause cerebral ischemia, and the primary goal of neuromonitoring is to determine the need for shunt insertion during the period of cross-clamping to carry blood from the CCA to the ICA and maintain cerebral perfusion. While shunting may prevent cerebral hypoperfusion due to cross-clamping, the routine use of an intraluminal shunt may increase the risk of perioperative stroke due to embolic events.21,22 Therefore, selective shunting guided by indicators of cerebral hypoperfusion during test clamping is often advocated.23 It should be acknowledged that there is no definitive evidence that selective shunting is better than routine shunting or nonshunting.24 However, whenever shunting is planned during CEA, TCD is a useful technique to guide the need for shunt insertion as well as to monitor shunt function.
Most surgeons monitor the MCA ipsilateral to the side of CEA. If insonation of the MCA is started with the patient awake, flow velocity monitoring demonstrates an expected decrease after anesthesia is induced. Thereafter, the velocity remains constant or drifts minimally with no decay over time during prolonged anesthesia.25 When TCD is used to determine the need for shunting, the immediate “preclamping” mean flow velocity and the relative change (or more specifically, the percent change) following clamping are used. The magnitude of the MCA mean flow velocity decrease following carotid cross-clamping depends on the status of the collateral vessels, and while this decrease may be small in patients with robust collaterals,21 in others a persistent drop to less than 15% of preclamp values and even complete loss of flow may be seen.21,26 Approximately 10% of patients exhibit an absolute intolerance to unilateral carotid clamping.27 Reported studies have indicated various threshold values for the critical decrease in MCA flow velocity that should trigger shunt insertion,8,9,21,28–31 and consequently, the TCD criteria for shunting vary among centers.
Electroencephalographic findings consistent with cerebral ischemia typically become evident when cerebral blood flow drops below 10 mL/100 g/min,28,29 which is consistent with an MCA mean flow velocity of 15 cm/s.28 In general, there appears to be good correlation between the decrease in MCA flow velocity and the frequency of EEG changes after carotid clamping.26 In the International Transcranial Doppler Collaborators study, cerebral ischemia was considered severe if the MCA mean flow velocity during the first minute after clamping was 0% to 15% of the preclamp value, mild if 16% to 40%, and absent if greater than 40%.21 These ranges were chosen early in this study and were influenced by ongoing correlative recordings of regional cerebral blood flow (rCBF), EEG, and carotid stump pressure made at some of the 11 participating centers, along with the perioperative rate of severe stroke attributable to intraoperative ischemia.21 Using the above TCD criteria for cerebral ischemia, it was observed that in patients with persisting ischemia (residual flow velocity 0% to 15%), the rate of severe stroke was very high and shunting was protective against stroke.21 At the same time, in patients with no ischemia (residual flow velocity >40%), the stroke rate was higher with shunting, although not as high as in the unshunted cases with severe ischemia.21
Similarly, Spencer et al30 using TCD and stump pressure measurements in 97 CEAs observed that a decrease of up to 60% in MCA mean flow velocity after carotid clamping was safe, and a residual mean flow velocity of less than 40% of the preclamp value required shunting.30 Others have recommended shunting for a drop in MCA mean flow velocity to less than 30% of the preclamping value.9,31 In a study of 48 patients undergoing CEA with regional anesthesia, a 48% relative reduction in MCA flow velocity after carotid clamping was found to detect clinical neurologic deterioration with 100% sensitivity and 86% specificity.8
In summary, most data suggest that a 40% to 50% reduction in the ipsilateral MCA mean flow velocity can be used safely as an indicator for selective shunting during carotid clamping. However, before attributing a decrease in flow velocity to carotid clamping, it should be verified that the angle of insonation, blood pressure, carbon dioxide levels, and anesthetic concentrations are stable. In addition to the flow changes in the ipsilateral MCA, if the contralateral ACA is simultaneously insonated during CEA, the mean flow velocity in the A1 segment of the contralateral ACA can be observed to increase almost instantaneously, within seconds of carotid clamping.32 The ACA velocity does not change thereafter during clamping, and then typically decreases after the clamp is released.32 A decrease in pulsatility index (indicating a drop in cerebrovascular resistance), is also observed in the MCA on the side of CEA.32
Following shunt insertion, TCD monitoring is helpful in documenting restoration of cerebral perfusion. Published data indicate immediate rebound of MCA flow velocity following shunting to between 50% and 120% of preclamp values.21,33 Monitoring should be continued after restoration of flow with a shunt because occasionally a shunt can malfunction due to blockage or kinking. In such situations, TCD can be helpful in early recognition of the problem based on an unexpected decrease in flow velocity.10,31,34,35 Augmentation of systemic blood pressure is often needed to maintain adequate blood flow through the shunt.36 Figures 11.2 and 11.3 demonstrate two different scenarios of serial changes in ipsilateral MCA flow velocity during CEA.
d. Intraoperative and postoperative monitoring for cerebral microemboli: The principal cause of stroke following CEA is embolism from the operative site.37,38 Although hemodynamic monitoring by itself cannot reduce perioperative stroke risk, the ability of TCD to detect microemboli is a unique advantage compared to other neuromonitoring techniques for CEA. Studies utilizing TCD have demonstrated that embolization occurs in more than 80% of patients over the course of a CEA.31,39,40 Intraoperative microembolism may be observed in 5% to 40% of patients during plaque dissection9,10,39–41 and in about 65% of patients during release of the carotid clamp.39,41 Microemboli may also be observed with shunting and following wound closure and restoration of blood flow39–42 and for up to 24 hours postoperatively.43,44 About 48% of patients have been reported to have one or more emboli detected in the postoperative period, with the majority of these occurring in the first 2 hours.43
Monitoring of the MCA using TCD during CEA allows real-time detection of cerebral microemboli. These microemboli can be recognized on TCD as high-intensity transient signals (HITS), and there are potential implications for the number of emboli observed as well as their differentiation into air and particulate types. In general, the majority of emboli observed during the arterial dissection before the arteriotomy and about one-third of those observed immediately after shunt insertion are particulate.41,45 However, the majority of emboli detected in the cerebral circulation after release of the carotid clamp are air, with less than 10% being particulate.45 It is likely that air emboli, while commonly observed, may have minimal pathologic consequences. Finally, during completion of the operation and closure, particulate emboli are more common. The TCD characteristics of HITS from particulate microemboli are illustrated in Figure 11.4 and include the following46:
- Intensity greater than 3 dB above background velocity spectrum
- Duration less than 0.3 seconds
- Unidirectional within the flow velocity spectrum
- Characteristic “chirping,” “snapping,” or “moaning” sound
It is important to note that HITS on TCD may also be produced as artifacts caused by probe movement, electrical stimuli, or diathermy, and these should be differentiated from microemboli. Bidirectional signatures in the flow velocity waveform are usually artifacts, although air emboli can sometimes produce similar signatures (Fig. 11.5). The major feature of air emboli that makes them grossly distinguishable from particulate emboli is their large dynamic range of around 60 dB compared to the 10 to 15 dB range for particulate emboli (Fig. 11.6). Most commercially available TCD instruments are “overloaded” by signals of such dynamic range, and therefore, air emboli result in signatures that extend outside the normal velocity spectral waveform. This distinction may work for bubbles down to approximately 30 μm in size but not smaller. Although investigators have suggested various techniques, such as the dual gating method and Wigner spectral analysis, to differentiate between air and particulate emboli,45 the currently available technology is unable to reliably make this distinction. However, the very high intensity signatures of air emboli, which typically overload the dynamic range of most TCD machines, generally produce some aliasing. On the other hand, the high intensity signals produced by particulate emboli are contained within the velocity waveform and are relatively subtle findings (Fig. 11.4).
Since the first report of the detection of microemboli in the MCA during and in the early postoperative period after CEA,47 attempts have been made to correlate the number of microemboli detected in a given time period with postoperative neurologic deficits and silent infarction demonstrated on brain imaging. In one of the first attempts to quantify the number of solid microemboli occurring during CEA and determine their clinical significance, Ackerstaff et al41 noted that microembolism did not result in new morphologic changes in postoperative computed tomography scans, although there was a correlation between more than 10 microemboli during dissection and new lesions on postoperative T2-weighted MRI scans. Others have noted a microembolization rate of greater than 20/hour to be associated with ischemic changes on MRI.48 In a series of 65 patients where microembolic signals (MES) were detected in 69% of the cases during the first hour postoperatively with counts ranging from 0 to 212/hour, the occurrence of greater than 50 MES/hour in the early postoperative phase was predictive of the development of ipsilateral focal cerebral ischemia.44 In another study of 81 consecutive patients undergoing CEA with TCD monitoring, Levi et al49 detected postoperative MES at a rate of more than 50/hour in 8 cases, with 5 of them suffering ischemic neurologic deficits in the territory of the insonated MCA, indicating a strong association between an early postoperative MES count of greater than 50/hour and the development of early cerebral ischemia.
The significance of detecting cerebral microemboli is often questioned because by the time the emboli are detected, they are already in the cerebral circulation. It has been suggested that surgeons could be guided by the documentation of embolic signals to change their operative technique, with the expectation that a decrease in the rate of microembolism would result in a decline in the perioperative stroke rate.31,41 Moreover, postoperative emboli detection can be used as an indication to intervene with heparin or Dextran to reduce embolization and the consequences thereof.43,50 In a prospective series of 100 patients who underwent CEA with a 6-hour period of postoperative TCD monitoring, Dextran 40 infusion was started if ≥25 emboli were detected in any 10-minute period.43 While only five patients developed sustained embolization, in each of these cases, embolization was abolished by Dextran administration, and during the period of this protocol, there was a 0% perioperative morbidity and mortality compared to the same group’s previous postoperative thromboembolic stroke rate of 3%.43
Other options to decrease cerebral emboli include a combination of clopidogrel or Dextran 40 with dipyridamole/aspirin; however, there has been no difference in the influence of various antiplatelet regimens on embolization detected by TCD following CEA.51 Subsequent studies have shown that clopidogrel administered the night before surgery, in addition to daily aspirin, significantly reduces postoperative embolization and Dextran utilization.52 Even when antiplatelet therapy is used preoperatively, TCD monitoring remains useful for determining the need for additional measures such as Dextran 40 in patients who continue to have microemboli during or after CEA.
FIGURE 11.1. TCD probe secured over the temporal acoustic window using a commercially available head frame to stabilize the angle of insonation during CEA. The patient is under general anesthesia and intubated.
FIGURE 11.2. Serial changes in ipsilateral MCA flow velocity during a CEA. A,Preclamp mean flow velocity 50.4 cm/s. B,Postclamp mean flow velocity 22.7 cm/s (45% of preclamp value). C,Shunt insertion leads to restoration of flow velocity close to the preclamp value (54.7 cm/s), and D,Transient hyperemia following completion of the endarterectomy and unclamping of the internal carotid. This patient may not have required a shunt based on the International Transcranial Doppler Collaborators criteria for cerebral ischemia (postclamp flow velocity <40% of preclamp value), but the surgeon elected to shunt.
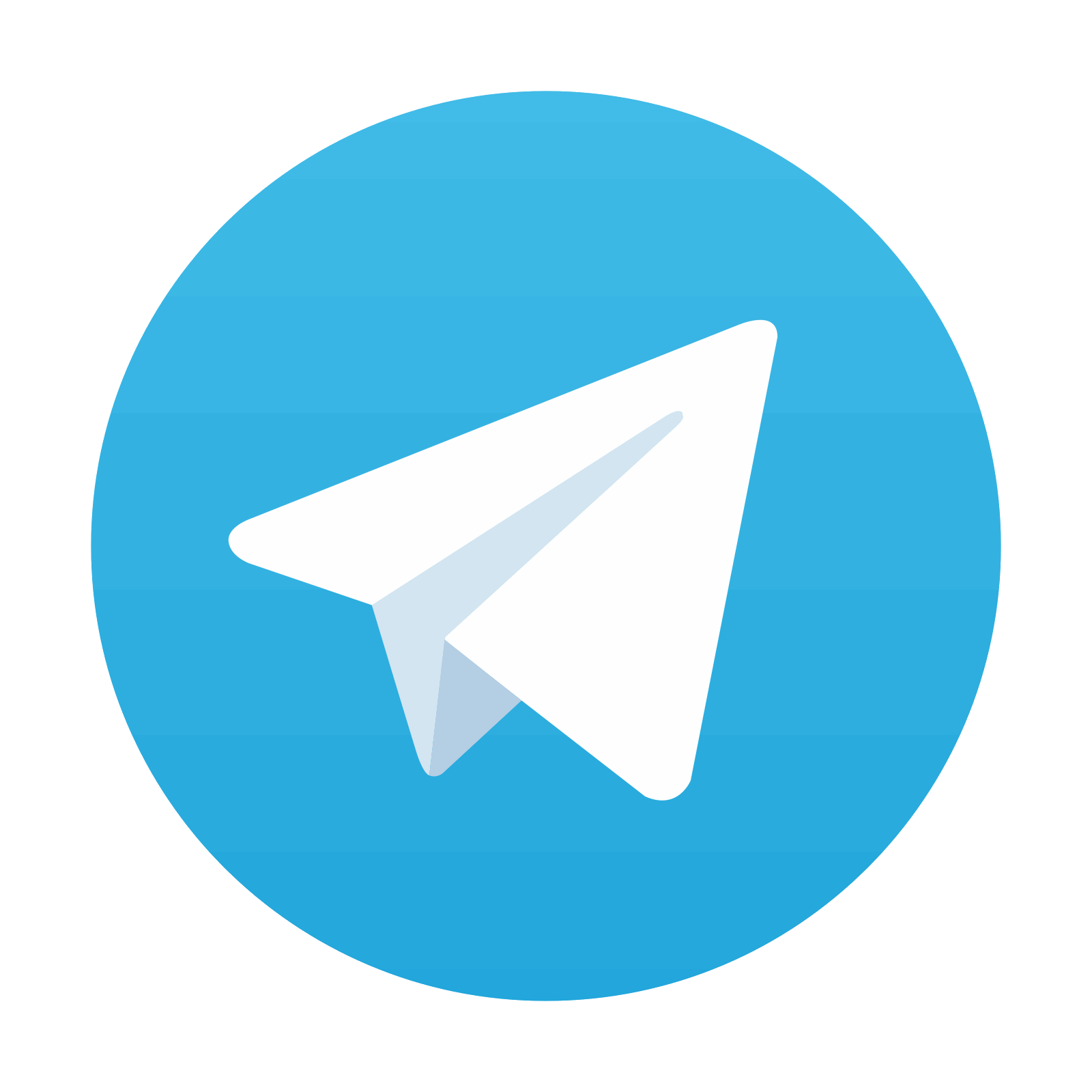
Stay updated, free articles. Join our Telegram channel

Full access? Get Clinical Tree
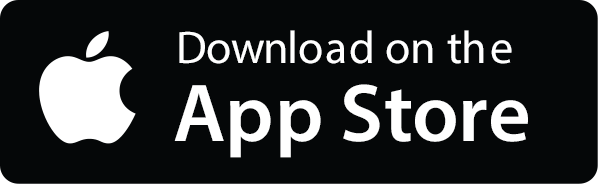
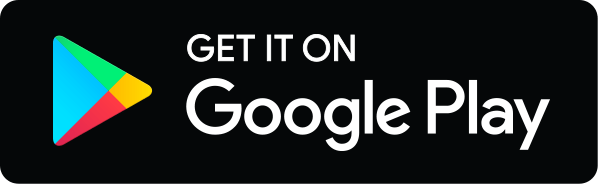