Background
The aim of this study was to evaluate the timing and magnitude of global and regional right ventricular (RV) function by means of speckle tracking–derived strain in normal subjects and patients with RV dysfunction.
Methods
Peak longitudinal systolic strain (PLSS) and time to PLSS in 6 RV segments (the basal, mid, and apical segments of the RV free wall and septum) were obtained in 100 healthy volunteers and 76 patients with RV dysfunction by tracking speckles inside the myocardium using grayscale images. Global PLSS and time to PLSS were based on the average of the 6 regional values.
Results
There was a significant and close correlation between RV contractility as measured by PLSS and tricuspid annular plane systolic excursion ( r = −0.83, P < .001). In normal subjects, PLSS was significantly greater in the free wall than in the septum (−28.7 ± 4.1% vs −19.8 ± 3.4%, P < .001), whereas time to PLSS was similar in the different regions of the right ventricle. In patients with RV dysfunction, global and regional PLSS was significantly less than in normal subjects (−13.7 ± 3.6% vs −24.2 ± 2.9%, P < .001), and a global PLSS cutoff value of −19% was helpful in distinguishing the two groups. Furthermore, time to PLSS in all of the RV septal segments and dispersion in RV contraction timing were significantly longer. Global PLSS in the patients with RV dysfunction was also significantly less in the presence of moderate to severe pulmonary hypertension (−12.7 ± 3.6% vs −14.4 ± 3.4%, P = .038).
Conclusions
Speckle tracking not only makes it possible to quantify global RV function but also illustrates the physiology of RV contraction and the pattern of activation at regional level. Speckle tracking–derived strain could become an important new means of assessing and following up patients with impaired RV function and increased pulmonary pressure.
The assessment of right ventricular (RV) function is of paramount importance in many cardiovascular and pulmonary diseases. However, conventional two-dimensional echocardiography does not allow a comprehensive evaluation because of the right ventricle’s complex, crescent-shaped structure, wrapped around the left ventricle. Global RV function is most frequently assessed by means of RV fractional area change (FAC) and tricuspid annular plane systolic excursion (TAPSE), although both techniques have intrinsic limitations.
The new echocardiographic method of speckle tracking assesses myocardial deformation or strain by tracking speckles in the myocardium on grayscale (B-mode) images and can be used to evaluate both global and regional myocardial strain without being limited by the Doppler beam angle. A number of studies have used speckle tracking–derived strain to evaluate the left ventricle, but there is relatively little information concerning the assessment of RV performance. Its feasibility and reproducibility have been demonstrated in selected populations of athletes and in subjects with congenital heart diseases, systemic sclerosis, acute pulmonary thromboembolism, and pulmonary arterial hypertension. However, the magnitude and timing of regional RV contraction in patients with RV dysfunction associated with left-sided heart failure have not been investigated and compared with these values in normal subjects.
The aims of this study were (1) to compare global RV systolic function as evaluated on the basis of speckle tracking–derived strain with that revealed by the conventional parameters of RV contractility (TAPSE and RV FAC), (2) to assess the timing and magnitude of speckle tracking–derived regional RV strain in normal subjects and patients with RV dysfunction, and (3) to compare the timing and magnitude of global and regional RV strain in patients with RV dysfunction with and without pulmonary hypertension.
Methods
Study Population
Between January and December 2007, 100 healthy volunteers (defined as having no histories of cardiac disease, alcohol or drug abuse, and pathologic physical examinations or abnormal 12-lead electrocardiographic findings) were prospectively enrolled at Fondazione Cardiocentro Ticino in Lugano, Switzerland. Subjects aged >40 years were eligible only if they had negative results on scintigraphy, or the absence of coronary artery disease (CAD) was documented by angiography or 64-multislice computed tomography. Subjects with any evidence of abnormal global or regional left ventricular (LV) and/or RV wall motion or any hemodynamically relevant valve abnormalities were also excluded.
From a larger group of consecutive patients with LV dysfunction (defined by an ejection fraction of <35%), 85 consecutive patients with RV dysfunction were identified on the basis of TAPSE < 2 cm. These patients were further divided into those with or without pulmonary hypertension using a cutoff value of pulmonary artery pressure in systole of 35 mm Hg. Subjects with atrial fibrillation or who had undergone previous tricuspid valve surgery were excluded. Nine patients were excluded from the analyses because the quality of their echocardiographic studies was found to be insufficient upon review. In the remaining population, it was not possible to analyze the RV apical free wall segment in 4 patients and the apical septum in 2 patients.
The final study population therefore consisted of 100 healthy subjects and 76 patients with RV dysfunction, 45 (59%) with normal or only slightly high pulmonary pressure and 31 (41%) with moderate or severe pulmonary hypertension. All subjects gave informed consent to participate in the study, which was approved by our institutional review board.
Echocardiographic Measurements
Echocardiographic examinations were performed using a GE Vivid 7 machine (GE Healthcare, Milwaukee, WI) with the subjects in the left lateral position. Images were acquired during breath holds with stable electrocardiographic recordings and were then digitally stored for subsequent offline analysis using EchoPAC software (GE Healthcare). LV volume and the ejection fraction were measured using Simpson’s biplane method in accordance with current guidelines. RV FAC was obtained by measuring RV area at end-diastole and end-systole in the 4-chamber view and calculating the ratio between the difference between end-diastolic and end-systolic area divided by the end-diastolic area ([end-diastolic area − end-systolic area]/end-diastolic area). TAPSE was obtained by drawing a straight line (M-mode) through the lateral tricuspid valve annulus and was quantified as the length of the excursion of the tricuspid annular plane during systole in millimeters.
RV strain was analyzed using conventional two-dimensional echocardiographic grayscale apical 4-chamber images and a frame rate of 70 to 80 frames/s, which seems to be the best compromise between appropriate temporal resolution and acceptable spatial definition of the LV lateral wall and RV free wall. In postprocessing analysis, the region of interest was obtained by tracing the RV endocardial borders at the level of the septum and the free wall in a still frame at end-systole. An automated software program calculated the frame-to-frame displacements of speckle patter within the region of interest throughout the cardiac cycle. Longitudinal strain curves were obtained for 6 RV segments (the basal, mid, and apical segments of the RV free wall and septum), and the global RV strain curve was based on the average of the 6 regional strain curves ( Figure 1 ); longitudinal strain curves of the lateral LV wall were obtained by repeating the same analysis. The extent of myocardial deformation (defined as the peak longitudinal systolic strain [PLSS]) was expressed as a percentage of the longitudinal shortening in systole compared with diastole for each segment of interest. The temporal pattern of RV mechanical contraction was evaluated as the time needed to reach peak strain (time to PLSS) using the beginning of the QRS complex as a reference point. The dispersion in contraction timing (calculated as the SD of the time to PLSS in the different RV regions) therefore represented right intraventricular synchronicity. Interventricular synchronicity was defined as the maximum difference in time to PLSS between lateral LV and the free RV wall.

Statistical Analyses
Continuous baseline data are expressed as mean ± SD and discrete variables as absolute numbers and percentages. The normality ranges of PLSS and timing were defined as ±2 SDs of the mean in the normal subjects. The groups were compared using 2-sample Wilcoxon’s rank-sum (Mann-Whitney) tests and multivariate linear regression analyses, as appropriate. The relationships between RV strain and the traditional measurements of RV function (ie, TAPSE and RV FAC) were tested by means of Pearson’s correlation. The cutoff value for RV strain that was most sensitive and specific in measuring the RV systolic function was determined by means of receiver operating characteristic curve analysis.
Twenty of the normal subjects and 20 of the patients with RV dysfunction were randomly selected, and the measurements were repeated after 1 month by the same echocardiographer to assess intraobserver reproducibility. Interobserver reproducibility was assessed by having a second echocardiographer repeat the measurements of PLSS and time to PLSS in 15 of the subjects from each group. Intraobserver and interobserver reproducibility (with 95% agreement limits) were analyzed using method of Bland and Altman.
All P values were 2 sided, and values < .05 were considered statistically significant. All statistical analyses were performed using Stata software (StataCorp LP, College Station, TX).
Results
Baseline Characteristics
Table 1 shows the baseline characteristics of the normal subjects and the patients with RV dysfunction. The normal subjects were younger and more likely to be female. As expected, the patients with RV dysfunction had larger left and right ventricles and depressed LV function. Among the patients with reduced TAPSE, RV FAC and RV fractional shortening were equally abnormal, and about 40% had pulmonary hypertension.
Variable | Normal subjects (n = 100) | Patients with RV dysfunction (n = 76) | P |
---|---|---|---|
Clinical variables | |||
Age (y) | 43 ± 13 | 68 ± 12 | <.001 |
Women | 46 (46%) | 13 (17%) | <.001 |
Echocardiographic variables | |||
LV dimensions and function | |||
LV ESV (mL) | 29 ± 9 | 131 ± 47 | <.001 |
LV EDV (mL) | 84 ± 22 | 180 ± 57 | <.001 |
LV EF (%) | 66 ± 5 | 28 ± 6 | <.001 |
RV dimensions and function | |||
RV diastolic diameter (cm) | 3.0 ± 0.3 | 3.0 ± 0.4 | .185 |
RV systolic diameter (cm) | 1.9 ± 0.3 | 2.3 ± 0.4 | <.001 |
RV FS (%) | 34 ± 5 | 26 ± 8 | <.001 |
RV diastolic area (cm 2 ) | 21 ± 4 | 23 ± 5 | .005 |
RV systolic area (cm 2 ) | 11 ± 3 | 16 ± 4 | <.001 |
RV FAC (%) | 45 ± 6 | 31 ± 7 | <.001 |
TAPSE (cm) | 2.5 ± 0.4 | 1.4 ± 0.3 | <.001 |
Tricuspid valve and pulmonary pressure | |||
Moderate to severe TR | 0 (0%) | 4 (5%) | .570 |
Systolic PAP > 35 mm Hg | 0 (0%) | 31 (41%) | <.001 |
RV Global Function
The normal subjects’ mean global PLSS of the 6 RV segments (basal, mid, and apical septum and free wall) was –24.2 ± 2.9% (range, −30.0% to −17.7%), significantly higher than in patients with RV dysfunction (mean, −13.7 ± 3.6%; P < .001).
There were good correlations between global PLSS and TAPSE ( r = −0.83, P < .001) and between global PLSS and RV FAC ( r = −0.73, P < .001) in the study population as a whole, as well as in the group of normal subjects (PLSS and TAPSE, r = −0.37, P < .001; PLSS and RV FAC, r = −0.16, P = .119) and the group with RV dysfunction (PLSS and TAPSE, r = −0.33, P = .004; PLSS and RV FAC, r = −0.38, P < .001) ( Figure 2 ).
The receiver operating characteristic curves showed that with TAPSE as the reference parameter, the most sensitive and specific global PLSS cutoff value was −19%, with sensitivity of 95% (95% confidence interval [CI], 87%-98%) and specificity of 85% (95% CI, 77%-91%). With RV FAC as the reference parameter, the best cutoff value was −16%, with sensitivity of 90% (95% CI, 77%-97%) and specificity of 83% (95% CI, 76%-88%) ( Figure 3 ).
RV Regional Function
Table 2 shows the ranges of regional PLSS in the normal subjects. PLSS was significantly higher in the free wall than in the septum (−28.7 ± 4.1% vs −19.8 ± 3.4%, P < .001). There was no significant difference between the apical and basal RV segments (−24.2 ± 6.1% vs −23.5 ± 4.1%, P = .375).
Variable | PLSS (%) | Time to PLSS (ms) |
---|---|---|
Global | −30.0 to −17.7 | 302 to 474 |
Basal free wall | −43.2 to −14.9 | 284 to 511 |
Mid free wall | −40.9 to −20.1 | 284 to 505 |
Apical free wall | −39.01 to −13.1 | 285 to 468 |
Basal septum | −26.8 to −12.5 | 283 to 494 |
Mid septum | −27.3 to −12.7 | 291 to 484 |
Apical septum | −33.6 to −9.7 | 294 to 467 |
Free wall segments | −37.7 to −19.8 | 287 to 482 |
Septal segments | −27.0 to −12.8 | 288 to 480 |
Apical segments | −35.5 to −12.2 | 293 to 460 |
Basal segments | −33.5 to −14.6 | 300 to 491 |
SD | 4.29 to 68.09 | |
Interventricular time | −158 to +94 |
Figure 4 A shows a comparison of global and regional PLSS between normal subjects and patients with RV dysfunction. PLSS of the free wall and septal segments was significantly lower in the group with RV dysfunction ( P < .001). On multivariate analyses using age, gender, and LV end-diastolic volume as covariates, PLSS of the free wall and septum and global PLSS were all lower than in normal subjects ( P = .018, P = .044, and P = .059, respectively).
Time to PLSS
Table 2 also shows the ranges of global and regional time to PLSS in the normal subjects. RV strain usually peaked between 302 and 474 ms after the beginning of QRS, and contraction of the free wall and septum occurred approximately simultaneously (388 ± 43 vs 385 ± 42 ms, P = .351); similarly, there was no significant difference in RV activation between the apex and base (384 ± 38 vs 391 ± 46 ms, P = .073).
As shown in Figure 4 B, time to PLSS was generally longer in patients with RV dysfunction (416 ± 75 vs 387 ± 39 ms, P < .001). There was no significant difference in the mean free wall time to PLSS between normal subjects and patients with RV dysfunction (388 ± 43 vs 394 ± 73 ms, P = .515), except at the apex, whereas the mean time to PLSS in all of the septal segments was significantly shorter in normal subjects (385 ± 43 vs 438 ± 99 ms, P < .001). However, multivariate analyses using age, gender, and LV end-diastolic volume as covariates showed that global and regional time to PLSS were no longer independent determinants of differences between the two groups.
The SD of time to PLSS was significantly larger in the patients with RV dysfunction (81 ± 29 vs 26 ± 14 ms, P < .001) and was an independent determinant of between-group differences on multivariate analysis ( P = .013). The RV free wall contracted earlier than the LV lateral wall in normal subjects but significantly later in patients with RV dysfunction (−11 ± 62 vs 91 ± 153 ms, P < .001).
Electrocardiographic Conduction Disturbances
The duration of the QRS interval was <100 ms in the normal subjects and 133 ± 33 ms in patients with RV dysfunction, of whom 50 (66%) had QRS intervals > 120 ms and 26 (34%) had QRS intervals < 120 ms. There were no differences in global PLSS, time to PLSS, or the SD of time to PLSS between patients with QRS intervals < 120 ms or > 120 ms, but interventricular dyssynchrony was significantly greater in the patients with QRS intervals > 120 ms (121 ± 137 vs 52 ± 117 ms, P = .032).
Etiology of RV Dysfunction
As stated in the inclusion criteria, all patients with RV dysfunction had concomitant left-sided heart failure: 46 (60%) had CAD, 22 (29%) had idiopathic cardiomyopathy, and 8 (11%) had biventricular dysfunction due to other etiologies (valvular disease [n = 4], hypertrophic cardiomyopathy [n = 2], and toxic cardiomyopathy [n = 2]). Global and septal PLSS were significantly greater in patients with CAD than in nonischemic patients (−14.7 ± −4.1% vs −12.9 ± −3.1%, P = .041; and −10.6 ± 4.0% vs −8.4 ± 3.1%, P = .012), but the differences in TAPSE, RV FAC, and free wall PLSS were not statistically significant. There were no significant differences in global or regional time to PLSS between these subgroups.
Magnitude and Timing of RV Strain in the Presence of Pulmonary Hypertension
On the basis of a cutoff value of 35 mm Hg, 45 of the patients with RV dysfunction (59%) had normal or only slightly high pulmonary pressure, and 31 (41%) had moderate to severe pulmonary hypertension ( Table 3 ). Both univariate and multivariate analysis (with age, LV end-diastolic volume, LV ejection fraction, the etiology of biventricular failure, and QRS duration as covariates) showed that the patients with pulmonary hypertension had significantly lower global PLSS but no significant segmental reductions or any significant differences in time to global and regional PLSS.
Systolic PAP (mm Hg) | P | |||
---|---|---|---|---|
Variable | <35 | >35 | Unadjusted | Adjusted ∗ |
PLSS (%) | ||||
Global | −14.4 ± 3.4 | −12.7 ± 3.6 | .038 | .037 |
Basal free wall | −18.8 ± 7.3 | −18.6 ± 7.2 | .913 | .864 |
Mid free wall | −19.3 ± 5.7 | −16.2 ± 6.3 | .030 | .058 |
Apical free wall | −17.3 ± 6.2 | −15.4 ± 6.0 | .183 | .239 |
Basal septum | −10.3 ± 4.9 | −8.6 ± 5.3 | .149 | .061 |
Mid septum | −9.2 ± 3.7 | −7.7 ± 4.2 | .105 | .084 |
Apical septum | −11.5 ± 4.7 | −9.5 ± 7.0 | .129 | .497 |
Time to PLSS (ms) | ||||
Global | 425 ± 71 | 403 ± 79 | .217 | .299 |
Basal free wall | 378 ± 89 | 391 ± 76 | .512 | .788 |
Mid free wall | 380 ± 78 | 379 ± 85 | .928 | .549 |
Apical free wall | 421 ± 89 | 425 ± 116 | .842 | .809 |
Basal septum | 449 ± 122 | 371 ± 129 | .009 | .045 |
Mid septum | 457 ± 120 | 391 ± 138 | .029 | .131 |
Apical septum | 465 ± 87 | 470 ± 98 | .820 | .832 |
SD | 78 ± 30 | 86 ± 27 | .230 | .167 |
Traditional parameters of RV contraction | ||||
TAPSE (cm) | 1.4 ± 0.2 | 1.3 ± 0.3 | .047 | .167 |
RV FAC (%) | 33 ± 7 | 28 ± 7 | .008 | .013 |
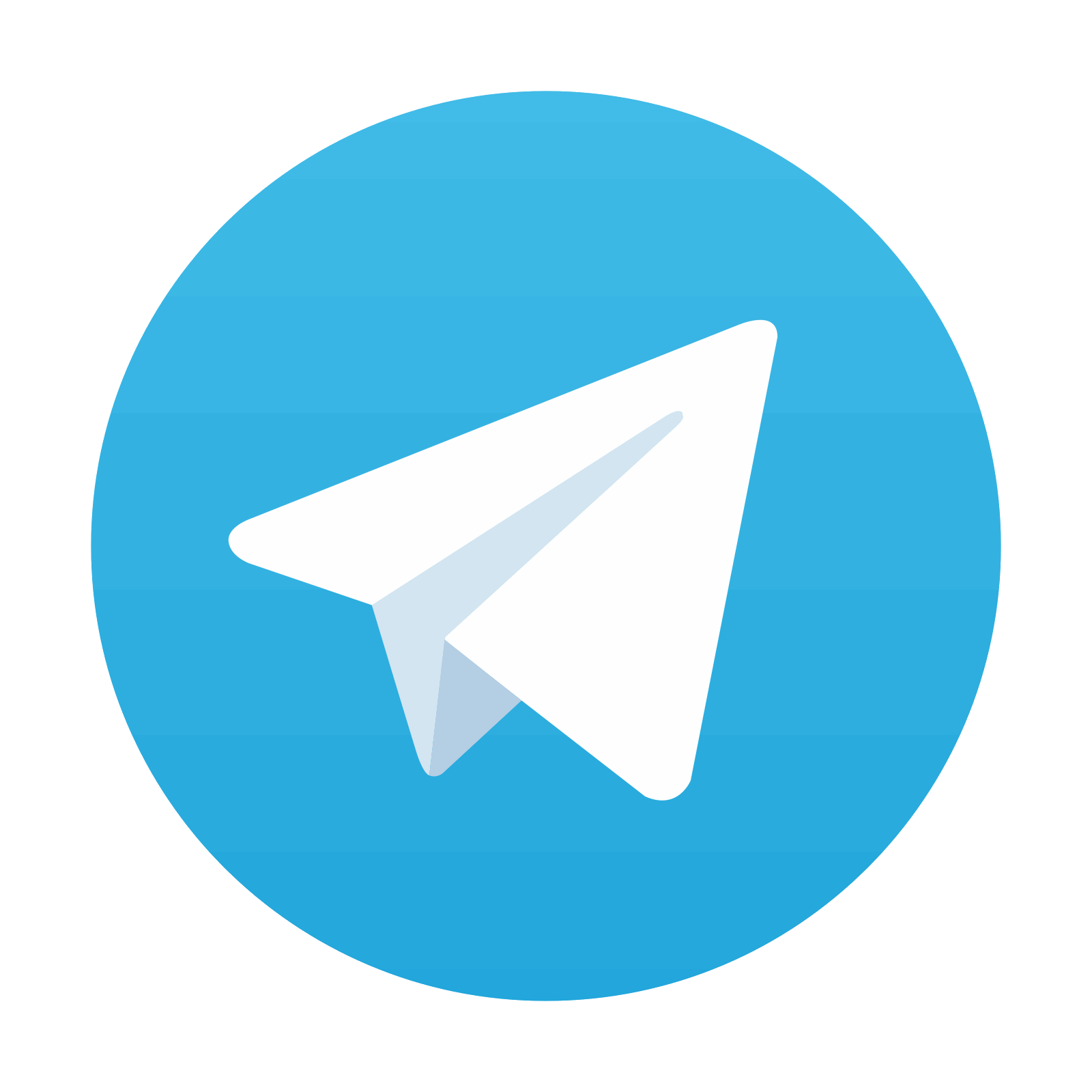
Stay updated, free articles. Join our Telegram channel

Full access? Get Clinical Tree
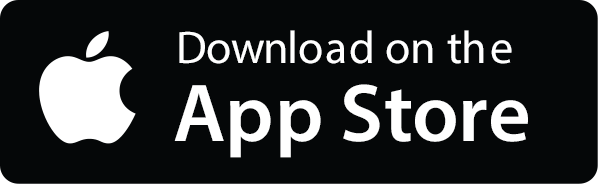
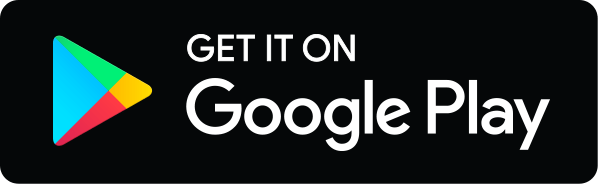
