With the continued development of genetically engineered mouse models of cardiac disease, further advancement of noninvasive techniques for evaluating cardiac diastolic dysfunction in these models would be valuable. Therefore, we performed comprehensive transmitral and pulmonary venous Doppler echocardiographic studies to devise novel indices of diastolic function in a mouse model with cardiac hypertrophy, which were validated against invasively measured hemodynamic parameters. We examined 10 HopX Tg transgenic mice with diastolic dysfunction and 10 age-matched controls sedated with 1% to 2% isoflurane (male, age 14–18 weeks). These studies revealed that the acceleration time of the transmitral Doppler E-wave was the best Doppler parameter for unmasking LV diastolic dysfunction in HopX Tg mice. This is the first study to assess the utility of the acceleration time of the E-wave and pulmonary venous Doppler echocardiography as a primary diagnostic modality for assessing murine diastolic function.
Over the past two decades, the prevalence of heart failure due to diastolic dysfunction has been gradually increasing. Despite the growing incidence of this disorder, no effective therapies exist to treat the disease, halt its progression, or reduce the associated mortality. The development of transgenic and gene targeting techniques in mice has significantly expanded our understanding of disease-causing pathways and increased the availability of faithful models for evaluating clinical therapies. Several groups have developed genetically engineered mice with impaired myocardial relaxation, and some investigators have used these models to assess therapeutic strategies for treating myocardial diastolic dysfunction. Transmitral Doppler echocardiography has been routinely used to identify left ventricular (LV) diastolic dysfunction in patients, and many of these indices have been validated against invasive hemodynamic measurements in small animal models. In addition, several groups have used high-resolution ultrasound systems to study mouse cardiac structure and function. This technology may provide better spatial and temporal resolution compared with conventional echocardiographic equipment. However, problems related to the complexity of interpreting the transmitral flow profile still exist, and some of the better established clinical indices may need to be reevaluated for their relevance in murine models because of interspecies differences in cardiac physiology. The main difference that affects interpretation of the murine transmitral inflow profile is the higher heart rate, which often fuses the E-wave and A-wave. Although pulmonary venous Doppler echocardiography is routinely used in the clinical setting to assess diastolic function, no detailed studies have reported the utility of pulmonary venous Doppler echocardiography for evaluating murine diastolic function. To address these questions, we performed a comparative study of transmitral and pulmonary venous Doppler echocardiography and compared these with invasive measurements of left ventricular end-diastolic pressure (LVEDP), -dP/dt min , and tau in a genetically engineered mouse model with diastolic dysfunction. These investigations revealed that the acceleration time of the transmitral Doppler E-wave is the best parameter for assessing LV diastolic dysfunction in HopX Tg mice. This is the first study to demonstrate that the acceleration time of the transmitral Doppler E-wave may be useful for assessing diastolic function in murine models. Although this study did not uncover any pulmonary venous Doppler parameters that correlated with invasive parameters of diastolic dysfunction in HopX Tg mice, the comprehensive evaluation of pulmonary venous Doppler analysis described in this report will likely be useful for assessing diastolic dysfunction in other mouse models.
Materials and Methods
Animals
Homeodomain-only protein transgenic mice ( HopX Tg ) develop cardiac hypertrophy with cardiomyocyte enlargement as the result of HDAC recruitment, as previously described. We studied 10 HopX Tg and 10 age-matched wild-type (WT) mice weighing 25 to 35 g and aged 14 to 18 weeks. All protocols used conformed to the guidelines established by the Association for the Assessment and Accreditation of Laboratory Animal Care and were approved by the University of Pennsy-lvania Animal Care and Use Committees. This investigation conforms to the Guide for the Care and Use of Laboratory Animals published by the US National Institutes of Health (Publication No. 85-23, revised 1996).
Transthoracic Echocardiographic Imaging
A comprehensive transthoracic two-dimensional and Doppler echocardiographic study was conducted using a high-resolution (30 MHz) Vevo 770 imaging system (VisualSonics Inc., Toronto, Canada) as previously described. Mice were lightly anesthetized with a mixture of 1% to 2% isoflurane gas and 100% oxygen while supine on a heated platform. Several studies have compared different anesthetic regiments on murine cardiovascular function, and inhaled anesthetics seem to be the most advantageous because they have the least cardiodepressive effects with a relatively short half-life. Furthermore, we compared 1% isoflurane versus 2% isoflurane on the echocardiographic and invasive parameters we measured in a subset of HopX Tg and WT mice, and found no significant differences in these measurements at either dose, suggesting the cardiovascular effects of isoflurane are close to maximal at a concentration of 1% in these mice ( Appendix 1 ). The mouse’s core temperature was monitored using a rectal temperature probe, and an infrared heating lamp was used to maintain body temperature at 37°C ± 0.5°C throughout the procedure. An electrocardiogram signal was also monitored through electrode pads on the heated platform. Chest hairs were removed using a chemical depilator (Nair, Church & Dwhite Co. Inc., Princeton, NJ) to minimize ultrasound attenuation. The ultrasound probe (RMV-707B, VisualSonics Inc., Toronto, Ontario, Canada) was placed on the mouse’s chest using warm ultrasound gel as a coupling medium.
Two-Dimensional Echocardiography
Two-dimensional images were recorded in the parasternal long- and short-axis views to guide M-mode recordings obtained at the mid-ventricular level. Wall thicknesses of the interventricular septum and LV posterior wall, as well as the LV diastolic and systolic internal dimensions, were measured, and the average values from both views are reported. LV systolic function was computed from the M-mode measurements according to the recommendations of the American Society of Echocardiography Committee. Relative wall thickness was calculated using the formula: (interventricular septum dimension + LV posterior wall dimension)/LV diastolic internal dimension, and left atrial (LA) area was measured in the 4-chamber view during LV systole using the polygon region of interest area measurement tool in the Visualsonics software suite (Visualsonics Standard Measurements, version 23, VisualSonics Inc.) with the program’s B-mode generic measurement feature.
Transmitral Doppler Echocardiography
Pulsed Doppler studies of LV diastolic function were performed in the apical 4-chamber view with the Doppler cursor oriented parallel to the long-axis plane of the left ventricle. The sample volume was placed just below the level of the mitral annulus and adjusted to render the highest early diastolic flow velocity peak of the transmitral Doppler flow signal. There was often a need for angle correction, which was always less than 20 degrees. The early and late diastolic peak velocity (E, A) and their ratio (E/A) were derived from the transmitral Doppler waveform. Left ventricular systolic intervals of the isovolumic contraction time, the ventricular ejection time, diastolic intervals of the isovolumic relaxation time (IVRT), and the acceleration and deceleration times of the E-wave (E AT and E DT , respectively) were also derived from the transmitral Doppler waveform.
Pulmonary Venous Doppler Echocardiography
The pulmonary vein (PV) was visualized by orientating the transducer in the parasternal long-axis view with the left atrium, left ventricle, and aorta in the field ( Figure 1 A, B). The transducer was then moved laterally and rotated clockwise by 10 to 20 degrees, and the beam was then oriented slightly inferiorly and anteriorly to visualize the PV ( Figure 1 C, D). The single PV connects to the LA close to the LV atrioventricular groove ( Figure 1 D). The PV Doppler waveform was obtained by placing the Doppler sample volume approximately 1 mm away from the LA-PV junction with an angle correction of less than 15 degrees. To locate the PV, we would first identify the aorta in the parasternal long-axis view and then tilt the transducer posteriorly until the aorta disappeared and the PV came into the field. In mice, the coronary sinus is in close anatomic proximity to the PV, and so the coronary sinus could be mistaken for the PV while trying to obtain Doppler recordings. This type of confusion may be avoided by analyzing the Doppler spectra, where blood flow in the coronary sinus is affected to a much larger degree by respirations than in the PV. Pulmonary venous flow velocity and the velocity-time integral (VTI) of all waves were then measured. All Doppler parameters are reported as the average value obtained from three consecutive cardiac cycles.

Invasive Hemodynamics
Protocols for invasive hemodynamic recordings have been described. Briefly, anesthesia was achieved using 1% to 2% isoflurane with 100% oxygen by ventilation, and core body temperature was maintained at 36°C to 37°C using a heating pad and rectal temperature probe. Intracardiac pressures were recorded with a 1.4F microtip catheter (SPR-839; Millar Instruments, Houston, TX) and zeroed in a saline bath before obtaining measurements from each animal. The catheter was inserted via the right carotid artery into the left ventricle to record intracardiac pressures. Signals were digitized at 2 kHz using a PowerLab/16 SP A/D converter (ADInstruments Ltd., Mountain View, CA) and stored on the hard drive of a Core Duo-based PC computer for off-line analysis. Data were analyzed using the PVAN3.2 analysis suite (Millar Instruments) and normalized to the mean arterial pressure to account for load-dependency. The LV relaxation time constant (tau) was calculated by two different methods: 1) the Weiss method (tau [w]): regression of log (pressure) versus time and 2) the Glantz method (tau [g]): regression of dp/dt versus pressure.
Intraobserver and Interobserver Variability
To evaluate intraobserver and interobserver variability of Doppler echocardiographic measurements, one same observer and two independent observers remeasured 12 sets of values for E AT , pulmonary venous first diastolic wave velocity and IVRT in 12 animals using the same cardiac cycles.
Statistical Analysis
All values are reported as the mean ± 1 standard deviation. Differences between groups were analyzed using the 2-tailed Student’s t test. Linear regression analysis was used to determine correlations between echocardiographic and invasive relaxation parameters. Agreement between two measurements was determined according to the method of Bland and Altman. A P value < .05 was considered significant for all analysis (Prism 5, GraphPad Inc., San Diego, CA). Data were not corrected for multiple comparisons to reduce type I error due to the risk of introducing type II error.
Results
We previously studied HopX Tg mice and found most of these animals develop LV hypertrophy with diastolic dysfunction by the age of 14 to 18 weeks. Therefore, we used these mice as a model of LV hypertrophy and diastolic dysfunction for these studies. We confirmed that all HopX Tg mice used in this investigation had LV hypertrophy, as denoted by a significant increase in diastolic relative wall thickness and LV mass-to-body weight ratio ( Table 1 ).
Relative Wall Thickness | LV Mass Index (mg/g) | Heart Weight (mg) | Heart Weight/Tibia Length (mg/mm) | |
---|---|---|---|---|
WT | 0.32 ± 0.03 | 2.62 ± 0.18 | 125.12 ± 14.50 | 7.23 ± 0.68 |
HopX Tg | 0.71 ± 0.12 | 3.92 ± 0.64 | 256.61 ± 33.83 | 14.35 ± 1.99 |
P value | <.0001 | <.0001 | <.0001 | <.0001 |
Evaluation of Left Ventricular Relaxation in HopX Tg Mice Using Invasive Hemodynamics
Although HopX Tg mice clearly develop LV hypertrophy, we next sought to determine the extent to which LV diastolic function was affected in these animals. Invasive hemodynamic recordings revealed HopX Tg mice had reduced LV relaxation compared with WT control mice, where tau (g) and LVEDP were significantly higher and -dP/dt min was much lower ( Figure 2 A, B; Table 2 ). These data confirm that HopX Tg mice with LV hypertrophy have impairments in LV relaxation. Furthermore, measurements of the LV peak pressure and +dp/dt max revealed that LV systolic function is preserved in HopX Tg mice ( Table 2 ).

Wild Type (n = 10) | HopX Tg (n = 10) | P Value | |
---|---|---|---|
Two-dimensional echocardiographic indices | |||
IVST (mm) | 0.64 ± 0.06 | 1.25 ± 0.16 | <.0001 |
LVPWT (mm) | 0.62 ± 0.04 | 1.19 ± 0.13 | <.0001 |
LVIDd (mm) | 3.90 ± 0.28 | 3.45 ± 0.28 | .003 |
LVIDs (mm) | 2.71 ± 0.38 | 1.64 ± 0.41 | <.0001 |
LVEF (%) | 60.5 ± 6.1 | 83.8 ± 7.1 | <.0001 |
LVFS (%) | 32.0 ± 4.2 | 53.8 ± 8.2 | <.0001 |
LVEDV (μL) | 70.4 ± 6.8 | 52.8 ± 10.5 | <.0001 |
LVESV (μL) | 29.8 ± 6.9 | 8.9 ± 5.6 | <.0001 |
SV (μL) | 40.6 ± 4.7 | 43.8 ± 7.1 | .242 |
CO (mL/min) | 19.7 ± 3.6 | 20.2 ± 2.8 | .730 |
LA (mm 2 ) | 3.7 ± 0.9 | 6.3 ± 0.9 | <.0001 |
Transmitral Doppler indices | |||
E flow velocity (cm/s) | 81.2 ± 7.2 | 54.75 ± 10.62 | <.0001 |
A flow velocity (cm/s) | 57.2 ± 7.1 | 42.7 ± 8.8 | .006 |
E/A ratio | 1.4 ± 0.1 | 1.19 ± 0.2 | .012 |
IVRT (ms) | 12.3 ± 2.5 | 15.6 ± 5.6 | .082 |
E AT (ms) | 11.9 ± 0.7 | 17.7 ± 1.1 | <.0001 |
E DT (ms) | 27.5 ± 9.6 | 31.0 ± 7.8 | .072 |
IVCT (ms) | 10.7 ± 3.4 | 9.7 ± 2.8 | .229 |
ET (ms) | 41.6 ± 4.4 | 45.5 ± 8.2 | .456 |
Vel LVOT (cm/s) | 28.7 ± 9.5 | 152.3 ± 46.8 | <.0001 |
Heart rate (bpm) | 484 ± 68 | 465 ± 44 | .445 |
Pulmonary venous Doppler indices | |||
PV a -wave velocity (cm/s) | −4.7 ± 1.6 | −6.0 ± 3.6 | .306 |
PV S-wave velocity (cm/s) | 22.8 ± 5.4 | 20.0 ± 3.9 | .197 |
PV D1-wave velocity (cm/s) | 48.5 ± 6.5 | 43.9 ± 12.6 | .279 |
PV D2-wave velocity (cm/s) | 34.8 ± 9.0 | 28.0 ± 9.5 | .202 |
PV S/D1 | 0.47 ± 0.10 | 0.48 ± 0.12 | .934 |
PV S/D2 | 0.69 ± 0.24 | 0.78 ± 0.32 | .405 |
PV VTI a (cm) | 0.03 ± 0.01 | 0.04 ± 0.01 | .127 |
PV VTIS (cm) | 0.62 ± 0.16 | 0.57 ± 0.19 | .523 |
PV VTID (cm) | 1.90 ± 0.37 | 1.56 ± 0.24 | .028 |
Hemodynamic indices | |||
-dP/dt min (mm Hg/sec) | −7950 ± 1490 | −6240 ± 1140 | .010 |
(-dP/dt min )/LVSP (s −1 ) | −74.4 ± 10.9 | −57.0 ± 14.9 | .007 |
Tau g (ms) | 10.0 ± 1.0 | 15.8 ± 4.0 | <.001 |
Tau w (ms) | 7.5 ± 1.1 | 9.3 ± 2.9 | .099 |
LVEDP (mm Hg) | 8.1 ± 4.0 | 13.6 ± 4.6 | .011 |
Peak LV pressure (mm Hg) | 112.3 ± 9.5 | 126.5 ± 9.3 | .335 |
+dP/dt max (mm Hg/sec) | 11,080 ± 2800 | 10,890 ± 1880 | .861 |
Evaluation of Left Ventricular Relaxation in HopX Tg Mice by Doppler Echocardiography
Transmitral Doppler echocardiography provided evidence for reduced LV relaxation in HopX Tg mice, as revealed by a decrease in the E-wave flow velocity and E/A ratio, and an increase in the acceleration time of the transmitral early diastolic peak flow velocity (E AT ) ( Figure 2 C, D; Table 2 ). On the other hand, the deceleration time of the transmitral early diastolic peak flow velocity (E DT ), which is a function of LV compliance and typically affected in later stages of diastole dysfunction, was not different between WT and HopX Tg mice ( Table 2 ).
Doppler interrogation of the PV revealed the waveform was composed of a small S-wave, two forward D-waves (D1 and D2), and a small, reversed a -wave in all WT mice examined. Although we could obtain pulmonary venous flows from every mouse we studied, approximately 50% of the HopX Tg mice examined did not have a D2 wave. Lack of a D2 wave in half of the HopX Tg mice probably accounts for the slight, yet significant, decrease in the pulmonary VTI during diastole (PV VTId) in HopX Tg mice compared with control littermates ( Figure 2 E, F; Table 2 ).
Comparison of Left Ventricular Relaxation by Echocardiography versus Invasive Hemodynamics in HopX Tg Mice
To validate the Doppler echocardiographic indices for assessing diastolic function in mice, we compared several noninvasive measurements of LV filling and relaxation with invasively measured parameters of LV relaxation. In this model, we found that the E AT by Doppler echocardiography and relative wall thickness and LA area by non-Doppler echocardiography correlated well with -dp/dt min , tau (g), and LVEDP ( Figure 3 ; Table 3 ). In contrast, the IVRT did not correlate well with -dp/dt min , and the E/A ratio did not show good correlation with any of the invasive parameters ( Table 3 ).
LVEDP (mm Hg) | (-dP/dt min )/LVSP (s-1) | Tau (g) (ms) | ||||
---|---|---|---|---|---|---|
Index | r | P Value | r | P Value | r | P Value |
E/A ratio | −0.05 | .822 | 0.05 | .819 | −0.28 | .239 |
E AT (ms) | 0.60 | .005 | 0.60 | .005 | 0.74 | <.001 |
E AT /R-R interval | 0.56 | .010 | 0.55 | .012 | 0.78 | <.0001 |
E flow velocity (cm/s) | −0.25 | .295 | −0.23 | .335 | −0.50 | .026 |
IVRT (ms) | 0.37 | .106 | 0.25 | .297 | 0.28 | .240 |
IVRT/R-R interval | 0.47 | .035 | 0.25 | .295 | 0.61 | .004 |
PV D1-wave velocity (cm/s) | −0.11 | .642 | 0.03 | .899 | −0.07 | .767 |
PV VTID (cm) | −0.14 | .557 | −0.28 | .237 | −0.20 | .403 |
IVST (mm) | 0.66 | .002 | 0.78 | <.0001 | 0.79 | <.0001 |
LVPWT (mm) | 0.62 | .004 | 0.66 | .001 | 0.59 | .045 |
RWT | 0.68 | .001 | 0.78 | <.0001 | 0.82 | <.0001 |
LA | 0.31 | .186 | 0.53 | .016 | 0.52 | .018 |
LVEDV (μL) | −0.55 | .012 | −0.67 | .001 | −0.79 | <.0001 |
LVESV (μL) | −0.56 | .009 | −0.68 | <.001 | −0.79 | <.0001 |
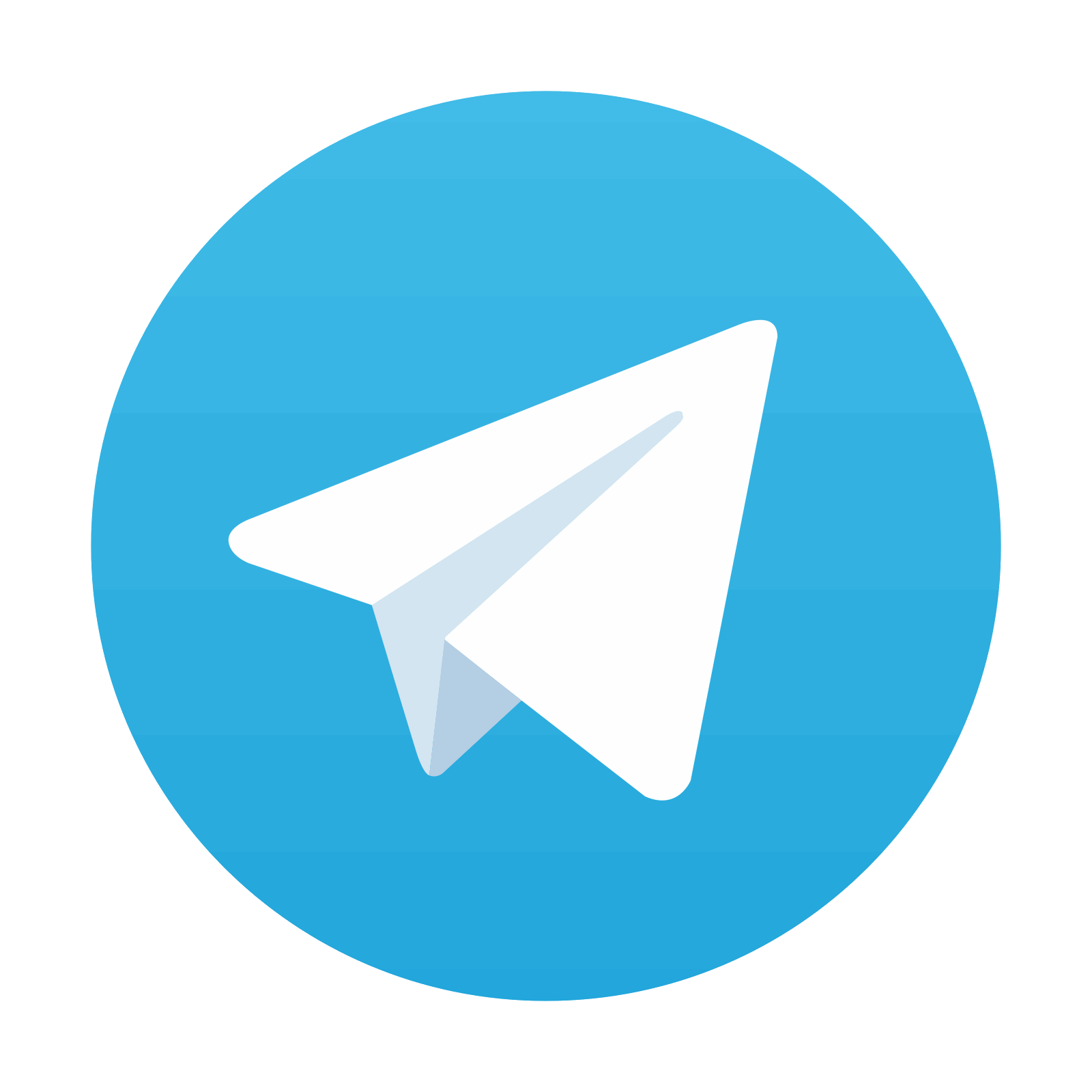
Stay updated, free articles. Join our Telegram channel

Full access? Get Clinical Tree
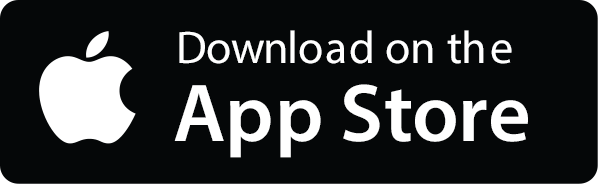
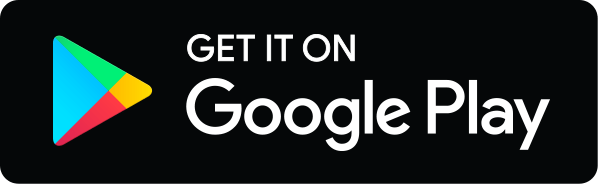
