Background
The mitral valve has a complex three-dimensional (3D) morphology that is incompletely described by two-dimensional echocardiography (echo). Three-dimensional echo provides a more robust tool to analyze the mitral valve. The shape of the mitral annulus and leaflets, and differences with age, have not been described by 3D echo in normal children. Our objective was to characterize and quantify the 3D mitral valve morphology in children with normal transthoracic echocardiograms over a broad spectrum of age and to identify differences in valve shape with age.
Methods
Three-dimensional midsystolic mitral valve models were constructed in 100 children and young adults with normal echocardiograms using 3D transthoracic images. Annular and leaflet metrics were quantified and regression equations were prepared. Interuser and intrauser variability was measured.
Results
Two hundred fifty patients, from neonate to young adult, were retrospectively reviewed to obtain 100 evaluable patients (40% evaluable). The annular height to commissural width ratio of the mitral valve (“saddle shape”) was preserved across age (median 24.3, IQR 21.8–28.1). Three-dimensional mitral valve area, length, and volume parameters were linearly related to body surface area ( P < .001). The ratio of anterior to posterior leaflet length and posterior leaflet angle increased with body surface area ( P = .0004 and .002, respectively) suggesting posterior movement of the coaptation line. Two-dimensional lateral annular diameter underestimated 3D lateral annular metrics ( P < .001, mean difference 20–22%) but was highly correlated ( R > 0.87, P < .001). Interuser and intrauser variability were acceptable.
Conclusions
Assessment of 3D mitral valve morphology in children is possible in a modern clinical pediatric echocardiography laboratory using transthoracic images, although further optimization of imaging is needed. The saddle shape of the mitral annulus was preserved across age and size. Most mitral valve parameters increased linearly with patient size. Further investigation is warranted to explore changes in valve morphology in the pediatric population in health and with disease.
Highlights
- –
Assessment of three-dimensional mitral valve morphology in children is possible in a modern clinical pediatric echocardiography laboratory using transthoracic images, although further optimization of imaging is needed.
- –
The saddle shape of the mitral annulus was preserved across age and size.
- –
Most mitral valve parameters increased linearly with patient size, however, the ratio of anterior to posterior leaflet length and posterior leaflet angle increased with BSA suggesting posterior movement of the coaptation line.
- –
Two-dimensional lateral annular diameter systematically underestimated three-dimensional lateral annular metrics, but was highly correlated.
Attention ASE Members:
The ASE has gone green! Visit www.aseuniversity.org to earn free continuing medical education credit through an online activity related to this article. Certificates are available for immediate access upon successful completion of the activity. Nonmembers will need to join the ASE to access this great member benefit!
Methods
Subjects
An institutional database was used to retrospectively identify patients with structurally normal hearts and existing 3D left ventricular (LV) volume datasets (in January 2014 our laboratory began acquiring these images as part of routine clinical care). Functional or structural heart disease had been suspected in these children but had been judged to be absent by their treating cardiologists. Specific inclusion criteria were as follows: (1) referral for clinical echocardiogram, (2) no history of congenital or acquired heart disease, (3) no more than trivial valvular regurgitation, (4) no structural defects (patent foramen ovale and trivial branch pulmonary artery stenosis permitted in infants), (5) LV end-diastolic volume Z score > −2.5 and < +2.5; and (6) normal LV systolic function (ejection fraction > 55%) on a complete 2D and Doppler echocardiogram. Exclusion criteria included significant stitch artifact, lack of inclusion of the entire mitral annulus in the acquisition, or inability to delineate the mitral valve sufficiently to reliably model the valve. Three-dimensional studies in 250 subjects were reviewed to obtain the 100 patients used in the study. The study was approved by the Committee on Clinical Investigation at Boston Children’s Hospital.
Transthoracic Image Acquisition and 2D Echo Data
The 3D images were acquired using full volume or 3D zoom mode, using 4-beat breath-held electrocardiiogram-gated acquisitions when possible (breath holding was not feasible in infants and young children). Transthoracic X7 or X5 probes were used with the Philips IE33 and EPIQ ultrasound systems (Philips Medical, Andover, MA). The 2D volumes and ejection fraction were calculated using the 5/6 area length formula. The 2D mitral annular lateral dimension was recorded from the clinical reports, where available.
3D Mitral Valve Modeling and Analysis
Mitral valve 3D models were constructed using the Tomtec 4D MVA analysis package (version 2.3), running within Image Arena 4.6 software (TomTec Imaging Systems, Unterschleisshem, Germany). Briefly, the mitral valve analysis began with the identification of anatomic landmarks and selection of the early systolic frame (first frame with valve closed) and the end-systolic frames (last frame before the mitral valve starts to open). The single midsystolic frame midway between these two frames was chosen for static modeling and analysis of the valve. This phase allowed direct comparison with multiple adult studies. Analysis of the leaflets was based on optical flow and pattern recognition. Annular dimensions and characteristics, and leaflet dimensions and characteristics, were automatically generated with manual user correction when needed. Annular dimensions are presented in Figure 2 (height, anterior-posterior diameter, anterolateral-posteromedial diameter, commissural diameter, circumference, and annular nonplanar angle); also recorded were 2D area, 3D area, and sphericity. Mitral valve sphericity was defined as the mitral annular anterior-posterior diameter divided by the anterolateral-posteromedial diameter. The AHCWR was defined as the annular height divided by the commissural width at the annulus, expressed as a percentage. Leaflet characteristics included anterior leaflet area, posterior leaflet area, anterior leaflet length, posterior leaflet length, coaptation length, posterior leaflet bending angle, tenting height, tenting area, and tenting volume ( Figure 3 ). Definitions of all quantities are shown in Table 1 .


Variable | Description |
---|---|
Anterior-posterior diameter (cm) | Distance from anterior mitral annulus to posterior annulus |
Anterolateral-posteromedial diameter (cm) | Distance from anterolateral annulus to posteromedial annulus |
Commissural width (cm) | Width of the mitral annulus at the level of the commissures |
Annulus circumference (cm) | Distance around the annular circumference |
Sphericity | Anterior-posterior diameter divided by the anterolateral-posteromedial diameter |
2D annular Area (cm 2 ) | 3D annular area projected onto the 2D best-fit plane of the annulus |
3D annular area (cm 2 ) | Minimum area “soap bubble” manifold surface enclosed by the 3D mitral annulus |
Annular height (cm) | Height of mitral annulus (lowest point to highest point) |
Nonplanar angle (degrees) | Angle between anterior and posterior leaflets |
Annular height to commissural width ratio | Annular height divided by annular commissural width (AHCWR) |
Tenting volume (cm 3 ) | Volume encompassed by surface defined by annulus and the leaflets |
Tenting area (cm 2 ) | Area between leaflets and a line from anterior to posterior annulus at midpoint of valve |
Tenting height (mm) | Distance from leaflet coaptation to anterior-posterior diameter line |
Anterior leaflet area (cm 2 ) | 3D area of the anterior leaflet |
Anterior length (cm) | Length of the anterior leaflet from annulus to coaptation point |
Posterior leaflet area (cm 2 ) | 3D area of the posterior leaflet |
Posterior length (cm) | Length of the posterior leaflet from annulus to coaptation point |
Posterior leaflet angle (degrees) | Angle between anterior-posterior diameter line and posterior leaflet length line |
Leaflet closure line length (3D) (cm) | Length of coaptation line between anterior and posterior leaflets |
Statistics
The data are presented as median and interquartile range (IQR) unless otherwise stated. Relationships between age, body surface area (BSA), and mitral valve parameters were modeled using linear regression. Interobserver and intraobserver reproducibility for 3D mitral measurements was assessed on a random subset of 20 subjects. For intraobserver assessment the same observer (M.J.) remeasured all the parameters at least 1 month apart. For interobserver assessment, a second observer (S.G.) performed all measurements without knowledge of the results of the first observer. Intraobserver reproducibility was quantified using the intraclass correlation coefficient (ICC), which was generated using the two-way model for absolute agreement for average measures. Interobserver agreement was assessed using Lin’s concordance correlation coefficient. Mean differences were calculated for both intraobserver and interobserver measurements. In addition, mean relative difference (%) was calculated as the mean of the ratio of absolute difference and average of each pair of observations expressed as a percentage for intraobserver and interobserver measurements. Statistical analysis was performed using SAS 9.4 (SAS Institute Inc., Cary, NC), IBM SPSS version 21.0 (IBM corporation, Armonk, NY), and STATA version 13.1 (StataCorp, College Station, TX).
Methods
Subjects
An institutional database was used to retrospectively identify patients with structurally normal hearts and existing 3D left ventricular (LV) volume datasets (in January 2014 our laboratory began acquiring these images as part of routine clinical care). Functional or structural heart disease had been suspected in these children but had been judged to be absent by their treating cardiologists. Specific inclusion criteria were as follows: (1) referral for clinical echocardiogram, (2) no history of congenital or acquired heart disease, (3) no more than trivial valvular regurgitation, (4) no structural defects (patent foramen ovale and trivial branch pulmonary artery stenosis permitted in infants), (5) LV end-diastolic volume Z score > −2.5 and < +2.5; and (6) normal LV systolic function (ejection fraction > 55%) on a complete 2D and Doppler echocardiogram. Exclusion criteria included significant stitch artifact, lack of inclusion of the entire mitral annulus in the acquisition, or inability to delineate the mitral valve sufficiently to reliably model the valve. Three-dimensional studies in 250 subjects were reviewed to obtain the 100 patients used in the study. The study was approved by the Committee on Clinical Investigation at Boston Children’s Hospital.
Transthoracic Image Acquisition and 2D Echo Data
The 3D images were acquired using full volume or 3D zoom mode, using 4-beat breath-held electrocardiiogram-gated acquisitions when possible (breath holding was not feasible in infants and young children). Transthoracic X7 or X5 probes were used with the Philips IE33 and EPIQ ultrasound systems (Philips Medical, Andover, MA). The 2D volumes and ejection fraction were calculated using the 5/6 area length formula. The 2D mitral annular lateral dimension was recorded from the clinical reports, where available.
3D Mitral Valve Modeling and Analysis
Mitral valve 3D models were constructed using the Tomtec 4D MVA analysis package (version 2.3), running within Image Arena 4.6 software (TomTec Imaging Systems, Unterschleisshem, Germany). Briefly, the mitral valve analysis began with the identification of anatomic landmarks and selection of the early systolic frame (first frame with valve closed) and the end-systolic frames (last frame before the mitral valve starts to open). The single midsystolic frame midway between these two frames was chosen for static modeling and analysis of the valve. This phase allowed direct comparison with multiple adult studies. Analysis of the leaflets was based on optical flow and pattern recognition. Annular dimensions and characteristics, and leaflet dimensions and characteristics, were automatically generated with manual user correction when needed. Annular dimensions are presented in Figure 2 (height, anterior-posterior diameter, anterolateral-posteromedial diameter, commissural diameter, circumference, and annular nonplanar angle); also recorded were 2D area, 3D area, and sphericity. Mitral valve sphericity was defined as the mitral annular anterior-posterior diameter divided by the anterolateral-posteromedial diameter. The AHCWR was defined as the annular height divided by the commissural width at the annulus, expressed as a percentage. Leaflet characteristics included anterior leaflet area, posterior leaflet area, anterior leaflet length, posterior leaflet length, coaptation length, posterior leaflet bending angle, tenting height, tenting area, and tenting volume ( Figure 3 ). Definitions of all quantities are shown in Table 1 .
Variable | Description |
---|---|
Anterior-posterior diameter (cm) | Distance from anterior mitral annulus to posterior annulus |
Anterolateral-posteromedial diameter (cm) | Distance from anterolateral annulus to posteromedial annulus |
Commissural width (cm) | Width of the mitral annulus at the level of the commissures |
Annulus circumference (cm) | Distance around the annular circumference |
Sphericity | Anterior-posterior diameter divided by the anterolateral-posteromedial diameter |
2D annular Area (cm 2 ) | 3D annular area projected onto the 2D best-fit plane of the annulus |
3D annular area (cm 2 ) | Minimum area “soap bubble” manifold surface enclosed by the 3D mitral annulus |
Annular height (cm) | Height of mitral annulus (lowest point to highest point) |
Nonplanar angle (degrees) | Angle between anterior and posterior leaflets |
Annular height to commissural width ratio | Annular height divided by annular commissural width (AHCWR) |
Tenting volume (cm 3 ) | Volume encompassed by surface defined by annulus and the leaflets |
Tenting area (cm 2 ) | Area between leaflets and a line from anterior to posterior annulus at midpoint of valve |
Tenting height (mm) | Distance from leaflet coaptation to anterior-posterior diameter line |
Anterior leaflet area (cm 2 ) | 3D area of the anterior leaflet |
Anterior length (cm) | Length of the anterior leaflet from annulus to coaptation point |
Posterior leaflet area (cm 2 ) | 3D area of the posterior leaflet |
Posterior length (cm) | Length of the posterior leaflet from annulus to coaptation point |
Posterior leaflet angle (degrees) | Angle between anterior-posterior diameter line and posterior leaflet length line |
Leaflet closure line length (3D) (cm) | Length of coaptation line between anterior and posterior leaflets |
Statistics
The data are presented as median and interquartile range (IQR) unless otherwise stated. Relationships between age, body surface area (BSA), and mitral valve parameters were modeled using linear regression. Interobserver and intraobserver reproducibility for 3D mitral measurements was assessed on a random subset of 20 subjects. For intraobserver assessment the same observer (M.J.) remeasured all the parameters at least 1 month apart. For interobserver assessment, a second observer (S.G.) performed all measurements without knowledge of the results of the first observer. Intraobserver reproducibility was quantified using the intraclass correlation coefficient (ICC), which was generated using the two-way model for absolute agreement for average measures. Interobserver agreement was assessed using Lin’s concordance correlation coefficient. Mean differences were calculated for both intraobserver and interobserver measurements. In addition, mean relative difference (%) was calculated as the mean of the ratio of absolute difference and average of each pair of observations expressed as a percentage for intraobserver and interobserver measurements. Statistical analysis was performed using SAS 9.4 (SAS Institute Inc., Cary, NC), IBM SPSS version 21.0 (IBM corporation, Armonk, NY), and STATA version 13.1 (StataCorp, College Station, TX).
Results
Out of 250 eligible 3D acquisitions, 100 (40%) were judged to have adequate images of the mitral valve to allow analysis upon retrospective review. The median frame rate of the analyzed images was 31 Hz (IQR 26–35 Hz). The most common indications for these echocardiograms were murmur ( n = 22), chest pain ( n = 19), family history of congenital heart disease ( n = 15), rule out connective tissue disease ( n = 14), abnormal electrocardiogram or palpitations ( n = 14), history of syncope or presyncope ( n = 9) or other indication ( n = 7). The median systolic time (duration of mitral valve being in the closed position) was 342 msec (IQR 305–370 msec).
Table 2 shows patient demographic and echocardiographic characteristics of the analyzed patient cohort. The study population ranged in age from 1 day to 22 years (median 9.4 y, IQR 5.1–14.6 y); 44 of 100 patients were female (44%). The patients’ weights ranged from 2.2 to 92.3 kg; BSA ranged from 0.17 to 2.18 m 2 . No patients were obese (BMI > 30 kg/m 2 ). All patients had a normal heart rate, blood pressure, and 2D LV EF recorded at the time of the echo study used for mitral modeling. As expected, the 2D mitral annular lateral dimension measurement increased with progressive age categories.
Variable | <1 y ( n = 11) | 1–5 y ( n = 12) | >5–9 y ( n = 22) | >9–13 y ( n = 26) | >13 y ( n = 29) |
---|---|---|---|---|---|
Age (y) | 0.1 (0.0–0.20) | 3.3 (3.0–4.7) | 7.0 (6.2–7.7) | 11.2 (9.8–11.6) | 16.0 (15.0–15.9) |
Female ( n ,%) | 4 (36) | 8 (67) | 11 (50) | 7 (27) | 14 (48) |
Height (cm) | 52 (49–58) | 100 (27–110) | 122 (118–125) | 143 (141–150) | 168 (164–174) |
Weight (kg) | 3.6 (2.9–5.3) | 15.7 (15.0–19.6) | 23.6 (21.8–25.9) | 37.1 (31.8–43.1) | 60.0 (55.7–64.5) |
BSA (m 2 ) | 0.23 (0.20–0.30) | 0.67 (0.64–0.80) | 0.91 (0.84–0.94) | 1.22 (1.11–1.34) | 1.68 (1.60–1.74) |
BMI (kg/m 2 ) | 12.9 (11.2–14.0) | 16.2 (15.4–16.6) | 15.9 (14.4–17.3) | 17.0 (16.4–20.0) | 21.1 (19.8–23.5) |
Heart rate (bpm) | 135 (124–143) | 94 (88–102) | 78 (76–84) | 74 (64–78) | 64 (58–72) |
SBP (mm Hg) | 83 (79–89) | 92 (89–99) | 102 (98–107) | 109 (98–117) | 116 (108–121) |
DBP (mm Hg) | 41 (34–58) | 57 (51–59) | 55 (51–64) | 59 (52–63) | 60 (52–65) |
MBP (mm Hg) | 54 (48–62) | 65 (60–67) | 66 (63–73) | 70 (65–74) | 74 (65–78) |
EF 2D (%) | 63 (61–66) | 62 (60–66) | 63 (61–66) | 64 (63–67) | 62 (60–64) |
LV EDV (mL) | 10 (6–15) | 46 (40–58) | 65 (57–73) | 92 (78–101) | 151 (131–166) |
LV ESV (mL) | 4 (2–6) | 18 (15–21) | 24 (22–25) | 34 (29–36) | 58 (46–67) |
2D mitral annulus lateral dimension (cm) | 1.1 (1.0–1.2) | 1.8 (1.8–2.1) | 2.1 (2.1–2.3) | 2.5 (2.2–2.6) | 2.8 (2.5–2.9) |
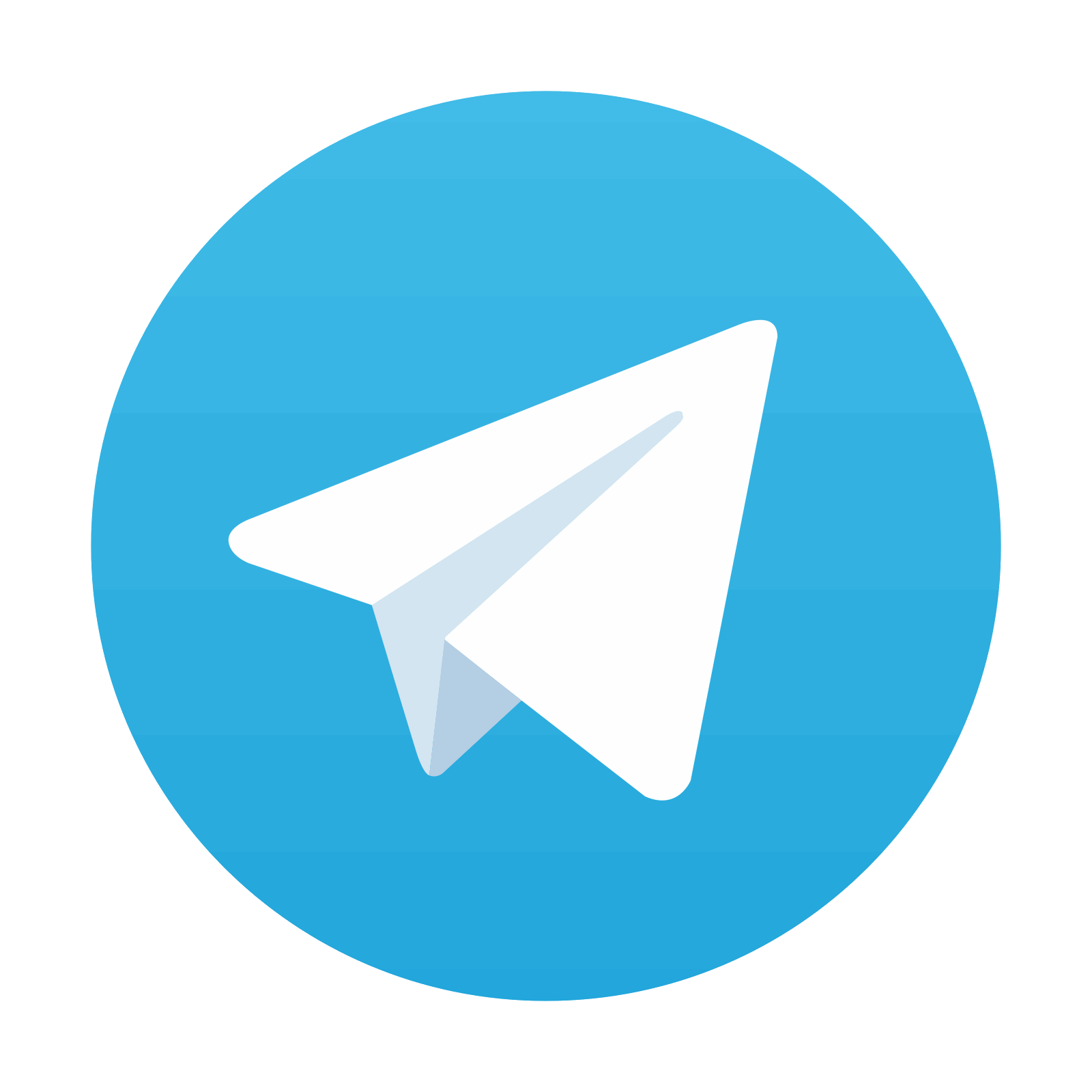
Stay updated, free articles. Join our Telegram channel

Full access? Get Clinical Tree
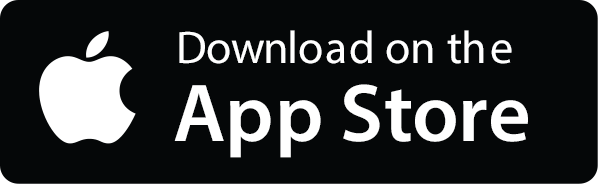
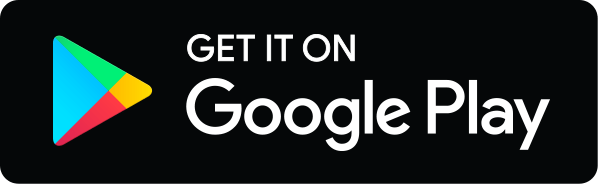
