Mass median aerodynamic diameter (MMAD) is a particle size (in microns, μm) that divides the mass in such a way that half of the particles of that aerosol have a larger diameter and the other half a diameter smaller than the diameter of the MMAD. The geometric standard deviation (GSD) is the ratio between the MMAD and the diameter of the particles at a median standard deviation (or 84th percentile). When the GSD is less than 1.22, an aerosol is considered monodisperse; if this value is higher, it is considered polydisperse. The greater the MMAD, the greater the average size of the particles; the greater the GSD, the wider the dispersion of particle sizes in that aerosol. Most aerosols for medical use are polydispersed.
The mass median aerodynamic diameter standardizes the size of the particle to the behavior of a spherical water drop, which by definition has a density of 1. Since medical aerosols never have a uniform diameter, shape, or density, the mass median aerodynamic diameter is determined by inertial impact, laser diffraction or digital image processing methods, among others.
The primary method for defining the diameter of the particles and their distribution is the use of an inertial cascade impactor. For example, the Andersen Cascade Impactor has 8 different stages (collectors), capturing particles from 0.43 to 9.0 μm when operating at a flow rate of 28 L/min. The Next Generation impactor has 7 stages and captures particles from 0.24 to 11.7 μm at 30 L/min. The aerodynamic particle size distribution is determined by the quantities of drug deposited in each collector. The total mass of drug collected is reported as the delivered dose. When the partial mass of drug collected in the smaller diameter collectors is measured, the respirable or fine particle dose is obtained. The limit of this can be set arbitrarily in particles smaller than 6.4, 5.0 or 3.0 μm. Frequently, the fine particle dose includes those with diameters between 1 and 5 μm.
When an aerosol particle increases in size, so does its mass. By doubling the radius of a sphere, the volume grows approximately 8 times (V = 4 × k × r3). As the volume increases, the mass increases proportionally. When the mass of an aerosol particle increases, gravity will have a greater influence on it, and it will remain suspended for a shorter time.
Hygroscopicity : It is the intrinsic tendency of a material to absorb moisture from its environment. It is influenced by the material crystallinity and the morphology of the particles. Hygroscopic drugs pose a greater risk of physical and chemical instability. For dry powder drugs, moisture gain or loss due to changes in relative humidity can lead to dissolution and subsequent recrystallization, hindering deagglomeration and therefore affecting drug delivery from the device.
Moisture can be captured within the device prior to delivery (e.g., dry powder inhaler or DPI) or after the dose goes into the airway, where the particle is in a moist and warm environment (37 °C and 99% relative humidity in the carina). The susceptible particle will experience hygroscopic growth, which increases its mass and dimensions. When inhaled, this particle tends to be deposited in the airway in a more proximal location than that of a non-hygroscopic aerosol particle. This is because hygroscopic ones tend to coalesce, creating particles of greater mass and volume that tend to be suspended for less time.
Tonicity : It refers to solute concentration in a solution regarding the concentration in body fluids. When creating an aerosol with a hypertonic solution (>0.9% NaCl) its particles will be hygroscopic, increasing its size. An isotonic solution (0.9% NaCl) will create aerosol particles with neutral affinity for water that tend to remain stable. A hypotonic solution (<0.9% NaCl) will create aerosol particles with a tendency to release water, therefore decreasing in size and mass.
Electric charge: Because of the methods used to create an aerosol, the particles have an electric charge. This phenomenon seems to have little physiological effect on the patient, but it influences the lung deposition since there are interactions between the particles and the walls of the devices used.
Aerosol Deposition Mechanisms
The particles are deposited in the airway when they are definitively removed from the laminar flow that has been created by the inspiratory movement. The knowledge of the processes and factors that influence the deposition of particles in different regions of the airway makes it easier for the clinician to choose the best therapy for their patient. There are five mechanisms by which a particle can be deposited in the airway: inertial impaction, sedimentation, diffusion, interception, and electrostatic precipitation. The first two mechanisms are directly related to the size of the particle, while the third is inversely related to it. Interception depends on the shape of the particle and occurs when two of them meet, which is more common for elongated particles. Electrostatic precipitation is related to the electric charge, when two particles of opposite charges meet. The most relevant mechanisms are the first three.
Occurs when aerosol particles have sufficient momentum (mass × velocity) to maintain their trajectory despite a change in the air flow direction, thus colliding with the walls of the airway. The probability of inertial deposition increases when the particle is able to travel long distances, which depends on its mobility, mass, and speed. This is expressed in the following equation, where S corresponds to distance, B to mobility (velocity × unit force), m to mass, and v to particle velocity.
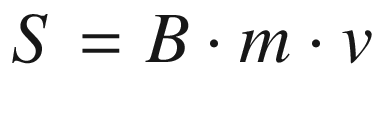
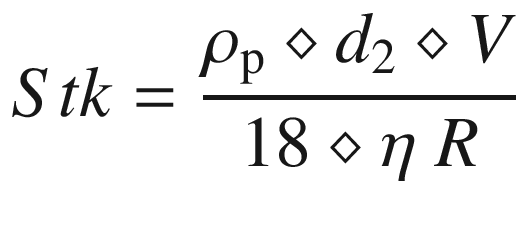
Large particles traveling at high speed are more likely to impact the airway proximal regions. This mechanism is responsible for the filter role carried out by the upper airway on the inspired air, since particles larger than 10 μm tend to be deposited. Impaction increases dramatically with high inspiratory flows; and it is the predominant mechanism of deposition in the first seven generations of the airway.
It is a time-dependent mechanism, in which the particles settle by the action of gravity. It is related to particle size and the flow velocity of the inhaled gas. This is the primary deposition mechanism for particles with a MMAD <2 μm, although it can affect larger particles in low flow conditions. This mechanism optimizes lung deposition. Gravitational sedimentation increases when the patient performs an apnea technique; and predominates as a deposition mechanism after the eighth generation of the airway, given the flow decrease and the smaller diameter.
Occurs in particles <0.5 μm, less influenced by gravity, in which Brownian motion plays a more important role and favors the coalescence of the particles. This mechanism is influenced by electrical charge. A significant percentage of these particles are exhaled.
General Factors That Affect Airway Aerosol Deposition
Factors of stability and airway deposition of an aerosol
Physical nature of the particle: size and mass, hygroscopicity, tonicity, electric charge |
Aerosol: Particle size, gravity, inertia, gas temperature, and gas humidity |
Patient: Age, respiratory pattern, airway anatomy, respiratory mechanics |
Equipment: Aerosol generator, type of drug, propellant, holding chamber or spacer device |
Aerosol-Dependent Factors
If the size and mass of the particle increase, the gravitational forces exerted on it have a greater influence and tend to remove it from the suspension. Ideally, most particles should have a mass such that the influence of gravity promotes deposition by sedimentation in the desired place, because when the particles are very small they lean to remain suspended, achieving minimum lung deposition and resulting in that many of them are exhaled. The optimal size for a proper deposition in the respiratory bronchioles ranges from 1 to 5 μm (breathable dose).
Inertia: It is related to the size and mass of the particle. According to Newton, kinetic energy is equal to 50% of the product of the mass times speed squared. When a larger mass particle is set in motion it will have more inertia than a smaller mass particle, even when both move at the same speed. When a particle starts moving, it tends to stay that way unless external forces act on it. Owing to its greater momentum, a particle of large mass tends to travel in a straight line even though there is a change in the direction of the gas flow in which it is suspended. Large particles (>10 μm) tend to be removed in the upper airway, while smaller particles can reach more distal regions.
Temperature and humidity: There is a close relationship between these two factors related to the percentage of aerosol particles that remain in suspension. When the temperature of gas increases and humidity remains stable, particles lose water and decrease in size. By increasing gas humidity and keeping the temperature stable, particles collect water, depending on their hygroscopic capacity, and tend to coalesce and increase their size. For very small particles that are deposited by diffusion, an increase in temperature favors this mechanism due to greater Brownian motion.
Patient-Dependent Factors
Respiratory pattern: This factor has a huge influence on the amount of lung deposition. In spontaneous ventilation, a slow and deep inspiration followed by a voluntary apnea of 6–10 seconds increases the deposition by sedimentation. On the contrary, the cry of an infant prompts an opposite effect, since the inspiration is short and expiration prolonged, minimizing lung deposition. Some elements to consider in this regard are: age, respiratory rate, tidal volume, inspiratory/expiratory time ratio, and aerosol delivery time regarding the respiratory cycle.
Anatomy of the airway and respiratory mechanics: Anatomical alterations influence the deposition fraction (dose deposited/nominal dose) of an aerosol, since they affect gas flow velocity. In areas where the airway bifurcates (bronchial carinae), impaction of the particles may occur, depending on their inertia. In regions with anatomical (bronchial stenosis, mucous plug) or functional (bronchospasm) narrowness, a low deposition fraction may occur. Although it is true that slow flow favors deposition by sedimentation, this means that the total amount of gas that reaches the distal region is lower, so fewer aerosol particles are available to be deposited. This happens in areas with high resistance to gas flow.
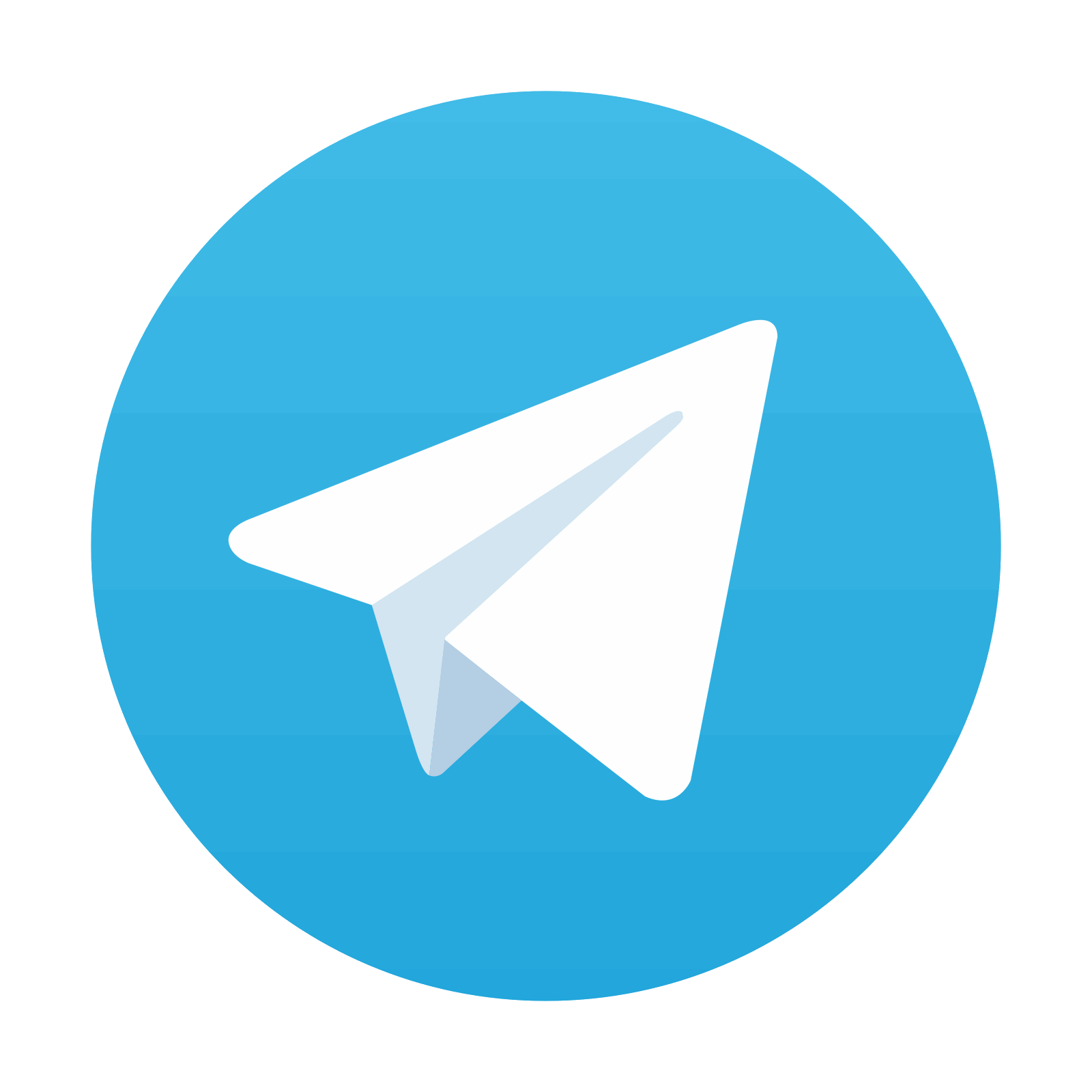
Stay updated, free articles. Join our Telegram channel

Full access? Get Clinical Tree
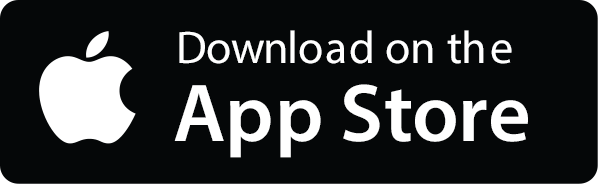
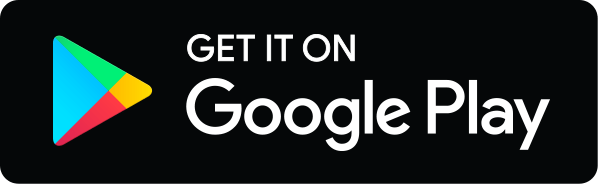