Theories involving DNA
DNA damage
mtDNA damage
Telomere shortening
Transposable element activation
Epigenetic modification
Error catastrophe
Accumulation theories
Clinker theories
Lipofuscin
Cross-links
Advanced glycation end products
Misfolded protein aggregates
Clunker theories
Mitochondria
Peroxisomes
Lysosomes
Systemic signaling theories
Endocrine
Immune
Stem cell
DNA/Genetic Theories of Aging
Genes provide the blueprint for RNA, proteins, and the enzymes that synthesize and catabolize nucleic acid, amino acid, carbohydrate, and lipid. The integrity of the genome is essential for reproductive fitness and survival. DNA sequences can be modified by spontaneous depurination reactions and errors in synthesis (internal stressors), as well as by ionizing radiation, aflatoxin, and alkylating agents (environmental stressors). Organisms have evolved error checking and repair mechanisms that depend on DNA encoded enzymes. The DNA damage theory holds that genes are susceptible to damage and mutations in DNA yield altered sequences that in turn change RNA and protein sequences. These mutations alter the function of structural, signaling, and/or repair molecules, and the accumulation of molecules with changed functional capacity yields the aging phenotype. Supporting evidence for this theory includes findings that whole-body irradiation shortens life span [8, 9], somatic mutations in some cells increase with age [10, 11], chromosomal abnormalities increase with age [12, 13], and mutations in genes involved in DNA metabolism are associated with a number of premature aging syndromes (Werner, Hutchinson-Gilford, ataxia telangiectasia, and Cockayne) [14]. While evidence of an association between DNA damage appears to be strong, whether it is a cause or a consequence of aging is still unclear [15].
The mitochondrial DNA damage theory proposed that damage to this particular organelle’s DNA accumulates over time, compromising mitochondrial function (energy production), producing more damaging metabolic by-products (reactive oxygen species, a type of highly energetic free radical containing molecule) that cause further cellular damage. The cell’s nucleus has a robust, evolved set of mechanisms for repairing nuclear DNA, while mtDNA does not. Other unique characteristics of mitochondria that contribute to their propensity for DNA damage and aberrant function include that mtDNA is in close proximity to the sites where reactive oxygen species are produced, aberrant mitochondria sometimes replicate faster than undamaged mitochondria, and while each cell translates only 7% of its nuclear DNA, almost all its mtDNA is translated (no spare “junk DNA” to absorb damage). The net results are reduced energy production, diminished control of other cell processes, increased reactive oxygen species, and accumulation of damaged harmful molecules and organelles leading to a progressive aging phenotype. Supporting evidences for this theory include mitochondrial dysfunction is a hallmark of aging [16], aberrant mitochondrial function is associated with an accelerated aging phenotype [17, 18], accumulation of mtDNA mutations is associated with the age-related loss of fast twitch (type II) muscle fibers [19], and damaged mitochondria can initiate cellular apoptosis [20].
The telomere theory of aging proposes that aging is a result of telomere shortening and limited cell proliferation. The failure in replication leads to deficits in cell replacement and tissue/organ renewal. Because the DNA replication machinery physically occupies space on the DNA, it can’t make a copy of the “final” sequence of DNA on the chromosome it is replicating. Telomeres are found at the ends of chromosomes and consist of a repetitive sequence. With every DNA replication, that piece of DNA under the polymerase machinery is not copied, and there is progressive shortening of the telomere length. While there is a specialized enzyme, telomerase, which maintains telomere length in stem cells, somatic cells have low levels of the enzyme. Cellular or replicative senescence occurs when telomeres reach a critical size. Supporting evidences include that telomere length is directly proportional to cell age [21], individuals with progerias have shorter telomeres [22], cancer and other immortal cells maintain a constant telomere length [23], and restoring telomerase enzyme in somatic cells in vitro causes an increase in replicative life span of these cells [24]. Additionally, cellular senescence has been linked with an altered expression pattern by cells that promotes a pro-inflammatory state – a chronic state associated with late life changes and morbidity [25]. Evidences that are not supportive include observations that telomere length is not related to life span as mouse telomeres are much longer than those in humans [26] and telomerase protects against replicative senescence but not cellular senescence triggered by other pathways (in response to DNA damage, reactive oxygen species, and activation of oncogenes).
The transposable element activation theory describes a particular mechanism that may contribute to increased somatic mutation with increasing age. Certain sequences of DNA (transposable elements) have the ability to move from one location in the genome to another, and their insertion in a different segment of DNA can lead to mutagenesis. This random insertion can yield mutations that serendipitously improve an organism’s fitness and favor evolutionary advance. Conversely, it can lead to DNA damage, replication errors, and genomic instability. As with the repair mechanisms of DNA described above, elaborate mechanisms have evolved to repress transposon activity. Supporting evidences include that transposition increases in frequency with age in mammals [27]; activation of transposable elements (loss of repression) is associated with progressive dysfunction of aging cells, induction of cell senescence, and cell loss [28]; and upregulating transposon activation in Drosophila brain caused progressive memory impairment and shortened lifespan [29].
The epigenetic theory of aging posits that epigenetic modifications alter gene expression patterns and cellular function yielding the aging phenotype. Epigenetics refers to changes in gene expression that alter cellular and physiological phenotypic traits but does not involve changes to the genetic code. Epigenetics plays a role in homeostasis through enabling external and/or environmental factors to modulate phenotype. The majority of cells in the human body are somatic cells, and their differentiation into specialized tissue is a result of epigenetic modifications. The differentiated phenotype is maintained, in part, through nucleic acid and protein interactions, DNA methylation, and histone acetylation. Noncoding RNAs (ncRNAs) that include microRNA, short interfering RNA, piwi-interacting RNA, and long ncRNA also function to regulate gene expression at the transcriptional and posttranscriptional level. These epigenetic changes can be influenced by age, lifestyle, and environment [30]. Supportive evidences for this theory include that DNA methylation increases with age [31], epigenetic silencing of repressive transcription factors can contribute to cells expressing a senescent phenotype [28], and epigenetic changes in mtDNA and mitochondrial ncRNAs not only impact mitochondrial structure, function, and dynamics but also regulate multiple homeostatic pathways including senescence, apoptosis, and energy metabolism [32].
Error catastrophe theory does not directly involve DNA; rather it concerns RNA and proteins involved in the transfer of DNA-encoded information. Normally, a steady state exists where low levels of random errors occur but cause little harm. These errors can be inaccuracies in transcription, proofreading, splicing, and transport of RNA and mistakes in amino acid sequences or in polypeptide folding and conformation for proteins. Error catastrophe theory holds that a critical threshold is crossed when the machinery for biosynthesis is sufficiently destabilized with increasing age such that the rate of errors increases and compromises macromolecular function. The theory predicts that old cells should contain significant levels of abnormal proteins and that mutations that diminish the fidelity of synthesis will accelerate aging . Testing of these two predictions has yielded equivocal results [33]. More recently, error catastrophe theory has been applied to pathological settings of mitochondrial mutations [34], tumor growth dynamics [35], and viral replication [36, 37].
Accumulation Theories
This group of theories focuses on aging as a consequence of the accumulation of cellular components or specific cell types with altered properties that compromise function leading to loss of tissue function and homeostasis . Clinker theories of aging involve specific macromolecules, often waste from metabolic processes which amass over time disrupting function. Lipofuscin is an oxidized lipid-containing product from catabolic reactions that accumulates in the lysosomes of long-lived postmitotic cells, such as neurons and cardiac myocytes. The failure to degrade lipofuscin further or eject it through exocytosis reduces lysosomal functional capacity (macromolecular catabolism, autophagy, receptor recycling, and cytoplasmic trafficking). Collagen cross-links in the skin and bone increase with increasing age and lead to altered properties of this scaffolding protein: loss of dermal elasticity and stiffer less flexible joints and bone. Similarly, the levels of advanced glycation end products increase with normal aging in multiple organ systems altering the biomechanical and functional properties of the molecular components with this nonenzymatic modification. Additionally, advanced glycation end products bind specific receptors that results in a pro-inflammatory immune response. Misfolded protein aggregates such as neurofibrillary tangles in the brain, amyloid deposition in the heart, and crystallin accumulation in the eye are thought to contribute to both normal aging and pathological changes. Whether the accumulation of compromised molecular components is a cause or a consequence of aging has been difficult to disentangle. While it is intuitive that the buildup of insoluble material will eventually compromise function [38], it is also possible that misfolded protein aggregation is a protective response [39, 40].
Clunker theories of aging involve cellular organelles such as mitochondria, lysosomes, peroxisomes, and cell nuclear membranes that have lost functional capacity through age-related changes in composition, structure, and/or metabolism. The role of aberrant mitochondria in aging initially focused on the accumulation of mtDNA damage that generates more reactive oxygen species which in turn causes further damage (see mitochondrial DNA damage theory above). The validity of that original theory has been questioned by observations that antioxidant treatment or genetic manipulation to either under- or over-express key enzymatic regulators of reactive oxygen species had equivocal effects on life span [41] and that reactive oxygen species appear to contribute to metabolic health and longevity [42]. This has led to a reformulation of a mitochondrial theory of aging where the emphasis has moved from reactive oxygen species production and mtDNA to other activities of the mitochondria, including biogenesis and turnover [43], calcium mobilization [44], cellular senescence [25], apoptosis [45], and epigenetic changes in mitochondrial DNA and by noncoding mitochondrial RNAs [32]. In this new mitochondrial theory of aging , mitochondrial dysfunction contributes to changes in key homeostatic pathways (apoptosis, senescence, and energy metabolism) that cause cell dropout and altered phenotypic expression and energy metabolism that are associated with an aging phenotype.
The peroxisome theory of aging posits that cumulative damage to these organelles compromises the multiple functions of this organelle contributing to cell death and aging. Peroxisomes, like mitochondria, are organelles that can multiply by fission and are involved in the metabolism of reactive oxygen species and in antiviral innate immunity (detecting cytosolic viruses) [46]. Peroxisomes breakdown long-chain fatty acids through beta-oxidation, synthesize ether phospholipids and bile acids, and resupply mitochondria with metabolic (tricarboxylic acid cycle) intermediates. Evidence in support of peroxisome modulation of organelle and cellular aging includes peroxisome activity in deactivating reactive oxygen species with antioxidant enzymes [47], converting nicotinamide to nicotinic acid (an NAD+ salvage pathway) in response to caloric restriction and various mild stressors (treatments which increase longevity) [48], and operating as a modulator of levels of non-esterified fatty acids which accelerate age-related necrotic and apoptotic cell death [49] and diacylglycerol which sensitizes cells to age-related stresses [50, 51].
Lysosomes were originally described by Christian de Duve as “suicide bags” of hydrolases that played a role in cell death. The lysosome theory of aging proposes that dysfunction of this organelle leads to an aging phenotype. Lysosomes are the major degradative compartment of the endosomal/lysosomal system. They also play roles in other cellular processes, including nutrient sensing, cell development, differentiation and apoptosis, and resistance to stress. Lysosomes are where cellular components are disassembled for recycling (autophagy) and where damaged, defective organelles also undergo cellular component disassembly (e.g., mitophagy, pexophagy). Evidence in support of this theory includes that lysosomes carry out autophagy which catabolizes macromolecules to facilitate survival during temporary starvation [52] and removes damaged proteins and organelles as part of normal cellular maintenance [43]. Additionally, lysosomes are a potential source of lipofuscin [53] which can contribute to their own cumulative dysfunction and lead to an error cascade when lysosomes become dysfunctional and damage proteins and organelles (mitochondria, peroxisomes) accumulate causing cellular dysfunction [54]. This loss of lysosome function provides a potential mechanism for increased cell and tissue loss of function with advancing age.
Systemic Signaling Theories
The endocrine theory of aging holds that loss of homeostasis occurs systemically when hormone levels and signaling change with age and that the aging phenotype arises from dysregulated hormone signaling. Hormones provide an organism-wide system of signaling. Hormone activity depends upon synthesis, secretion, and binding to a target receptor. The production of many hormones changes with increasing age. Their secretion patterns (e.g., pulsatile, diurnal) also alter. In addition, the number and functional ability of hormone receptors to transduce signals in target organs are reduced. For example, many organs decrease in size with age; age-related deficits in the levels of growth hormone or sex steroid hormones negatively affect target organs’ size and ability to repair and maintain functional capacity. Evidence in support of this theory includes the role of changes in growth hormone-IGF-I axis and the aging phenotype of diminished mass [55], hypothalamic-pituitary-adrenal axis, dysregulated cortisol, and stress response [56].
The immune theory of aging asserts that diminished systemic defensive and repair responses negatively affect the functional capacity of other organ systems and contribute to the aging phenotype. The immune system has evolved to enable slow-growing organisms to respond to and keep in check rapidly growing pathogens/parasites as well as to deal with wound repair and remodeling of specialized tissue. Innate immune mechanisms consisting of humoral mechanisms (coagulation cascade, complement) and cellular components (natural killer cells, neutrophils, macrophages) and acquired immune mechanisms involving humoral (antibodies) and cellular (T and B lymphocytes) components provide a highly evolved homeostatic system. Increasing age is associated with “immunosenescence” which is a gradual decline in the acquired immunity that has been associated with increased morbidity and mortality in late life. “ Inflammaging” is a corollary theory concerning an aging-related increase in innate immunity activity, perhaps as a compensatory mechanism for declining acquired immunity. A ramped up innate system is marked by increased levels of cytokines (such as interleukin-6) and macrophage activation that perpetuates a chronic pro-inflammatory state. The effect of chronic repair and remodeling programs of inflammation results in alterations in protease and growth factor levels, tissue structure, and cellular organization, which negatively alter organ system function. The evidence in support of the immune theory of aging includes associations of immunosenescence with altered function in aging such as decrease in the number of naïve lymphocytes and clonal expansion of memory cells impacting ability to respond to new immune challenges [57], altered functional capacity of multiple immune cell types [58–61], associations of immunosenescence, and inflammaging with age-related diseases and geriatric syndromes [62–64].
The stem cell theory of aging hypothesizes that pluripotent cells exhibit reduced regenerative capacity with age, and this inability to replenish cellular supplies gives rise to the aging phenotype. Hematopoietic stem cells in the bone marrow and adult stem cells in the many tissues and in circulation maintain homeostasis by replenishing depleted reserves. Satellite cells that contribute to muscle mass and repair of muscle tissue, osteoprogenitor cells that replenish osteoblasts and form bone, neural stem cells that give rise to neurons, and astrocytes and oligodendrocytes in the brain are examples of adult stem cells that mediate regeneration and repair. With increasing age, precursor cells become depleted. This deficit in regenerative capacity has multiple potential causes including phenotypic drift, precursor arrest or injury, illness, and environmental challenge consuming progenitors leading to cellular burnout. Evidence in support of this theory includes studies of age-associated cumulative DNA damage driving cell loss for hematopoietic stem cells and hair follicle stem cells [65, 66] and the shift in the pathway commitment of bone mesenchymal stem cells away from osteoblastic or myoblastic cells toward the adipocytic cell lineage being associated with osteopenia, sarcopenia, and an increase in fat content in bone marrow and muscle [67].
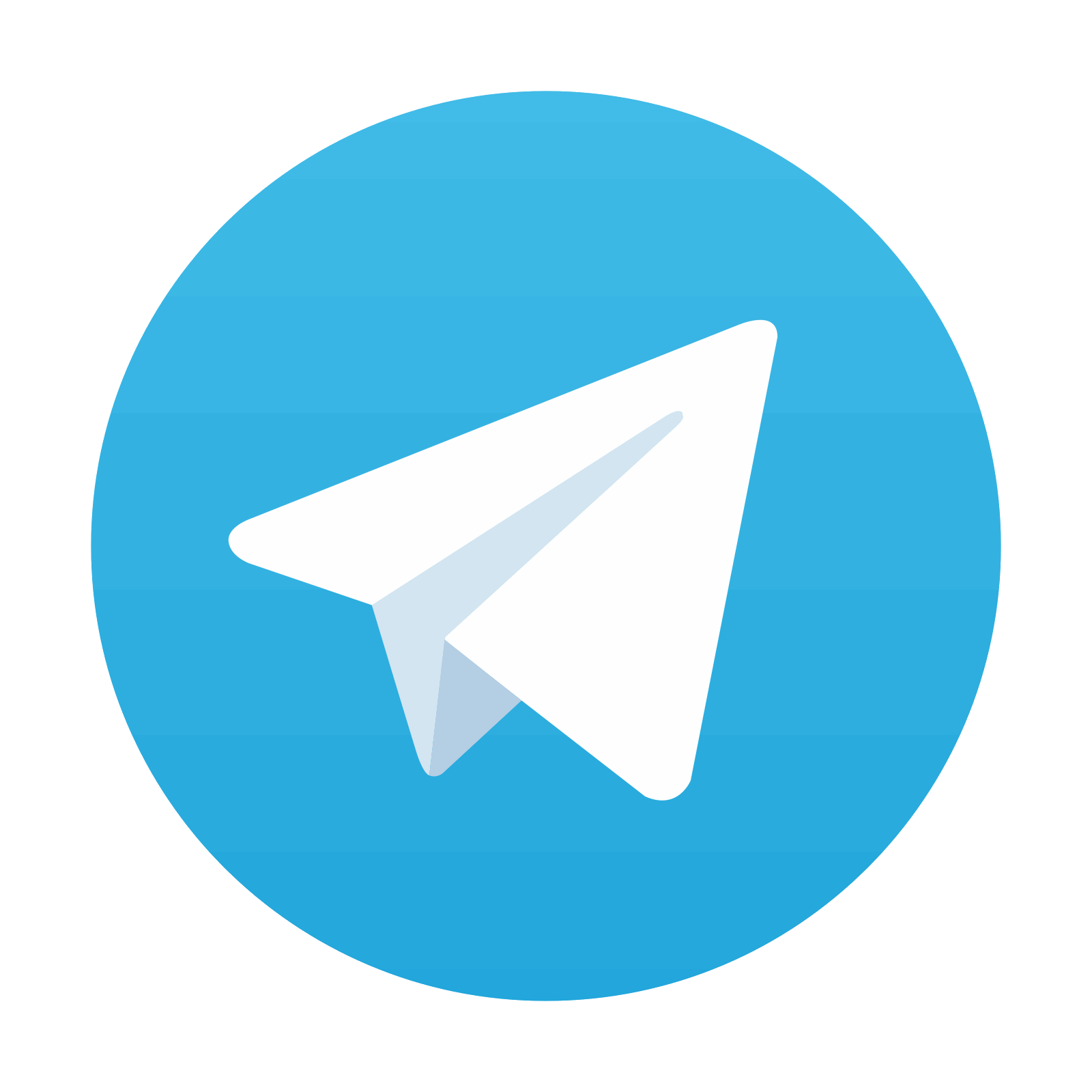
Stay updated, free articles. Join our Telegram channel

Full access? Get Clinical Tree
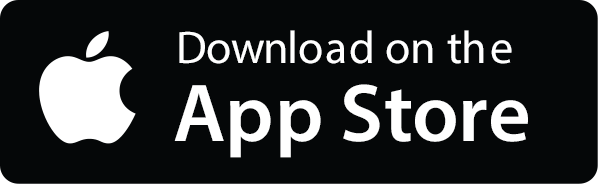
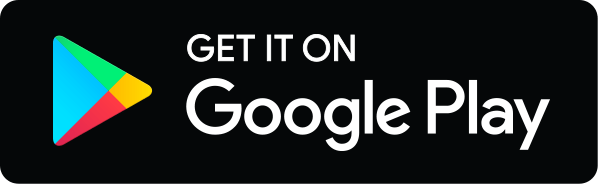