Fentanyl
Alfentanil
Sufentanil
Remifentanil
Morphine
Methadone
Meperidine
Hydromorpho
Volumes (L)
V1
12.7
2.2
17.8
4.9
17.8
7.7
18.1
11.5
V2
50
7
47
9
87
12
61
115
V3
295
15
476
5
199
184
166
968
Clearances (L/min)
Cl1
0.62
0.20
1.16
2.44
1.26
0.13
0.76
1.33
Cl2
4.82
1.43
4.84
1.75
2.27
2.19
5.44
3.45
Cl3
2.27
0.25
1.29
0.06
0.33
0.38
1.79
0.92
Exponents (min−1)
α
0.67
1.03
0.48
0.96
0.23
0.50
0.51
0.51
β
0.037
0.052
0.030
0.103
0.010
0.025
0.031
0.012
γ
0.0015
0.0062
0.0012
0.0116
0.0013
0.0005
0.0026
0.0005
Half-lives (min)
t1/2α
1.03
0.67
1.43
0.73
2.98
1.38
1.37
1.35
t1/2β
19
13
23
7
68
28
22
59
t1/2γ
475
111
562
60
548
1377
271
1261
Blood-brain equilibration
k e0 (min−1)
0.147
0.770
0.112
0.525
0.005
0.110
0.067
0.015
t1/2 k e0 (min)
4.7
0.9
6.2
1.3
139
6.3
10.
46
Tpeak (min)
3.7
1.4
5.8
1.6
93.8
11.3
8.5
19.6
VD peak effect (L)
76.9
6.0
94.9
17.0
590.2
30.9
143.3
383.3

Fig. 18.1
The time course of plasma concentration following a bolus of fentanyl, alfentanil, sufentanil, remifentanil, morphine, methadone, meperidine, and hydromorphone, based on the pharmacokinetics shown in Table 18.1. The y-axis is the percent of the initial concentration, which by definition is 100% at time 0, permitting display of the relative time courses of these opioids independent of the dose administered
The plasma is not the site of drug effect, and thus the time course of concentration seen in Fig. 18.1 will not reflect the time course of effect-site concentration or behavioral activity. By incorporating the plasma–effect-site equilibration delay into our calculations, we can examine the time course of the onset of drug effect , as shown in Fig. 18.2. In this case, we have normalized the effect-site concentrations to peak effect concentration [73] to again permit comparisons of the time course of drugs independent of the differences in potency. Alfentanil and remifentanil both reach a peak about 1.5 min after bolus injection, although the overall remifentanil drug effect is more evanescent. The peak fentanyl concentration occurs about 3.5 min after bolus injection, whereas the peak sufentanil effect is about 6 min after bolus injection. Methadone and meperidine are nearly indistinguishable following bolus injection, each reaching a peak about 12 min after a bolus. The peak for hydromorphone is 15–20 min after the bolus. Morphine is the outlier in terms of onset. Five minutes after a bolus injection, morphine is at 50% of the peak concentration. However, morphine reaches its peak concentration in the effect site about 90 min after the bolus injection. Table 18.1 shows the time to reach peak concentration for each of the opioids, as well as the volume of distribution at the time of peak effect, which is useful for calculating initial loading doses [74–76].


Fig. 18.2
The time course of effect-site concentration following a bolus of fentanyl, alfentanil, sufentanil, remifentanil, morphine, methadone, meperidine, and hydromorphone, based on the pharmacokinetics and rate of plasma–effect-site equilibrium shown in Table 18.1. The curves have been normalized to the peak effect-site concentration, permitting comparison of the relative rate of increase independent of dose. The times to peak effect correspond to those shown in Table 18.1
One clinical applications of the time course of drug effect following bolus injection is to guide programming of the lockout of PCA devices. A 10-min lockout for hydromorphone and methadone is a logical choice, because patients are able to make a decision to redose themselves after reaching peak drug effect. The slower onset of morphine is somewhat problematic, because patients will administer another dose while the prior dose is still reaching peak effect, creating the possibility of stacking bolus doses.
Considerable attention is given to “equianalgesic dosing” of opioids. The calculation of the equianalgesic dose is complicated by the relative intrinsic potency of the opioids, the different pharmacokinetic profiles, and the large differences in the rate of blood–brain equilibration. Table 18.2 shows equianalgesic doses of frequently used opioids, based on the “minimum effective analgesic concentrations” or “MEAC” (also called “MEC”) of fentanyl [77], alfentanil [78], sufentanil,5 remifentanil,6 morphine [80],7 methadone [81], meperidine [82], and hydromorphone [72, 83].8 Reflecting anesthesiologists’ familiarity with fentanyl, all of the calculations have been made using fentanyl as the reference opioid. The calculation of an equianalgesic bolus dose depends on when the observation of drug effect is made. For example, because fentanyl has a very rapid onset, and morphine has a very slow onset, 5 mg of morphine has the same effect at 10 min as 50 μg of fentanyl, whereas 60 min after the dose, 1 mg of morphine has the same effect as 50 μg of fentanyl. Similarly, because the drugs accumulate during infusions at different rates, the relative potencies of the opioids change depending on how long the infusion has been running, as shown in Table 18.2.
Table 18.2
Relative potency of frequently used opioids, based on the time of the observed effect
Fentanyl | Alfentanil | Sufentanil | Remifentanil | Morphine | Methadone | Meperidine | Hydromorphone | |
---|---|---|---|---|---|---|---|---|
MEAC (ng/mL) | 0.6 | 14.9 | 0.056 | 1.0 | 8 | 60 | 250 | 1.5 |
Equipotent bolus dose at: | (μg) | (μg) | (μg) | (μg) | (mg) | (mg) | (mg) | (mg) |
Peak effect | 50 | 92 | 5.5 | 17 | 4.9 | 1.9 | 37 | 0.6 |
10 min | 50 | 197 | 4.4 | 72 | 5.3 | 1.4 | 28 | 0.4 |
30 min | 50 | 174 | 3.9 | 282 | 2.0 | 0.9 | 17 | 0.2 |
Figure 18.3 shows the increase in effect-site concentration during a continuous infusion for each of these opioids. As expected, remifentanil increases the fastest, whereas methadone increases the slowest. Note, however, that even after 10 h of drug administration, most of these opioids are only at 60–80% of the eventual steady-state concentration. This speaks to the problem of background infusions for PCA. Even after many hours, patients are not at steady state, and the increasing drug concentration from the background infusion may expose a patient to toxicity 12–24 h after initiation of the infusion. Given the increased sensitivity of elderly patients to the effects of opioids, background infusions are likely a particularly poor choice in this population.


Fig. 18.3
The increase to steady state during an infusion of fentanyl, alfentanil, sufentanil, remifentanil, morphine, methadone, meperidine, and hydromorphone, based on the pharmacokinetics and rate of plasma–effect-site equilibrium shown in Table 18.1. The curves have been normalized to the steady-state effect-site concentration, permitting comparison of the relative rate of increase independent of infusion rate. Only remifentanil and alfentanil are at steady state after 10 h of continuous infusion
Offset
The offset of drug effect is a function of both the pharmacokinetic behavior and the rate of blood–brain equilibration. The “context-sensitive half-time” [73, 84] is a useful way to consider the plasma pharmacokinetic portion of the offset time, as shown in Fig. 18.4. The x-axis on Fig. 18.4 is the duration of an infusion that maintains a steady concentration of drug in the plasma. The y-axis is the time required for the concentrations to decrease by 50% after the infusion is terminated. Remifentanil’s pharmacokinetics are so fast that the context-sensitive half-time blurs right into the x-axis. Perhaps surprisingly, fentanyl is the outlier here. Fentanyl accumulates in fat, and so an infusion that maintains a steady concentration in the plasma winds up giving patients a large dose of fentanyl, resulting in slow recovery. Meperidine similarly shows long recovery. Note that for infusions of less than 10 h, morphine, hydromorphone, and sufentanil are nearly indistinguishable based on the plasma pharmacokinetics.


Fig. 18.4
The “context-sensitive half-time” (50% plasma decrement time) for fentanyl, alfentanil, sufentanil, remifentanil, morphine, methadone, meperidine, and hydromorphone, based on the pharmacokinetics shown in Table 18.1. Remifentanil shows virtually no accumulation over time with continuous infusion s, whereas the offset of fentanyl changes considerably as it is administered to maintain a steady plasma concentration
Once again, we have to consider that the plasma is not the site of drug effect. Therefore, we must consider the 50% effect-site decrement time [73, 85], as shown in Fig. 18.5. Because fentanyl and remifentanil have very rapid plasma–effect-site equilibration, they have changed little between Figs. 18.4 and 18.6. Note , however, the huge change for morphine and hydromorphone. One might have thought from Fig. 18.4 that these drugs would result in rapid offset of drug effect following a continuous infusion . This is clearly not the case, because the blood–brain equilibration delay results in these drugs having far slower offset than alfentanil or sufentanil. The “surprise” here is methadone. One would rarely think of methadone as a reasonable choice for infusion during anesthesia, but the pharmacokinetics of methadone suggest that it might be a reasonable choice for anesthetics of 4 h or less.



Fig. 18.5
The 50% effect-site decrement curves for fentanyl, alfentanil, sufentanil, remifentanil, morphine, methadone, meperidine, and hydromorphone, based on the pharmacokinetics and rate of plasma–effect-site equilibrium shown in Table 18.1. For drugs with rapid plasma–effect-site equilibrium, the 50% effect-site decrement curve closely follows the context sensitive half-time curve. However, for drugs with slow plasma–effect-site equilibration, a 50% decrement in effect-site concentration is considerably slower than a 50% decrement in plasma concentration (e.g., morphine)

Fig. 18.6
The 20% effect-site decrement curves for fentanyl, alfentanil, sufentanil, remifentanil, morphine, methadone, meperidine, and hydromorphone, based on the pharmacokinetics and rate of plasma–effect-site equilibrium shown in Table 18.1. The effect-site levels of all opioids, except morphine, will decrease by 20% quickly when an infusion is terminated. The slower decrease for morphine is because of its slow plasma–effect-site equilibration
Figure 18.6 shows the 20% effect-site decrement curve for these eight opioids. Figure 18.6 speaks to how often one might expect to redose a patient with chronic pain who is titrating the analgesic level to a just-adequate concentration. Because of its slow blood–brain equilibration, morphine would need to be given approximately every 2 h. Hydromorphone, fentanyl, and methadone would need to be given approximately every hour.
Specific Opioids
Morphin e
Morphine has three unique aspects among the opioids frequently used in anesthesia practice: it is an endogenous ligand of the μ receptor, has an active metabolite, and has a very slow onset of effect. Morphine was initially identified in the brains of mice that had never been exposed to exogenous morphine [86]. It has subsequently been found in the brains of cows [87], rats [88], and humans [89]. Codeine has also been identified as an endogenously synthesized substance. However, because codeine is mostly an inactive prodrug of morphine, its presence in the brain does not diminish morphine’s distinction as the only endogenous ligand of the μ receptor that is also a frequently administered drug.
Morphine is metabolized by glucuronidation into two metabolites, morphine-3-glucuronide, which is mostly inactive, and morphine-6-glucuronide, which is itself a potent analgesic [90]. Although the potency of intrathecal morphine-6-glucuronide is 650-fold higher than that of morphine [91], morphine-6-glucuronide crosses the blood–brain barrier very slowly, so slowly that it is unlikely that it contributes to the acute analgesia provided by morphine [92, 93]. However, with chronic administration, the levels of morphine-6-glucuronide will increase to pharmacologically active concentrations [94].
Morphine-6-glucuronide is eliminated by the kidneys [95]. Creatinine clearance is reduced with advancing age, as shown in the often-cited equation of Cockroft and Gault [96]:
![$$ {\displaystyle \begin{array}{l}\begin{array}{l}\mathrm{Men}:\mathrm{Creatinine}\ \mathrm{clearance}\ \left(\mathrm{mL}/\min \right)\hfill \\ {}=\left\{\left[140-\mathrm{age}\left(\mathrm{years}\right)\right]\times \mathrm{weight}\left(\mathrm{kg}\right)\right]\Big\}\hfill \\ {}\kern0.50em \ \kern0.63em \ \left[72\times \mathrm{serum}\ \mathrm{creatinine}\ \left(\mathrm{mg}\%\right)\right]\hfill \end{array}\hfill \\ {}\mathrm{Women}:85\%\mathrm{of}\ \mathrm{the}\ \mathrm{above}.\hfill \end{array}} $$](https://i0.wp.com/thoracickey.com/wp-content/uploads/2018/01/A371425_3_En_18_Chapter_Equa.gif?w=960)
![$$ {\displaystyle \begin{array}{l}\begin{array}{l}\mathrm{Men}:\mathrm{Creatinine}\ \mathrm{clearance}\ \left(\mathrm{mL}/\min \right)\hfill \\ {}=\left\{\left[140-\mathrm{age}\left(\mathrm{years}\right)\right]\times \mathrm{weight}\left(\mathrm{kg}\right)\right]\Big\}\hfill \\ {}\kern0.50em \ \kern0.63em \ \left[72\times \mathrm{serum}\ \mathrm{creatinine}\ \left(\mathrm{mg}\%\right)\right]\hfill \end{array}\hfill \\ {}\mathrm{Women}:85\%\mathrm{of}\ \mathrm{the}\ \mathrm{above}.\hfill \end{array}} $$](https://i0.wp.com/thoracickey.com/wp-content/uploads/2018/01/A371425_3_En_18_Chapter_Equa.gif?w=960)
This reduction means that the creatinine clearance of an 80-year-old patient will be about half that of a 20-year-old patient. Thus, morphine-6-glucuronide will accumulate more in elderly patients, necessitating a reduction in the dose of chronically administered morphine. Of course, if the patient has renal insufficiency, it might be better to select an opioid without an active metabolite.
The second unique aspect of morphine is the slow onset of effect. The peak effect following a bolus dose of morphine occurs approximately 90 min after the bolus. This has been demonstrated using pupillometry [97–99], ventilatory depression [98], and analgesia [99] as measures of morphine drug effect. The likely explanation for this is that morphine is a substrate for P-glycoprotein, which actively transports morphine out of the central nervous system [100].
Figure 18.7 shows a simulation of the analgesic (y-axis > 1) and ventilatory (y-axis < 1) effects of three different morphine doses: a bolus of 0.2 mg/kg, a bolus of 0.2 mg/kg followed by an infusion of 1 mg/70 kg per hour, and repeated boluses of 0.1 mg/kg every 6 h [101]. The solid line is the median prediction, whereas the shaded area represents the 95% confidence bounds. As seen in Fig. 18.7, the time course of analgesia and ventilatory depression is similar, although the analgesia wanes somewhat faster than the ventilatory depression.


Fig. 18.7
Simulated analgesic (y > 1) and ventilatory (y < 1) effects of three different doses of morphine: 0.2 mg/kg (a), 0.2 mg/kg plus an infusion of 1 mg/70 kg/h (b), and a bolus of 0.1 mg/kg every 6 h (c). The analgesic and ventilatory effects peak concurrently, about 90 min after the morphine bolus. Because the concentration versus response relationship is steeper for analgesia than ventilatory depression, the analgesic effect dissipates before the ventilatory depression (Reprinted from Dahan et al. [101]. With permission from Wolters Kluwer Health, Inc.)
It is important to appreciate the slow onset of morphine when titrating to effect. Aubrun and colleagues [102, 103] have advocated postoperative titration of morphine in elderly patients by administering 2–3 mg boluses every 5 min. This is not logical for a drug with a peak effect about 1.5 h after bolus injection. It is surprising that Aubrum and colleagues did not see any toxicity with this approach, given the potential for accumulation with repeated titration of small doses of morphine to effect. However, it does explain why their study is unique in finding that elderly patients require the same amount of opioid as younger patients.
Meperidine
Meperidine, also called “pethidine,” has little role in the management of pain. Meperidine is still a popular drug because of the familiarity of its use, particularly among surgeons and obstetricians. Meperidine is unique among opioids in that it has significant local anesthetic activity [104, 105]. Meperidine has been used as the sole analgesic intrathecally for obstetric anesthesia, but its benefit over a combination of local anesthetic with another opioid is unclear. The only unique perioperative role for meperidine is the treatment of postoperative shivering, in which doses of 10–20 mg are typically effective.
The problems with meperidine are its complex pharmacology and its toxic metabolite. Holmberg and colleagues [106] examined the pharmacokinetics of an intravenous meperidine bolus in young and elderly surgical subjects. They found that elderly patients had reduced meperidine clearance, resulting in a longer half-life for meperidine. There was minimal change in the initial volume of distribution. The clinical implication is that the initial dose of meperidine in elderly subjects should not be reduced based on pharmacokinetics, but meperidine will accumulate in elderly subjects with repeated administration. This makes meperidine a particularly poor choice for administration by PCA in elderly patients [107].
A worrisome aspect of meperidine is the toxic metabolite, normeperidine (or “norpethidine”). In a subsequent study, Holmberg and colleagues examined the renal excretion of both meperidine and normeperidine in elderly surgical patients [108]. Renal excretion was reduced in elderly patients, particularly for normeperidine. The result is that normeperidine will likely accumulate with repeated doses in elderly patients. Because normeperidine is highly epileptogenic, meperidine is probably a poor choice for PCA or other forms of continuous opioid delivery in elderly patients.
Meperidine has several other unique aspects to its pharmacology. It is the only negative inotrope among the opioids [109]. Meperidine also has intrinsic anticholinergic properties, which can result in tachycardia. Elderly patients with coronary artery disease are clearly at risk of adverse events if given drugs that have negative inotropic or positive chronotropic effects.
Last, meperidine is associated with several unusual reactions, including the potential for acute serotonergic syndrome when combined with monoamine oxidase (MAO)-A inhibitors and a significant increase of delirium in elderly patients compared to other opioids [110]. Fortunately, the classic MAO-A inhibitors, phenelzine (Nardil), tranylcypromine (Parnate), and isocarboxazid (Marplan), are now rarely used. Selegiline, often used in Parkinson’s disease, is a weak MAO-B inhibitor and has been implicated in one nonfatal interaction with meperidine [111]. However, given the polypharmacy common in elderly patients, it would seem wise to avoid using meperidine when opioids with more selective pharmacology and inactive metabolites are available.
Hydromorphone
Hydromorphone in many aspects acts as a rapid-onset morphine. However, it lacks the histamine release associated with morphine and does not have active metabolites. There are no studies explicitly examining the role of age in hydromorphone pharmacokinetics or pharmacodynamics. In fact, there are surprisingly few studies examining the perioperative use of hydromorphone. Keeri-Szanto [112] found intraoperative hydromorphone to be approximately eight times more potent than morphine, with a half-life of 4 h versus 5 h for morphine. Kopp et al. [113] investigated whether 4 mg of hydromorphone provided any evidence of preemptive analgesia and found that it did not.
Rapp and colleagues [114] compared hydromorphone PCA to morphine PCA in postoperative patients following lower abdominal surgery. They found that hydromorphone PCA was associated with better mood scores, but with increased incidence of nausea and vomiting. They found that 1 mg of hydromorphone was approximately equianalgesic with 5 mg of morphine. This is about half as potent as suggested by Hill and Zacny [72], who determined that hydromorphone was tenfold more potent than morphine. Although Rapp and colleagues did not specifically study the effects of age, one would expect this ratio to be independent of age in the immediate postoperative period. Because morphine has an active metabolite that accumulates and hydromorphone does not, the apparent potency of morphine relative to hydromorphone may increase with chronic administration.
Lui and colleagues [115] compared epidural hydromorphone to intravenous hydromorphone, both administered by PCA in a double-blind/double-dummy protocol. They found more pruritus in patients receiving epidural hydromorphone, but no differences in postoperative analgesia , bowel function, or patient satisfaction. Overall, hydromorphone in the epidural group was half of that in the intravenous group, indicating that hydromorphone is acting spinally when administered via the epidural route. Hydromorphone and morphine both reach their peak concentrations in the cervical cerebrospinal fluid about 60 min after epidural administration [116], suggesting they have similar potential for delayed ventilatory depression after epidural administration. In a study of obstetric patients, Halpern and colleagues [117] found 0.6 mg of hydromorphone to be clinically indistinguishable from 3 mg of morphine, consistent with the 1: 5 relative potency reported for intravenous hydromorphone and morphine in the postoperative period.
Fentanyl
Fentanyl is among the “cleanest” opioids in terms of pharmacology. It has a rapid onset, predictable metabolism, and inactive metabolites. It is (obviously) the first of the “fentanyl” series of opioids, notable for their rapid metabolism and selective μ potency. It is the only one of the opioids that is available for transdermal and transmucosal delivery, although these methods of administration are being investigated for sufentanil as well.
Bentley et al. [118] studied aging and fentanyl pharmacokinetics in young and elderly groups of patients. They found that fentanyl clearance was decreased among the elderly, resulting in a prolonged half-life.
Scott and Stanski [63] used high-resolution arterial sampling during and after a brief fentanyl infusion to characterize the influence of age on the pharmacokinetics of fentanyl. These investigators did not find any effect of age on the pharmacokinetics of fentanyl or alfentanil, except for a small change in rapid intercompartmental clearance.
The minimal influence of age on the pharmacokinetics of fentanyl was subsequently confirmed by Singleton and colleagues [119]. These investigators found no change in the dose-adjusted concentration of fentanyl between young and elderly patients, except for a transient increase in concentration in elderly individuals at 2 and 4 min after the start of the infusion. These findings are consistent with the decreased rapid intercompartmental clearance reported by Scott and Stanski.
Scott and Stanski used the EEG as a measure of drug effect to estimate the potency of fentanyl [63, 120]. They observed a decrease of approximately 50% in the dose required for 50% of maximal EEG suppression (C50) from age 20 to age 85, as shown in Fig. 18.8. Because the pharmacokinetics of fentanyl seem nearly unchanged by age, it is likely that elderly patients require less fentanyl because of intrinsic increased sensitivity to opioids. Put another way—the elderly brain is twice as sensitive to opioids as a younger brain. This predicts that elderly patients require half of the fentanyl that younger patients require. Because the pharmacodynamics of fentanyl (i.e., the C50) is affected by age, and not the pharmacokinetics, the offset of fentanyl drug effect in elderly patients who receive an appropriately reduced dose of fentanyl should be as fast as it is in younger patients.


Fig. 18.8
The influence of age on the 50% maximal effective dose (C50) of fentanyl, as measured by electroencephalogram depression. Although there is considerable variability, overall there is about a 50% reduction in C50 from age 20 to age 80, reflecting increased brain sensitivity. This has been shown for alfentanil [66] and remifentanil [68] and appears to be a class effect of opioids (Adapted with permission from Scott and Stanski [63]. With permission from American Society for Pharmacology and Experimental Therapeutics)
The 50% reduction in fentanyl suggested by Scott and Stanski’s integrated pharmacokinetic/pharmacodynamic model is in reasonable agreement with an analysis by Martin and colleagues [121] of intraoperative fentanyl utilization. Using the automated electronic record system in place at Duke University Hospital, they found that intraoperative doses of fentanyl decreased by about 10% per decade after age 30.
Other Fentanyl Delivery Systems
Fentanyl is also available in two unique dosage forms : oral transmucosal fentanyl citrate and transdermal fentanyl. Holdsworth and colleagues [122] studied pharmacokinetics and tolerability of a 20-cm2 transdermal fentanyl patch in young and elderly subjects. Plasma fentanyl concentrations were nearly twofold higher in the elderly subjects compared with younger subjects, reflecting either increased absorption or decreased clearance. Given that fentanyl clearance seems unchanged in the elderly, the likely explanation is that transdermal fentanyl absorption is more rapid in elderly patients, possibly because the skin is thinner and poses less of a barrier to fentanyl absorption. The increased concentrations in elderly subjects were associated with increased adverse events—so much so that the patch was removed for the study in every elderly subject, whereas none of the patches were removed in younger subjects.
Davis and colleagues [123] also noted that the time course of absorption of fentanyl through the skin is delayed in the elderly, with subcutaneous fat acting as secondary reservoir leading to prolonged release even after the removal of the patch.
Kharasch and colleagues [124] examined the influence of age on the pharmacokinetics and pharmacodynamics of oral transmucosal fentanyl citrate (the fentanyl “lollipop”). They found no change in the pharmacokinetics of fentanyl with age, including the absorption characteristics of the buccal mucosa. Perhaps unexpectedly, they also found no increase in sensitivity to fentanyl, as measured by pupillary miosis . Thus, in their view, the data do not support reducing the dose of oral transmucosal fentanyl citrate in elderly patients.
Alfentanil
The relationship between opioids and age becomes more complex when we consider alfentanil. Scott and Stanski [63] reported similar findings for alfentanil as previously described for fentanyl. In particular, they did not find any effect of age on the pharmacokinetics of alfentanil, except for a small change in the terminal half-life. Shafer et al. [125] also reported no relationship between age and alfentanil pharmacokinetics. Sitar and colleagues [126] reported a modest decrease in alfentanil clearance and central compartment volume in elderly subjects. In a study that used historical control data, Kent and colleagues [127] also reported a modest decrease in alfentanil clearance with advancing age. Lemmens et al. [128] observed that the pharmacokinetics of alfentanil in men (as studied exclusively by Scott and Stanski) were unaffected by age, whereas the pharmacokinetics in women showed a clear negative correlation between age and clearance.
In an effort to sort out these modestly conflicting results, Maitre et al. [129] pooled alfentanil concentration data from multiple prior studies and performed a population pharmacokinetic analysis to estimate the influence of age and gender on the pharmacokinetics of alfentanil. Maitre et al. found that clearance decreased with age and that the volume of distribution at steady state increased with age, the net effect being a longer terminal half-life with increasing age. That might sound like the end of the story, except that Raemer and colleagues [130] prospectively tested the Maitre et al. pharmacokinetics in two groups of patients, young women and elderly men, using computer-controlled drug administration. In this prospective test, the pharmacokinetics reported by Maitre et al. did not accurately predict the observed plasma alfentanil concentrations. However, pharmacokinetics reported by Scott and Stanski, which predict no influence of age or gender on alfentanil pharmacokinetics, accurately predicted the concentrations in both young women and elderly men. From these results, we can conclude that pharmacokinetics of alfentanil does not change in a clinically significant manner with age.
Although they found no change in pharmacokinetics with age, Scott and Stanski demonstrated that the C50 for EEG depression with alfentanil decreased by 50% in elderly subjects, nearly identical to the increased potency of fentanyl in elderly subjects [66]. This would suggest that, based on pharmacokinetic alterations with age, the dose of alfentanil in elderly patients should be about half of the dose that would be used in younger patients. Unfortunately, subsequent studies by Lemmens et al. [131–133], based on clinical endpoints, found no influence of age on the pharmacodynamics of alfentanil. However, Lemmens et al. [134] observed that the alfentanil dose required to maintain adequate anesthesia, when administered by target-controlled infusion, was decreased by approximately 50% in elderly subjects. Thus, Lemmens et al. saw a similar change in dose-response relationship, in that the elderly required half as much opioid as younger subjects, but could not explain it as a pharmacodynamic difference. However, it is a bigger difference in concentration than any of the pharmacokinetic studies would have predicted, and there was no control group—the control group was a historical control group.
Where this leaves us is that there are many studies suggesting that the alfentanil dose in elderly subjects is about half of the dose in younger subjects. The available data suggest that the change is probably pharmacodynamic, but there may be a pharmacokinetic component to the increased sensitivity as well. If the change is mostly pharmacodynamic, perhaps, with a modest change in terminal half-life in elderly subjects, then the offset of alfentanil should be as fast in older subjects as it is in younger subjects, provided the dose has been appropriately reduced.
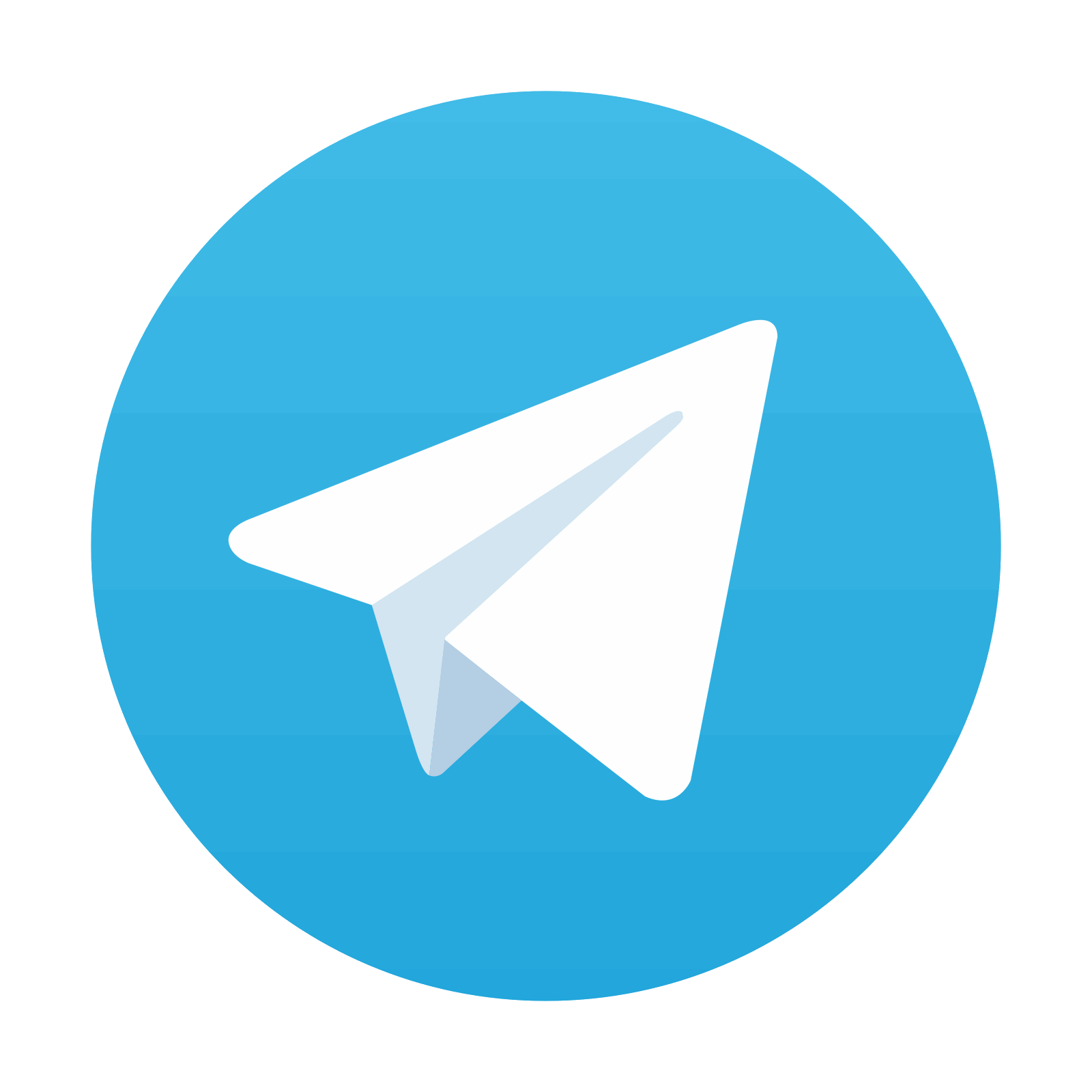
Stay updated, free articles. Join our Telegram channel

Full access? Get Clinical Tree
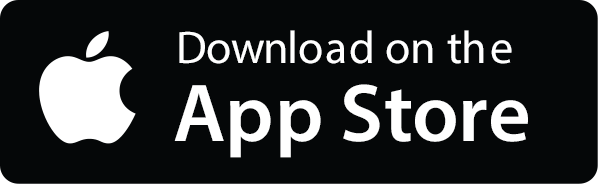
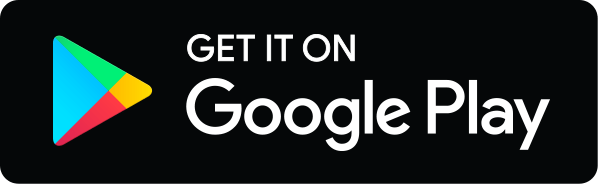