Nearly 10% of the population older than 65 years has atherosclerotic lower extremity peripheral arterial disease. Older patients and those with risk factors may have up to a 25% incidence of lower extremity peripheral arterial disease.1 Percutaneous vascular interventions are rapidly emerging as a less invasive therapy for symptomatic lower extremity occlusive arterial disease.2,3,4 Despite recent advances, restenosis remains a significant problem with catheter-based revascularization. By delivering high local doses, drug-eluting stents have the potential to overcome the limitations of restenosis and advance stent-based therapies for lower extremity peripheral arterial disease (Table 39-1).
There is interest in the applicability of drug eluting stent (DES) in other arterial beds as well. Most studies and case series have focused on the efficacy in the renal arteries, intracranial circulation, and extracranial carotid or vertebral arteries. To date, the bulk of clinical studies have been in the evaluation of drug-eluting stents to reduce restenosis in the coronary arteries, with dramatic results, which are simultaneously changing the treatment paradigm for multivessel coronary artery disease and raising long-term safety concerns.
Balloon angioplasty of occlusive arterial disease results in a lumen diameter gain through several mechanisms. Angioplasty compresses the atherosclerotic plaque, shifting it laterally and axially, as well as causing a “controlled” intimal and medial dissection.5 Angioplasty is, however, limited by the acute elastic recoil properties of the arterial wall with up to a 40% loss in lumen area.5,6 Flow-limiting dissections can occur and may lead to acute or subacute vessel closure.7 As a scaffold, stents improve the initial results of percutaneous interventions by increasing the arterial lumen and tacking up intimal flaps between the stent and the vessel wall. The scaffolding properties of stents virtually eliminate acute arterial recoil and reduce the subsequent risk of acute or subacute vessel closure.5,7,8,9
Restenosis is characterized by three processes: acute vessel recoil, late vessel remodeling (negative/constrictive remodeling), and neointimal hyperplasia.5,7,10 Stents effectively eliminate acute vessel recoil and negative vessel remodeling. In-stent restenosis is, therefore, almost entirely owing to neointimal hyperplasia.8,11,12 Compared to balloon angioplasty, stents can cause more tissue proliferation by virtue of greater chronic injury to the vessel wall. The long-term effectiveness of stents is mitigated by this “late loss” of the arterial lumen because of tissue proliferation within the stent.
The pathophysiology of vascular repair mechanisms after angioplasty and stenting has been extensively studied in a variety of experimental models. Angioplasty and stenting cause local vascular injury, including disruption of the intima, denudation of the endothelium, and penetration of the media.6,7,8 Disruption of the endothelium and exposure of the contents of the subintimal space such as collagen, von Willebrand factor, and the lipid core result in vessel inflammation, the activation of platelets, and the release of growth factors, oxygen-derived free radicals, cytokines, adhesion molecules, and macrophage chemotactic factors.5,6,7,13 Fibrin deposition and thrombus formation at the injury site can provide a matrix for cellular migration and proliferation.6 The degranulation of platelets and stimulation of inflammatory cells trigger the release of mitogens and further cellular activation and proliferation.8,11,14,15 Subsequently, there is the migration and proliferation of vascular smooth muscle cells and fibroblasts into the area of injury. The final component of the tissue response to injury is extracellular matrix deposition of collagen and proteoglycan.7,8 The neointima of in-stent restenosis has a limited cellularity, primarily vascular smooth muscle cells, and is mostly composed of collagen, proteoglycans, and extracellular material.6,8,13
The time course of the vascular proliferative response following stenting has been well established in experimental restenosis models and has been confirmed in human atherosclerotic lesions. Earlier, following stent implantation, there is deposition of platelets, fibrin, and acute inflammatory cells around the stent struts. Within a few weeks after stent implantation, the stent is covered by an immature neointima consisting of macrophages and alpha-actin negative spindle cells. This is followed by smooth muscle cell differentiation and proliferation and extracellular matrix formation. The final step in the healing process is re-endothelialization, which occurs within several weeks to a couple of months following stent implantation.
Current pharmacologic approaches to inhibit restenosis target key steps in the cell cycle (FIGURE 39-1). The cell cycle describes the sequence of events that regulate cellular growth and ultimately cell division. During Gap 1 (G1) the cell is regulated by external stimuli with gene expression and protein synthesis in preparation for the synthesis (S) phase. The nuclear content doubles during the S phase. The Gap 2 (G2) is characterized by increased protein synthesis and cellular growth. G2 phase further prepares the cell for mitosis (M phase). During mitosis, the cell undergoes cytokinesis (producing two daughter cells). The daughter cells can then enter a quiescent phase (G0) or enter G1.
Regulatory pathways of the cell cycle are complex and still being elucidated. Cyclin and cyclin-dependent kinases are involved in the regulation of cellular progression through the cell cycle. p53, a tumor suppressor, inhibits cell-cycle progression if DNA is damaged (or induces apoptosis if there is severe DNA damage). p27 binds cyclin and cyclin-dependent kinase, blocking progression to the S phase of the cell cycle. Phosphorylation of the retinoblastoma protein (pRb) is involved in the regulation of the cell entering the S phase and, subsequently, the G2 phase.
In order to have maximal vascular inhibitory effects on restenosis, yet minimize injury and delay of re-endothelialization, it is necessary to determine the optimal elution kinetics for each candidate molecule. This requires an understanding of the therapeutic window for each molecule, the optimal inhibitory concentration, rate and duration of release, as well as polymer interactions.
To establish a predictable release of drugs, polymer coatings are utilized as a reservoir for controlled elution. With the exception of some compounds, such as paclitaxel, which are lipophilic and can be dip coated onto stents, a polymeric coating is necessary.15 In order for drug-eluting stents to be successful, the polymeric vehicle must not induce inflammation and subsequent in-stent restenosis. Several biodegradable and nonbiodegradable polymers have been tested as candidate vehicles for drug-eluting stents. Van der Giessen et al.16 demonstrated that a variety of biodegradable polymers (polyglycolic acid/polylactic acid, polycaprolactone, polyhydroxybutyrate valerate, polyorthoester, and polyethyleneoxide/polybutylene terephthalate) and nonbiodegradable polymers (polyurethane, silicone, and polyethylene terephthalate) are not inert and can induce extensive inflammatory responses. Several other polymers have been tested, including chondroitin sulfate A/gelatin, polyacrylate plastic sleeves, and polyethylenevinylacetate/polybutylmethacrylate, with varying success.7,17,18
Clinical studies of paclitaxel-coated stents in coronary arteries have evaluated nonpolymer bonding and a variety of polymers and elution kinetics. Park et al.19 evaluated the efficacy of two doses of non-polymer-based paclitaxel elution for coronary stenting. They found that in-stent intimal hyperplasia was reduced in a dose-dependent manner. TAXUS II evaluated two separate polymer-based elution regimens in the coronary arteries. Both formulations were designed to have an initial bolus release for the first 48 hours post implantation and a slower release during the next 10 days. The TAXUS-SR (slow release) stent had eight times less drug released than the TAXUS-MR (moderate release) stent. Six-month angiography revealed significantly less in-stent restenosis in both TAXUS-SR and TAXUS-MR arms (5.5% and 8.6%, respectively) compared to their respective controls (20.1% and 23.8%).20
Similar elution kinetic studies have been performed on sirolimus-coated stents. Two principal release formulations have been tested for sirolimus—both in the coronary and in the peripheral arteries. A ratio of approximately 30:70 sirolimus to copolymer is utilized. The polymer is a 50:50 combination of polyethylenevinylacetate and polybutylmethacrylate.7 Stents are coated with a basecoat copolymer and sirolimus; in order to achieve a slower elution, a top-coat diffusion barrier is applied. The fast-release formulation allows for drug release in <15 days, whereas the slow-release allows for a ≥28-day release.21 In general, there has been similar results for the slow- and fast-release sirolimus stents, although there may be an advantage in in-stent late loss for the slow-release formulation.22
There is evidence in the coronary literature and emerging data in the periphery to suggest that stent design may have an impact on restenosis (Table 39-2).
Lasers are generally used to cut patterns into either metal tubes or sheets, which are subsequently welded together, to manufacture stents.23 Stents can be broadly classified by the cell design. Open-cell design allows for the cell area to change during flexion, while closed-cell stents maintain a relatively fixed cell area. Each design allows for trade-offs between deliverability, plaque covering, apposition, and access to side branches.24 Stent design also has an impact on local drug delivery. Hwang et al.25 demonstrated that the local tissue concentration of eluted drugs is dependent, in part, on strut spacing—more so for hydrophilic than for hydrophobic drugs. Inhomogeneity in strut spacing may also be significant for drugs with a narrow therapeutic window.24
The ISAR-STEREO trial evaluated the effect of stent strut thickness on angiographic restenosis in coronary arteries. Patients were randomized to receive the ACS RX Multi-Link stent (Guidant/Advanced Cardiovascular Systems, Santa Clara, CA) with a strut thickness of either 50 or 140 μm. The primary end point of angiographic restenosis ≥50% was seen in 15% of those receiving the thin strut and in 25.8% receiving the thick strut stent, a 42% risk reduction.26 A follow-up study (ISAR-STEREO-2) compared the ACS RX Multi-Link stent with a strut thickness of 50 μm and a strut width of 100 μm to the BX Velocity (Cordis Corporation, Miami, FL) with a strut thickness of 140 μm and strut width of 130 μm. The ACS RX Multi-Link stent has an interconnected ring design, whereas the BX Velocity is a closed-cell design (both stainless steel). The primary end point of angiographic restenosis ≥50% was seen in 17.9% of the thin-strut and 31.4% of the thick-strut stent—a 43% risk reduction, suggesting that thinner strut thickness, regardless of stent design, results in less angiographic restenosis.27
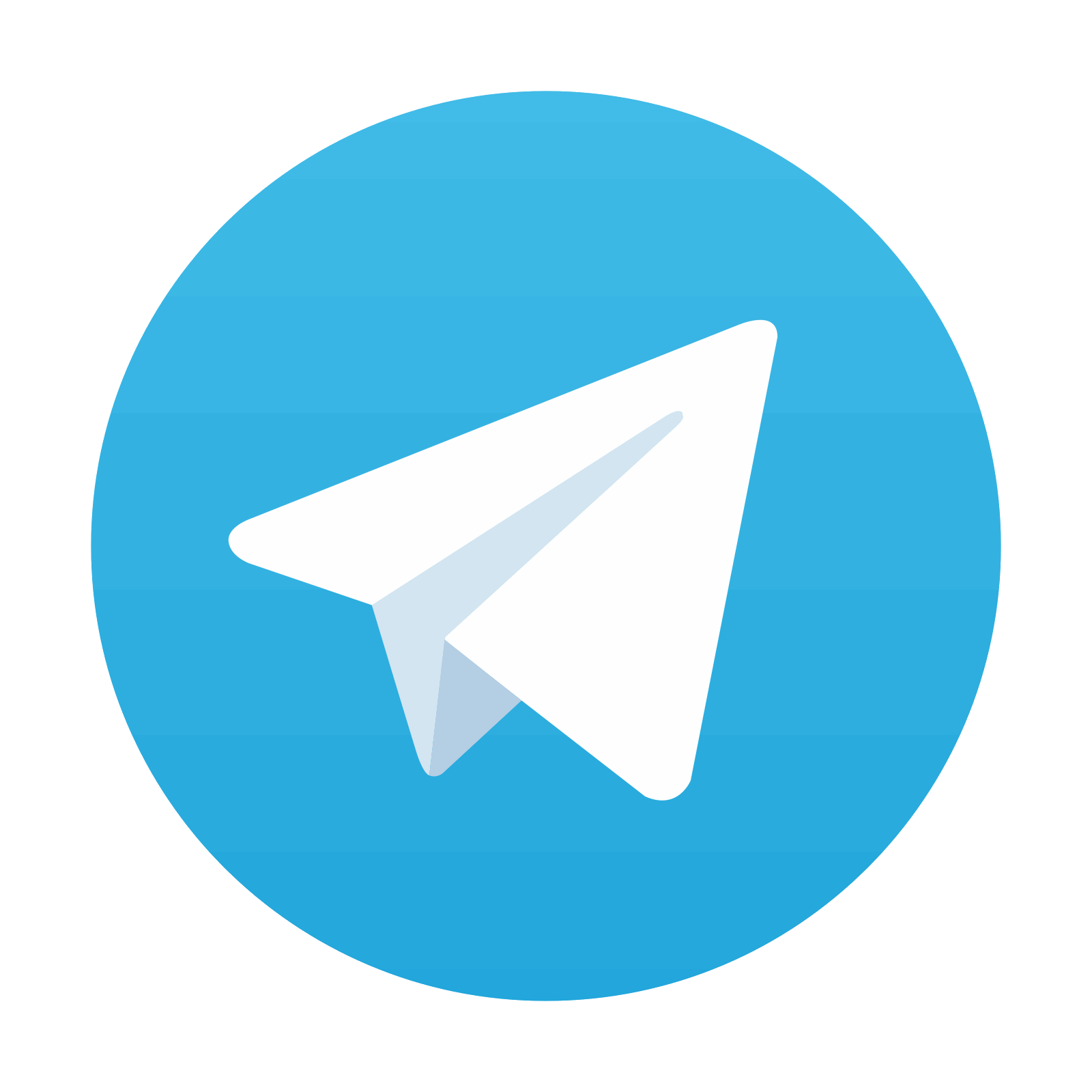
Stay updated, free articles. Join our Telegram channel

Full access? Get Clinical Tree
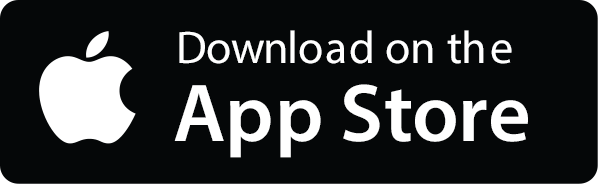
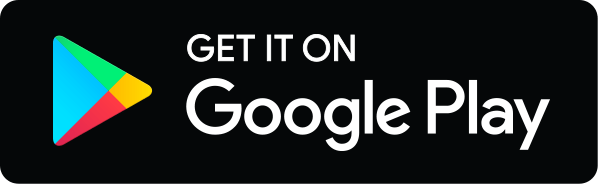