Background
The aims of this study were to establish absolute ranges for right ventricular (RV) structural and functional parameters for endurance athletes and to establish any impact of body size. These data may help differentiate physiologic conditioning from arrhythmogenic RV cardiomyopathy.
Methods
A prospective observational study design was used, and standard two-dimensional echocardiography was performed on 102 endurance athletes, providing RV structural indices. A two-dimensional strain (ϵ) technique was used to provide indices of RV ϵ and strain rate. The association of RV chamber size to body surface area (BSA) and functional indices was examined by simple ratio scaling as well as adoption of the general, nonlinear allometric model.
Results
The values for RV inflow, outflow, length, and diastolic area were greater than published “normal ranges” in 57%, 40%, 69%, and 59% of the population, respectively, while 28% of the population had RV outflow tract values greater than the proposed “major criteria” for arrhythmogenic RV cardiomyopathy. Simple ratio scaling for all RV dimensions to BSA did not produce size independence, whereas scaling for BSA allometrically did. Strain and strain rate values were consistent with published normal ranges, and there is no evidence to suggest that scaling is required.
Conclusions
RV chamber dimensions are larger in endurance athletes than those described by “normal ranges” and frequently meet the major criteria for the diagnosis of arrhythmogenic RV cardiomyopathy. Functional assessment of RV ϵ may aid in this differential diagnosis. RV size is allometrically related to BSA and therefore scaling for population-specific b exponents is encouraged.
It is well established that participation in long-term endurance exercise is associated with cardiac adaptation, including hypertrophy and dilatation of the left ventricle, which can complicate the differential diagnosis of athlete’s heart from those of both hypertrophic and dilated cardiomyopathy. The impact of physiologic conditioning on right ventricular (RV) structure and function, and a similar diagnostic challenge with arrhythmogenic RV cardiomyopathy (ARVC), has received considerably less attention. It is thought that ARVC is implicated in 4% to 21% of all cardiac sudden deaths in the young (aged < 35 years) athletic population. Furthermore, an ARVC phenotype without genetic cause has been observed in a number of endurance athletes, raising the possibility that forms of ARVC may be acquired through long-term intensive endurance exercise.
Echocardiography plays a vital role in the cardiovascular evaluation of athletes, in particular in differentiating between ARVC and normal physiologic RV enlargement. However, data for RV dimensions in athletes are scant, with even less available information relating to RV function in this group. This is likely due to inherent difficulties in providing accurate structural and functional indices because of complex RV geometry and excess trabeculation. Despite these obstacles, the American Society of Echocardiography (ASE) published normal ranges for RV dimensions in 2005, which were adopted by professional bodies such as the British Society of Echocardiography. A subsequent ASE guideline report provided a more comprehensive and substantial range of RV dimension values including myocardial deformation parameters to facilitate the identification of RV pathology, but these data were obtained from the sedentary population. More recently, studies in athletes, albeit in small cohorts, have demonstrated that some athletes exhibit large RV dimensions. Myocardial deformation studies within these groups have attempted to establish parameters that may readily differentiate cardiac physiology from ARVC in athletes with enlarged RV dimensions. Although the relationship of cardiac morphology to body size is well established, neither the ASE nor the British Society of Echocardiography guidelines provide ranges for RV data indexed to body surface area (BSA). Therefore, the exact nature of how RV morphology data relate to body size has not been studied previously. Similarly the relationship between RV size and myocardial deformation remains to be elucidated. In view of the importance of RV size and function in the echocardiographic assessment of endurance athletes in providing clear normative data and thus differentiating from ARVC, the primary aims of this study were threefold: (1) to provide a range of absolute values for RV dimensions in 102 endurance athletes and to assess the nature of the relationships between these variables and measures of body size, (2) to provide a range of data for global RV strain (ϵ) and strain rate (SR) indices and to establish the nature of any relationships between indices of RV morphology and deformation, and (3) to establish if any increase in RV size is consistent with increases in left ventricular (LV) and left atrial (LA) size and, furthermore, whether the left-sided chambers require scaling by the same factors and methods that are applicable to RV assessment.
Methods
One hundred two endurance athletes (86 men, 16 women) with a broad age range (mean, 36 ± 11 years; range, 21–71 years) volunteered to participate in the study. We recruited elite, internationally competitive athletes via direct contact with athletes, coaches, and race organizers. All athletes were competing at the highest level within their chosen sports, including internationally renowned competitions. Height and body mass were measured using standard equipment; the mean height was 178 ± 8 cm (range, 156–193 cm), and the mean body mass was 71 ± 10 kg (range, 44–100 kg). BSA was calculated using the equation of Dubois and Dubois : {[(height) 0.425 × (body mass) 0.725 × 71.84]/1,000}. The mean BSA was 2.02 ± 0.24 m 2 (range, 1.36–2.68 m 2 ). The mean heart rate was 55 ± 10 beats/min (range, 34–89 beats/min). All subjects were healthy and free from known cardiovascular disease and any early family history of cardiovascular disease and were not currently taking any form of prescribed medication. All athletes had no subjective evidence of ARVC, such as saccular outpouching or prominent moderator bands. All subjects were either endurance runners ( n = 73) or endurance cyclists ( n = 29) and were scanned during a period of “peak” conditioning. We adopted a definition of “endurance athlete” on the basis of training and competition history that clearly reflected prolonged but intermittent periods of cardiovascular work. Moreover, training must have been continuous for ≥2 years before this assessment, and the mean number of training hours per week over this period ranged from 8 to 24. The population was purposely heterogenic, with a wide age and body size range. All subjects provided written informed consent to participate, and the studies were granted ethics approval by the Ethics Committee of Liverpool John Moores University.
Echocardiographic Assessments
After a full explanation of procedures, participants lay supine for 5 min before the echocardiographic examination. Heart rate was taken from the electrocardiograph inherent to the ultrasound system. All echocardiographic images were acquired using a commercially available ultrasound system (Vivid I or Vivid 7; GE Vingmed Ultrasound AS, Horton, Norway) with a 1.5-MHz to 4-MHz phased-array transducer. All images from all individual studies were acquired by the same single experienced sonographer using the same standard protocol, with the subject lying in the left lateral decubitus position. Images were recorded to DVD in a raw Digital Imaging and Communications in Medicine format. Offline analysis was performed by the same sonographer using commercially available software (EchoPAC version 7.0; GE Vingmed Ultrasound AS), and a minimum of three cardiac cycles were averaged for all indices.
Standard two-dimensional echocardiographic parameters were obtained from parasternal and modified apical acoustic windows. All settings were optimized to obtain maximum signal-to-noise ratio and two-dimensional images to provide optimal endocardial delineation. In accordance with ASE guidelines, RV size was measured at end-diastole from the proximal RV outflow tract (RVOT) using a parasternal short-axis orientation at the level of the aortic valve and at the basal RV inflow (RVI) from a modified apical four-chamber orientation (see Figure 1 ). RV length (RVL) was also measured from the RV apex to the tricuspid annulus. RV end-diastolic area (RVDarea) and end-systolic area were calculated by tracing around the endocardium from a modified apical four-chamber orientation, and RV fractional area change was calculated.

Color-coded Doppler tissue imaging was used to assess RV myocardial velocities. Standard color Doppler tissue imaging acquisition was undertaken as previously described, with a temporal resolution > 150 frames/sec. Offline analysis allowed a 6 × 6 mm sample volume to be placed in the tricuspid annulus of the RV lateral wall, and peak velocities in systole (S′) and early diastole (E′) were measured.
Conventional two-dimensional assessments of LV and LA structure were made in accordance with ASE guidelines. LV mass, LV end-diastolic volume (LVEDV), and LV end-diastolic diameter (LVd) were calculated for scaling purposes, while LV end-systolic volume was also calculated using Simpson’s biplane methodology to enable the calculation of LV ejection fraction. LA size was presented as the standard anterior-posterior dimension (LAd), measured using a standard parasternal long-axis orientation, while LA volume at end-systole was measured using apical four-chamber and two-chamber orientations and Simpson’s biplane methodology. To establish the extent of physiologic adaptation of the right ventricle relative to LV remodeling, the ratio of RVI to LV dimension in the apical four-chamber orientation was calculated.
Two-Dimensional Myocardial Speckle Tracking
For the acquisition of RV functional data, the apical four-chamber orientation was used and frame rates were acquired as high as possible, but no greater than 90 frames/sec, with the focal point positioned at the midlevel of the RV cavity to minimize the impact of beam divergence. All images were optimized with gain, compression, and dynamic range to enhance myocardial definition, while depth, frequency, and insonation angle were standardized from all acquisitions to reduce the impact of variability. For offline analysis, the region of interest was placed around the RV lateral wall from base to apex. Global peak values were obtained as the average of the base, mid, and apical wall segments. This was considered important in that there is some evidence to suggest a basal-to-apical gradient in the athletic heart. Indices obtained included peak RV ϵ and SR during ventricular systole (SRS′) and during early and late ventricular diastole (SRE′ and SRA′) and their ratio.
A further 20 subjects with two separate acquisitions were analyzed for RV intraobserver variation. Unlike other studies, this was achieved using a within-participant, within-day design to ensure the “best case” reliability on acquisitions rather than reliability of a single measurement. Only intraobserver variation was assessed, given that this study used a single operator for both acquisition and measurement, allowing meaningful interpretation of the data.
Data Analysis and Statistics
We provide mean ± SD and range data for all absolute values of RV, LV, and LA dimensions. Initially, the dimensional data were scaled for individual differences in BSA using a ratio-scaling approach ( y / x ). The aim was to facilitate comparisons with past data. On the basis of theoretical and empirical limitations of ratio scaling, we checked if this scaling approach removed the influence of body size using a simple bivariate correlation approach ( y / x : x ). Where ratio scaling failed to remove the influence of body size, we subsequently investigated the nature of allometric relationships between BSA and RV, LV, and LA dimensions and determined if allometric scaling for BSA provided size-independent dimensional indices.
The mean ± SD and range data are also provided for all absolute values of RV ϵ and SR. Scaling of such data has not been previously assessed, so we determined the nature of the relationships between RV ϵ and individual differences in RV morphology using allometry. Allometric scaling of the general form y = a · x b was used. All allometric regression model parameters were solved by working in the arithmetic space defined by the original, raw x and y variables using an iterative, nonlinear protocol (Levenberg-Marquardt algorithm). In this procedure, small successive corrections to parameter estimates are made until a global solution converges. Size exponents ( b ) together with their 95% confidence intervals [CIs] were calculated and are presented along with the nonlinear correlation coefficient for the model. We define the smallest worthwhile effect for the allometric relationships as a correlation coefficient of r = 0.30, a moderate effect size in Cohen’s terms. Analyses were conducted using SPSS version 16.0 (SPSS, Inc., Chicago IL).
To assess the impact of age on chamber size independent of body size, a covariate analysis was undertake using the model y = a : x b × exp( c × age). The subsequent b was compared with that obtained using the general model y = a · x b . Bivariate Pearson’s correlations were also used to assess any relationship between absolute structural values and age and also whether there was any association between right-sided and left-sided enlargement.
Results
RV Morphology and Function
Intraobserver variability of RV indices is expressed using the coefficient of variation, with values for ϵ, SRS′, SRE′, and SRA′ of 7%, 13%, 17%, and 15% respectively. RVOT, RVI, RVL, and RVDarea all provided excellent intraobserver reproducibility, with values of 2%, 1%, 4%, and 4%, respectively.
All RV structural data are presented in Table 1 . All 102 athletes had adequate image quality to allow a complete set of RV measurements. The values for RVI ranged from 30 to 55 mm, with 57% of the population having RV diameters greater than the considered normal range (see Figure 2 ). Proximal RVOT ranged from 26 to 49 mm, with 40% of the population having values above the ASE’s normal range (see Figure 3 ). Furthermore, 28% of the population had values greater than the proposed “major criteria” for ARVC. RVL ranged from 70 to 110 mm and RVDarea from 13 to 38 cm 2 , with values falling above ASE cutoffs in 69% and 59% of the population, respectively. When indexed (ratio scaling) for BSA, proximal RVOT ranged from 13 to 25 mm/m 2 , with 6% of the population meeting major criteria for ARVC.
Parameter | Mean ± SD (range) | ASE normal value ∗ | Indexed ratio scaling: BSA, mean ±SD (range) | Size independence | Indexed allometric scaling: BSA, mean ±SD (range) | Size independence |
---|---|---|---|---|---|---|
RVOT (mm) | 34 ± 5 (26–49) | <35 | 17 ± 3 (13–25) mm/m 2 | No | 28 ± 4 (21–39) mm/(m 2 ) 0.311 | Yes |
RVI (mm) | 44 ± 5 (30–55) | <42 | 22 ± 3 (15–30) mm/m 2 | No | 32 ± 4 (21–39) mm/(m 2 ) 0.468 | Yes |
RVL (mm) | 92 ± 9 (70–110) | <86 | 45 ± 5 (32–61) mm/m 2 | No | 70 ± 6 (56–86) mm/(m 2 ) 0.366 | Yes |
RVDarea (cm ) | 26 ± 5 (13–38) | <25 | 13 ± 2 (9–18) cm 2 /m 2 | No | 14 ± 2 (10–19) cm 2 /(m 2 ) 0.880 | Yes |


All RV dimensions were modestly but significantly correlated with BSA (RVOT, r = 0.267; RVI, r = 0.458; RVL, r = 0.434; and RVDarea, r = 0.573; all P values < .001). This prompted the need to scale these data, and common clinical practice is to accomplish this by ratio indexing for BSA. This process, however, did not produce size-independent parameters ( Table 1 ). Specifically, the bivariate correlations ( y / x : x ) were also significant for all RV dimensions (RVOT, r = 0.657; RVI, r = 0.512; RVL, r = 0.378; and RVDarea, r = 0.786; all P values < .001). Subsequent allometric analysis of the relationships between RV dimensions and BSA revealed diverse b exponents and CIs (RVOT, b = .311 [95% CI, 0.076–0.545], r = 0.272; RVI, b = 0.468 [95% CI, 0.275–0.661], r = 0.460; RVL, b = 0.366 [95% CI, 0.209–0.524], r = 0.448; and RVDarea, b = 0.880 [95% CI, 0.604–1.156], r = 0.570). The use of these data in y / x b scaling did produce size-independent indices for all dimensions (RVOT, r = 0.03, P = .980; RVI, r = 0.05, P = .961; RVL, r = 0.012, P = .906; and RVDarea, r = 0.048, P = .651). Figure 4 is an exemplar scatterplot of RVOT dimensions, demonstrating the standard ratio-scaling linear relationship and the more accurate allometric relationship y = a : x b .

Adequate speckle tracking was achieved in all segments in all 102 subjects. Peak RV ϵ ranged from −18% to −41% and peak RV SRS′ from −0.75 to −2.65 sec −1 , consistent with normal ranges proposed by the ASE (see Table 2 ). RV diastolic deformation indices displayed marked individual variability, with a dominant SRE′ (mean, 2.0 ± 0.61 sec −1 ; range, 0.87–3.76 sec −1 ) and smaller SRA′ (mean, 1.25 ± 0.56 sec −1 ; range, 0.28–2.88 sec −1 ), hence providing a mean SRE′/SRA′ ratio of 1.8 ± 1.03.
Parameter | Mean ± SD (range) | ASE normal value ∗ |
---|---|---|
RV ϵ (%) | −27 ± 6 (−18 to −41) | −18 to −39 |
RV SRS′ (sec −1 ) | −1.53 ± 0.43 (−0.75 to −2.63) | −0.7 to −2.54 |
RV SRE′ (sec −1 ) | 2.00 ± 0.61 (0.87 to 3.76) | NA |
RV SRA′ (sec −1 ) | 1.25 ± 0.56 (0.28 to 2.88) | NA |
RV FAC (%) | 47 ± 7 (35 to 61) | 35 to 63 |
RV S′ (cm/sec) | 11 ± 1.3 (7 to 14) | >6 |
RV E′ (cm/sec) | −10 ± 2.1 (−6 to −17) | NA |
The allometric relationships between RV ϵ data and RV morphology dimensions were generally small (all r values < 0.168) and nonsignificant ( b -value CIs straddled zero). The lack of any relationship between peak RV ϵ as well as RV SRE′ with any RV morphologic parameter would suggest that absolute values are themselves size independent and do not require scaling. For RV SRS′ on RVL scaled significantly, but this relationship was still weak (low r value and broad CIs), and thus, promotion of scaling on this basis would be tenuous. RV SRA′ scaled significantly with RVI, RVL, and RVDarea, but again, the relationships were weak; however, it is clear that further analysis of these relationships may be warranted.
RV fractional area change ranged from 35% to 61%, with only two subjects having values lower than the proposed normal range, while mean myocardial velocities were all within normal limits (S′, 7 to 14cm/sec; E′, −6 to −17cm/sec) (see Table 2 ).
LV and LA Morphology
All LV and LA structural data are presented in Table 3 . LV ejection fraction was within normal limits (mean, 63 ± 5%; range, 55%–75%). The values for LV mass index ranged from 62 to 166 g/m 2 , for LVd from 20 to 35 mm/m 2 , and for LVEDV from 44 to 98 mL/mm 2 , meaning that for these parameters, 36%, 6%, and 31%, respectively, had values greater than considered normal. The values for LA morphology were generally larger than the normal population, with LAd ranging from 14 to 25 mm/m 2 and LA volume at end-systole from 20 to 58 mL/m 2 , making 6% and 88%, respectively, of the population above the normal ranges proposed by the ASE ( Figure 5 ).
Parameter | Mean ± SD (range) | ASE normal values ∗ | Indexed ratio scaling: BSA, mean ± SD (range) | ASE normal values | Size independence | Indexed allometric scaling: BSA, mean ± SD (range) | Size independence |
---|---|---|---|---|---|---|---|
LVd (mm) | 52 ± 5 (42–62) | Female <53, male <59 | 27 ± 3 (20–35) mm/m 2 | Female <32 mm/m 2 , male <31 mm/m 2 | No | 42 ± 3 (35–49) mm/(m 2 ) 0.324 | Yes |
LV mass (g) | 221 ± 41 (118–377) | Female <162, male <224 | 110 ± 22 (62–166) mm/m 2 | Female <95 g/mm 2 , male < 115 mL/mm 2 | Yes | NA | NA |
LVEDV (mL) | 137 ± 31 (60–218) | Female <104, male <155 | 69 ± 12 (44–98) mm/m 2 | Female <75 mL/m 2 , male <75 mL/m 2 | Yes | NA | NA |
LAd (mm) | 40 ± 4 (29–54) | Female <38, male <40 | 20 ± 4 (14–25) mm/m 2 | Female <23 mm/m 2 , male <23 mm/m 2 | No | 31 ± 3 (24–40) mm/m 0.38 | Yes |
LA volume at end-systole (mL) | 65 ± 17 (37–111) | Female <52, male <58 | 32 ± 8 (20–58) mL/m 2 | Female <29 mL/m 2 , male <29 mL/m 2 | Yes | NA | NA |
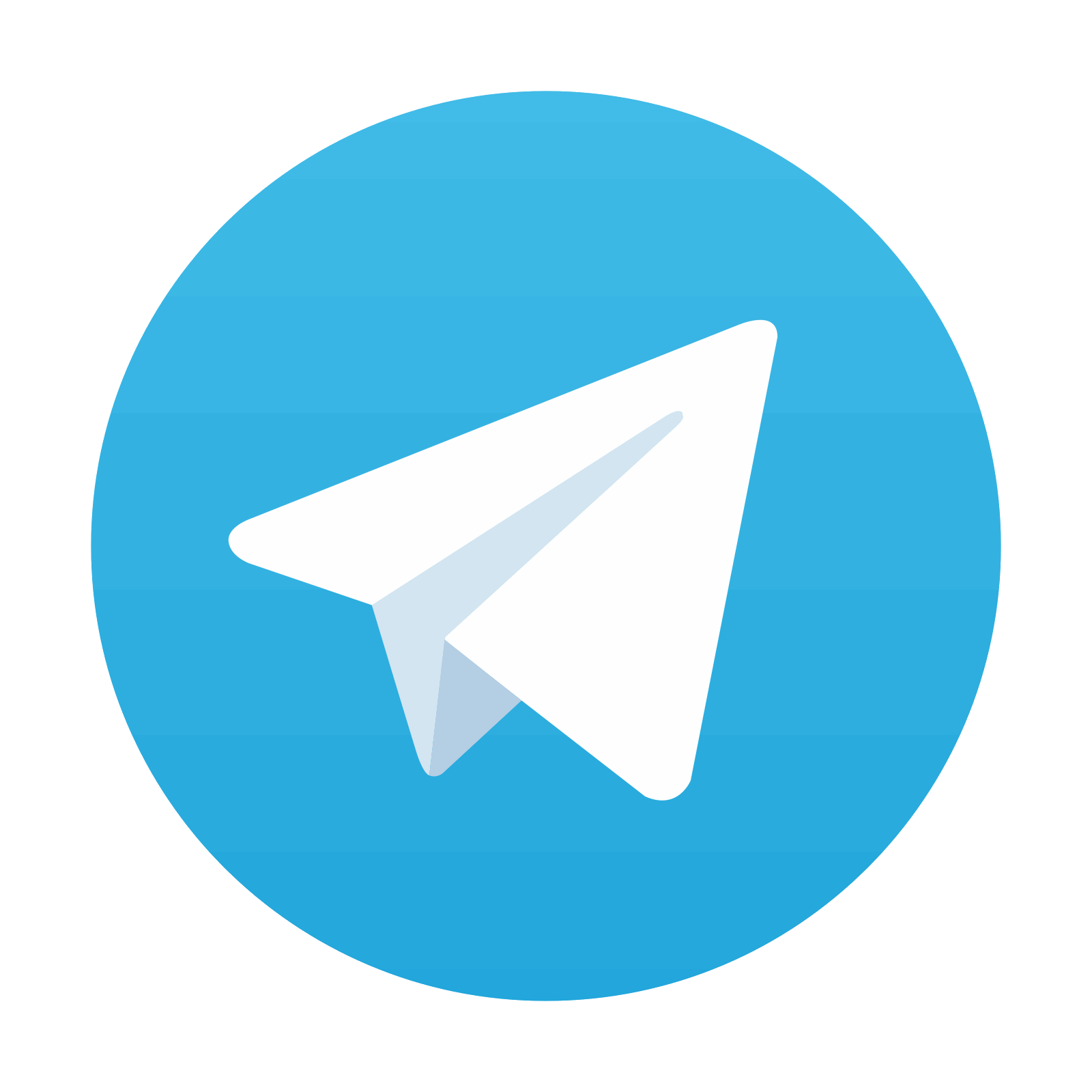
Stay updated, free articles. Join our Telegram channel

Full access? Get Clinical Tree
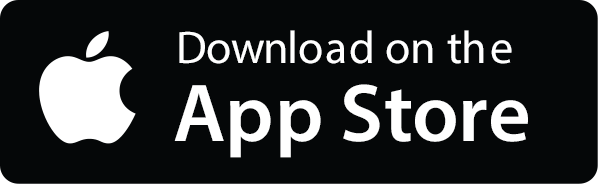
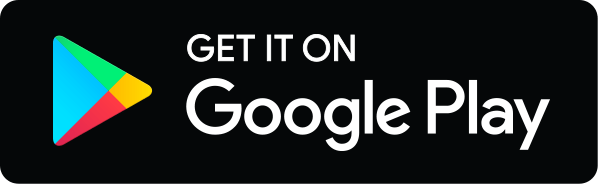
