Fig. 11.1
Prevalence of hypertension , coronary artery disease, congestive heart failure, and stroke with aging (Based on data from Mozaffarian et al. [1])
Not only does the aging process contribute to the development of CV disease, aging appears to worsen the outcome of disease. For example, elderly patients are not only more likely to experience myocardial infarction, but they are also more likely to develop heart failure as a consequence of a myocardial infarction than their younger counterparts [3–5]. Furthermore, elderly patients are also more likely to die from their myocardial infarction, develop cardiac arrest and papillary muscle rupture, and acquire ventricular septal defect and free wall rupture [3].
Assigning a high ASA physical status number to an elderly patient is often a result of the presence of coexisting cardiovascular diseases, but it is often the underlying physiological adaptations to CV disease that impact decisions with anesthesia delivery. This impact of CV disease devalues the importance of the chronological age of an individual and emphasizes the functional aging process in determining the physical status. The functional aging process has known variability because the CV system is constantly adapting to short-term and long-term influences. Assessment of functional age requires a focused history to better understand a patient’s activity level and abilities to manage CV demand such as walking, climbing stairs, or more advanced endurance activities. In the process, a composite picture of adaptation to age-related effects on interdependent variables of heart rate, coronary blood flow, afterload or impedance, preload or diastolic filling, and inotropic state is obtained. All show relative degrees of age-dependent changes. Modulation of these factors is in part via the autonomic nervous system (ANS) acting through both sympathetic and parasympathetic control mechanisms. Age-related changes of the ANS further contribute to modifying cardiovascular function and adaptation to stress in the elderly. The sum of all changes typically results in a reduction in the overall cardiovascular reserve with age. In order to produce the best possible patient outcome, the anesthesiologist must demonstrate both knowledge and skill when managing the autonomic responses to surgical pain and intravascular volume changes, the functional status of individual components of the CV system, and their interdependence.
This chapter addresses predominantly the physiological and pathophysiological effects of aging on the cardiovascular system. It is difficult to clearly differentiate the aging process from age-related diseases. In a particular patient, both processes interact to yield the specific physiological state of the system. In this chapter, the age-related changes in the cardiovascular system will be discussed first. The general morphologic changes induced in both the vasculature and the heart are similar with stiffening, thickening, dilatation or enlargement, and endothelial or myocardial dysfunction as common themes. The vascular system is closely coupled to the ventricles, and the progressive changes in the vasculature lead to compensatory changes in the cardiac function. The cardiac conduction system and the cardiac valves also degenerate over time. Changes in the autonomic regulation and the neuroendocrine system, which occur with aging, and their impact on the cardiovascular system, will be then reviewed. Finally, fluid management and general principles of cardiovascular management in the elderly will be discussed.
Cardiac and Vascular Morphologic Changes with Aging
As the human body ages, it undergoes a variety of changes, some are relatively benign although some can have major influences to impair the overall health of the aging person. An example of such a detrimental digression accompanying increasing age is the increased stiffness of the heart and vascular tree.
Vascular stiffness is the result of increased collagen, decreased elastin, glycosylation of proteins, free radical damage, calcification, and chronic mechanical stress (also described as “fatigue failure”). The concept of “fatigue failure” is extrapolated from the effect of that observed in rubber tubing subjected to repetitive stretch/relaxation cycling [6]. Aging can radically transform the endothelial layers via changes in extracellular matrix compositions. Elasticity in connective tissues depends primarily on the properties of its constituent collagen and elastin. Both connective tissue proteins are long-lived but slow in their production. By the age of 25, production of elastin has essentially ceased, and the rate of turnover of collagen decreases with increasing age. With aging, the elastic lamellae undergo thinning and fragmentation [7] with gradual transfer of mechanical forces to collagen. The consequent increase in the collagen-to-elastin ratio, plus an accumulating damage to collagen by glycation and free radicals, results in progressive connective tissue stiffness. Thus, the arteries, veins, and myocardium become less compliant over time.
Nonenzymatic glycation is a reaction between reducing sugars and proteins on the vascular endothelium. Over time, these glycation sites cause tight cross-linking of proteins called advanced glycation end products (AGE) . This AGE formation leads to changes in the physiochemical properties of endothelial tissues. AGE cross-linking structurally results in vessels with less elasticity and compliance [8]. Furthermore, the interaction of AGE with receptors for AGE (RAGE) on endothelial cells has been implicated as an initiating event in atherogenesis. In smooth muscle cells, binding of AGE-modified proteins to RAGE is associated with increased cellular proliferation of smooth muscle cells. This interaction also causes an increase in vascular cell adhesion molecule-1, which enhances binding of macrophages to the endothelial surface. This induces oxidative stress on the vascular endothelium and contributes to vascular stiffness [9].
Another factor that contributes to vascular stiffening with aging is progressive vascular calcification. This is a complicated process whereby in certain disease states, vascular smooth muscles cells, pericytes, and endothelial cells change their phenotypes to mesenchymal cells, osteoblasts, and chondrocytes [10]. All these processes can then lead to increased calcium deposition in the vasculature and cause vascular stiffness [10].
Vessels thicken with age, primarily from intimal thickening that is attributed to increases in collagen, fibronectin, proteoglycans, and migrating smooth muscle cells [11]. These changes are stimulated by TGF-β 1, angiotensin-II, and decreased levels of inhibitory cytokines and degrading enzymes [12]. ACE inhibitors produce beneficial effects by reducing connective tissue remodeling, smooth muscle hypertrophy, and arterial stiffness.
Several studies have shown that the nitric oxide pathway becomes less functional with age. This has implications on vascular compliance. Nitric oxide suppresses key events in atherosclerotic development such as vascular smooth muscle proliferation and migration. It also inhibits the adhesion of monocytes and leukocytes in the endothelium, as well as platelet-vessel interaction. Furthermore, nitric oxide is known to regulate endothelial permeability, reducing the flux of lipoproteins into the vessel wall [13]. The reduced effects of nitric oxide on all of these pathways may contribute to vascular stiffness in aging.
Another mediator that may contribute to endothelial dysfunction is endothelin-1 . Endothelin-1 is 50 times more potent at vasoconstriction than norepinephrine [14]. Though the endothelin-I expression is variable in different vascular beds, increased levels of endothelin-1 have been noted with aging and may be responsible for the glomerulosclerosis that is observed in aging kidneys [15, 16]. Endothelin-1 levels are positively associated with aging and account for increased endothelin-1-mediated vasoconstriction in older people [17, 18].
Increased expression of prostanoid vasoconstrictor proteins, altered cyclooxygenase, and prostaglandin H synthase activities [19] develop with aging. In contrast, vascular endothelial growth factor (VEGF) and hypoxia-induced factor (HIF) are reduced with aging. Endothelial dysfunction leads to an attenuated vasodilator responses in skin microvasculture [20, 21] and contributes to microvascular dysfunction of the skin. The latter may predispose the elderly to impaired wound healing [22].
Atherosclerosis and arteriosclerosis are inflammatory processes. Increased levels of C-reactive protein and increases in erythrocyte sedimentation rate suggest an increased inflammatory propensity in the elderly [10]. Some have coined the condition as “inflammaging,” and the process is thought to be due to upregulation of a range of proinflammatory cytokines [23, 24]. However, concurrent immunodeficiency has also been noted in the elderly that makes them prone to infection and immune-mediated diseases. It is not unreasonable to assume that the inflammatory milieu affects vascular aging.
The above mechanisms serve to explain the pathogenesis of vascular stiffness associated with aging. As the heart is closely coupled to the vascular system, it is important to note that many of the changes to the aging heart are closely linked to progressive changes in the vascular system [25]. The vascular system serves both as a reservoir and a conductive system. It serves a critical role in buffering the effects of intermittent ejection (stroke volume ). In a young person, the aorta and proximal arteries expand 10% with each contraction, whereas the distal muscular arteries expand only 3% [26]. Generalized stiffening of the arterial tree leads to increased arterial wave reflectance, increased systolic blood pressure, decreased diastolic blood pressure, and a widened pulse pressure.
As arterial walls stiffen, blood vessel compliance is reduced, leading to an increase in systolic blood pressure and pulse wave velocity (Fig. 11.2). A number of studies demonstrate a significant association between increased pulse wave velocity and all-cause mortality and adverse cardiovascular events [27]. The reflected waves return earlier to the thoracic aorta, arriving by late ejection instead of early diastole. Thus, the left ventricle must pump against a higher pressure in late ejection than under normal circumstances. This additional afterload places an increased burden on the heart, particularly because it occurs late in systole when the myocardial muscle is normally losing its strength, and therefore provides a significant stimulus for cardiac hypertrophy (Fig. 11.3).



Fig. 11.2
Mean aortic pressure (triangles) and pulse wave velocity (circles) in two Chinese populations: rural Guanzhou (unfilled symbols) and urban Beijing (filled symbols) (Adapted from Avolio et al. [123]. With permission from Wolters Kluwer Health)

Fig. 11.3
Left ventricular (LV) posterior wall thickness (mm/m2) in normotensive men as a function of age (years) (Adapted from Gerstenblith et al. [124]. With permission from Wolters Kluwer Health)
Diastolic Dysfunction
The cardiac muscle hypertrophy that develops secondary to the increased late systolic afterload also leads to myocardial stiffening and diastolic dysfunction. Diastolic dysfunction is defined as impairment in the relaxation phase of the ventricles. The aging heart contains AGE cross-linked collagen, which has the same effect on stiffness as it does in the peripheral vascular system. It is implicated in the signaling of macrophage recruitment in hypertensive myocardial fibrosis that contributes to deteriorating diastolic function [8]. Another consequence of altered extracellular matrix formation is that scar formation and healing are impaired in the elderly making them more prone to severe complications after myocardial infarction [12].
In diastolic dysfunction, there also is a functional component to the impairment of relaxation. It has been proposed that alterations in the myocyte calcium-handling proteins disturb the calcium transient in failing hearts. The rate of calcium uptake in the sarcoplasmic reticulum declines with heart failure because of reduced expression of certain calcium channel enzymes [28]. This contributes to increased duration of contraction and slowed relaxation of the myocardial muscle fibers, and the stiff ventricles have less ability to “spring open” in early diastole [29].
As a consequence, there is a progressive decrease in ventricular filling during early diastole between the ages of 20 and 80. At its worst, the early diastolic filling period is reduced by 50% compared with younger controls. With increased stiffness, there also is a decline in the diastolic filling rate (Fig. 11.4). However, resting end-diastolic volume does not change with increasing age. Because the early ventricular filling is impaired with age, the heart is increasingly dependent on an adequate atrial filling pressure and the atrial contraction (Fig. 11.5). The atrial pressures must rise to maintain the end-diastolic volume in the presence of stiffened ventricles. The increased atrial pressure can result in increased pulmonary blood pressures and ultimately lead to congestion in the systemic venous circulation. The cumulative effect of these alterations results in diastolic dysfunction (Fig. 11.6).




Fig. 11.4
Changes in early diastolic left ventricular filling and the atrial contribution to filling associated with increased age. Age and peak filling rate relationship was obtained at rest (squares) and maximum workload (triangles). Inset: top image = left ventricular filling, young; bottom image = left ventricular filling, advanced age (Adapted from Lakatta [125]. With permission from Elsevier)

Fig. 11.5
Echo-Doppler evaluation of diastolic filling in healthy men and women as a function of age. (a) Early diastolic filling volume (% of total volume). (b) Diastolic filling caused by atrial contraction (% of total volume) (Adapted from Lakatta [126]. With permission from Oxford University Press)

Fig. 11.6
The increased ventricular stiffness associated with age requires an increased atrial pressure to achieve the same end-diastolic volume (Adapted from Dauchot et al. [127]. With permission from Wolters Kluwer Health)
About half of heart failure in the elderly population (older than 75 years) is associated with impaired left ventricular diastolic function , with a relatively preserved left ventricular systolic function [30]. Unfortunately, patients with isolated left ventricular diastolic dysfunction are not as likely to present with the traditional physical manifestations of heart failure. Instead, they are frequently asymptomatic or subtly present with only mild pulmonary congestion, exertional dyspnea, and orthopnea. These symptoms may be aggravated by systemic stressors such as fever, exercise, tachycardia, or anemia. As a result, detection of diastolic heart failure during a history and physical exam may be difficult since it is often recognized only by echocardiography.
Systolic function of the heart also is affected by the aging process. From a functional standpoint, the prolonged myocardial contraction maintains the flow delivered to the stiffened arterial tree, thereby maintaining cardiac output (Fig. 11.7). The functional adaptation to vascular stiffening and afterload is able to maintain cardiac output at rest; however, an age-related decline in systolic function may be unmasked in the presence of exercise or sympathetic stimulation. For example, administration of an α-adrenergic agonist such as phenylephrine will acutely increase afterload to the heart, increasing left ventricular wall stress during systole, and unmasking an age-related decrease in contractile reserve [31].


Fig. 11.7
A cascade of functional adaptations to vascular stiffening in the elderly. LV Left ventricle (Adapted from Lakatta [125]. With permission from Elsevier)
Further studies have shown that there is abnormal systolic function in many patients who have hypertension-induced concentric hypertrophy with a normal ejection fraction. Reduced midwall shortening in relation to stress is clearly evident in patients with greater relative wall thickness. This translates to abnormal pump function and reduced cardiac output. Subtle systolic dysfunction may be present even if patients have seemingly normal ejection fractions and are without clinical heart failure, and it would be incorrect to equate a normal ejection fraction with normal systolic function [32].
Reduced vascular compliance, diastolic dysfunction, and systolic dysfunction in the elderly are all interconnected. It is reasonable to assume that these are not separate pathologies and in fact develop in parallel. Reduced vascular compliance resulting in hypertension, increased afterload, and eventual cardiac remodeling, is an extremely common finding in the aging population. In a large portion of this group, this inevitably results in some evidence of diastolic dysfunction. Furthermore, the above concepts demonstrate that some systolic dysfunction exists in many of these same hypertensive elderly patients.
Other physiological consequences of increased arterial wave reflectance are increased systolic blood pressure, decreased diastolic blood pressure, and a widened pulse pressure (Fig. 11.8) [33, 34]. Data from the Framingham Heart Study shows that systolic blood pressure increases by 5 mmHg per decade until the age 60 and then increases by 10 mmHg per decade, while the diastolic pressure remains the same. This leads to a large difference between systolic and diastolic blood pressure, for example, 80 mm Hg, and is sometimes alluded to as “pulse-pressure hypertension” [32]. Widened pulse pressure is a hallmark of aging and has been associated with poor clinical outcomes.


Fig. 11.8
Directly measured arterial waveforms from a peripheral (radial) artery and calculated aortic pressure waves for a 26-year-old man (upper panels) and his 83-year-old grandfather (lower panels) (Courtesy Michael O’Rourke, MD, University of Sydney, Australia)
A relatively high systolic pressure in comparison to diastolic pressure is harmful for several reasons. First, a high pulse pressure indicates that the patient’s arterial conduit system is stiff. Low compliance means that a high systolic pressure is required in order to distend the aorta and other large arteries as the stroke volume is received. Even though this increase in pressure occurs relatively early in ejection, it still forces the ventricle to pump against a high pressure and stimulates hypertrophy that, in turn, increases myocardial stiffness and further impairs diastolic relaxation. Indeed, there is a strong correlation between the severity of reduced arterial compliance and the severity of diastolic dysfunction [35]. Second, when the diastolic pressure is low compared with systolic pressure, there is an immediate predisposition to an imbalance of myocardial oxygen supply and demand. Demand correlates most closely to systolic pressure [36], whereas coronary blood flow occurs mostly during diastole, making supply highly dependent on diastolic pressure. With rapid transit of reflected arterial waves, there is loss of the accentuated pressure in early diastole. This lowering of aortic pressure during diastole potentially diminishes coronary perfusion. In patients with coronary disease, this imbalance could result in subendocardial ischemia, thereby worsening diastolic relaxation and increasing atrial pressure.
Because of the consequences of arterial stiffening, arterial compliance has been suggested as a better measure of biologic age, as opposed to chronologic age [37]. And it is not surprising that there is great interest in strategies to reduce or even reverse arterial stiffening in the hope of preventing CVD. Current human therapy primarily involves drugs that relax smooth muscle tone. Statins not only inhibit myocardial remodeling but may lessen vascular stiffness. Angiotensin blockers and aldosterone seem to lessen fibrosis, and exercise slows vascular stiffening and remains a useful therapy for all ages.
Neuroendocrine Changes with Aging that Affect the Cardiovascular System
Aging of the neuroendocrine system can have a significant effect on the cardiovascular system. Changes include the number of adrenergic receptors in the cardiac and vascular tissues, attenuation of signal transduction pathways, and changes in the balance between sympathetic and parasympathetic activity. The renin-angiotensin-aldosterone system, vasopressin, and natriuretic peptides are also affected by aging.
Adrenergic Receptor Activity and Aging
Aging has been associated with a decrease in the response to stimulation of β-receptors. This is noted in the peripheral circulation by a reduced arterial and venous dilation response to the β-agonist, isoproterenol, and the mixed agonist, epinephrine, in the elderly. In cardiac muscle, there is a reduction in the inotropic response to exercise and to exogenous catecholamine administration in the aging patient [38]. In isolated cardiac myocytes, it has been shown that the EC50 for isoproterenol (a β1– and β2-agonist) is nearly twice as high in the elderly [39]. As a result of the decreased contractile response to β-adrenergic stimulation in the elderly, there is a greater dependency on the Frank-Starling (length-tension) mechanism of contraction to maintain cardiac output.
Although multiple studies indicate that the heart rate increase to β-stimulation of the heart is attenuated with age (Fig. 11.9), at least one study has questioned the age-related attenuated chronotropic response [38]. Studies also provide conflicting results regarding age-related changes in myocardial β-adrenoceptor density. The mechanism for decreased cardiac inotropic response to sympathetic stimulation is more likely attributable to changes in the second messenger system. Impaired coupling of the β-adrenoceptor to the Gs protein and to the catalytic unit of adenylyl cyclase is consistently observed in the elderly myocardium. Furthermore, an increase in Gi protein levels observed in aged myocardial tissue indicates a reduction in the catalytic subunit of adenyl cyclase [40]. Both of these mechanisms will attenuate 3′,5′-cyclic adenosine monophosphate (cAMP) formation and subsequent β-adrenoceptor response. This desensitization of the intracellular processing of receptor signaling is likely a compensatory adaptation to an increase in endogenous norepinephrine resulting from age-related increases in sympathetic activity and reduced neuronal uptake of norepinephrine. Furthermore, the decrease in proportion of β-1 vs β-2 with aging and in heart failure is implicated as a potential mechanism of decreased responsiveness to β-adrenergic stimulation in the elderly [41]. In addition to β-1 and β-2 adrenergic receptors, cardiac myocytes have β-3 receptors. Cardiac β-3 adrenergic receptors are coupled to cGMP/NO pathway and cause negative inotropic effects, serving thereby as a brake in sympathetic overstimulation which is seen in heart failure [42].


Fig. 11.9
The effect of intravenous isoproterenol infusions on increasing heart rate in healthy young (filled circles) and older (unfilled circles) men at rest (Adapted from Lakatta [125]. With permission from Elsevier)
This attenuated β-adrenoceptor response as a result of changes in second messenger function has implications in the peripheral vascular system. Vasorelaxation is accomplished in vascular smooth muscle cells via cAMP. cAMP activates protein kinase A (PKA) that then lowers cytosolic calcium levels, causing vasorelaxation. Decreased generation of cAMP in the vasculature leads to impairment of this pathway. This may be a contributing factor for hypertension in the elderly. Because cAMP is an antiproliferative agent, this deficiency may be associated with the progression of atherosclerosis [40].
Genetic variation in β-adrenoceptors is documented and has a significant role in CVD heterogeneity among individuals. There are many known polymorphisms of both β1– and β2-adrenoceptor subtypes. These variants may have differing effects on the cardiovascular system with age. The most common polymorphism, whose allele frequency is 60%, causes enhanced down regulation of β2-adrenoceptors. Because peripheral β2-receptors cause vasodilation and a reduction in blood pressure, individuals with this polymorphism are more prone to hypertension with increasing age. This fact has been confirmed in familial studies, which show increased prevalence of this allele in families with a history of essential hypertension. Another β-adrenoceptor polymorphism with important implications in cardiac disease is one that causes blunted agonist responsiveness. Studies have shown that in heart failure patients, this variant carries a relative risk of death or transplant of 4.8 compared with the normal allele. There also exists a particular polymorphism that tends to improve survival in those with heart failure. The existence of β-receptor polymorphisms may have additional implications for the efficacy of β-blockade. However, at this time, little is known about their particular impact on patient therapy [43].
Alpha-adrenergic receptors (AR) are also affected by aging [44–46]. Decreased expression of α-1A and α-1D receptors (involved in contractile function) has been noted with aging, which may be an adaption to cardiac hypertrophy [47]. A reduced responsiveness with age has been also reported for alpha-adrenergic receptors in healthier elderly patients [20] with potential implications for reduced muscle blood flow and augmented blood pressure during exercise. Interestingly, in normotensive older subjects, an increased rate of infusion of an α-agonist is required to achieve the same degree of vasoconstriction compared with young subjects [30]. Animal studies have shown that maximal binding of vascular α1-receptors is significantly reduced with age.
The α2-receptors appear to show some age-related decline. Normally, α2-receptors predominate in the venous side of the circulation, suggesting that a compromised venoconstrictor response to the upright posture, secondary to α2-receptor loss, might contribute to orthostatic intolerance in the elderly [48]. The evidence of adrenergic receptor desensitization with age has further implications as hypertension develops in the elderly. In normotensive elderly subjects, the decrease in responsiveness of α-adrenergic receptors seems to be a regulated compensatory effect of the heightened level of sympathetic nervous system activity in the elderly. Despite some evidence of diminished α-adrenergic responsiveness, it seems that the overall baroreflex control of vasoconstriction is well preserved with age and might be heightened compared with young adults [28, 49].
As with β-adrenoceptors, polymorphisms in α-adrenergic receptors may have implications on hypertension and cardiac disease in the elderly. It has been proposed that individuals with a particular α2B-adrenergic receptor polymorphism may be at greater risk for acute coronary events and sudden cardiac death [50]. In addition to the changes in α- and β-adrenergic receptors, dopaminergic receptor content and dopaminergic transporters decrease, and cardiac contractile responsiveness to dopaminergic stimulation is blunted with aging [51].
Sympathetic Nervous System Activity
The sympathetic nervous system exerts various effects on the cardiac physiology, including increase in atrioventricular conduction (positive dromotropy), heart rate (positive chronotropy), cardiac contractility (positive inotropy), and cardiac relaxation (positive lusitropy). Likewise, it plays a crucial role in the regulation of vascular tone due to its ability to control at the same time both peripheral resistances and cardiac output.
The sympathetic nervous system plays an important role in both aging and cardiovascular disease. Sympathetic nervous system activity increases with age, and by some estimates the sympathetic nerve activity is almost two times higher in a 65-year-old than a 25-year-old person [52]. This is probably due to increased catecholamine release, decreased neuronal uptake, and increased sympathetic nerve activity [53]. These alterations seem to be region specific and are seen in the skeletal muscle, splanchnic areas, and the heart [21]. Circulating norepinephrine concentrations increase by 10–15% per decade after adulthood [21, 54]. In addition, there is an age-dependent reduction in activity of the cardiac neuronal noradrenaline reuptake mechanism, resulting in higher concentrations of noradrenaline at β1-receptor sites in the heart [55]. Similarly, the increase in norepinephrine levels during exercise is greater in elderly subjects. The decrease in catecholamine sensitivity of adrenergic receptors in the heart and blood vessels reduces the response to the increased catecholamine release [56]. In the vasculature, however, the vasoconstrictor response is at least equivalent, if not exaggerated, in comparison to younger adults [52].
At the vascular level, systemically circulating or locally released catecholamines trigger two main classes of adrenergic receptors, α-1 AR and β-2 AR, causing vasoconstriction and vasodilatation, respectively. With aging, such a fine equilibrium is progressively shifted toward increased vasoconstriction, most likely due to a defective vasodilatation in response to β-2 AR stimulation. Supporting this hypothesis, β-AR agonist administration in the human brachial artery induces vasodilatation, and this response appears to be attenuated in hypertensive patients. The mechanistic role of β-2 AR in the vasculature is also corroborated by the fact that genetic variants of β-2 AR causing excessive desensitization have been shown to lead to reduced vasodilatation, promoting the development of atherosclerosis [57]. Long-term sympathetic stimulation is detrimental to the heart, and increased noradrenaline levels lead to changes in the collagen turnover and increased fibrosis [58].
Parasympathetic Nervous System Activity
Aging is associated with decreased responses to parasympathetic stimulation in cardiac and vascular tissues. Sympathetic tone predominates and vagal tone diminishes with the aging process. Females maintain greater vagal tone than males [59]. Vagal terminals and axons in cardiac ganglia will degenerate with the aging process [60]. One way to assess autonomic outflow of the cardiovascular system is to assess the heart rate variability. Heart rate variability has two components, a high-frequency component, which is under parasympathetic control, and a low-frequency component, which is under sympathetic control. Both components of heart rate variability decrease with age. Poor responsiveness to β-adrenergic receptor stimulation may explain depression in the sympathetic component, whereas low vagal output at rest is the likely mechanism behind the diminished parasympathetic, high-frequency variability [52].
The decreased vagal tone can be either due to reduced vagal outflow or reduced intracellular responses to muscarinic receptor activation with age [61]. Both changes seem to be present in the elderly. Lower resting vagal tone in the elderly has been implicated in the diminished heart rate increase in response to a large dose of atropine compared with younger controls. Studies have shown that right atrial muscarinic receptor density is significantly and negatively correlated with age [30]. Furthermore, it has also been shown that muscarinic receptor function declines in the elderly population. This is evident by a reduction in carbachol-induced inhibition of forskolin-activated adenylyl cyclase in muscarinic receptors of aged myocardium [62]. Finally, autoantibodies to M2-muscarinic receptors exist in the sera of normal individuals and are found in high levels in those with idiopathic dilated cardiomyopathy. The prevalence of these autoantibodies is significantly increased in the elderly [63]. All of these mechanisms, taken together , contribute to reduced vagal activity in the elderly. The implications of these findings on muscarinic receptor function and cardiac performance have yet to be determined.
Reflex Control Mechanisms and Aging
Reflex autonomic cardiovascular control mechanisms are altered in the elderly. The aging process affects autonomic cardiovascular control mechanisms in a nonuniform manner. Attenuated respiratory sinus arrhythmia in older individuals suggests that parasympathetic control of sinus node function declines with age. Because the reflex regulation of heart rate in humans is primarily dependent on cardiac vagal activity, it is correct to assume that the impaired baroreflex regulation of heart rate is related to deficient parasympathetic mechanisms (Fig. 11.10). Although the parasympathetic component of the arterial baroreflex becomes diminished in the aging population, the baroreflex control of sympathetic outflow and the vascular response to sympathetic stimulation are well maintained in moderately old, active individuals [48]. It is well established that basal levels of plasma catecholamines and sympathetic nerve activity increase with age.


Fig. 11.10
(a) Individual cardiac baroreflex sensitivities versus age. Regression revealed a significant (p < 0.05) inverse relationship between reflex sensitivity and age. (b) Mean regression lines describing relationship between mean arterial pressure and corresponding R-R interval for each of the three age groups. Regression line slopes were smaller in older and middle-aged subjects than in younger subjects. Baseline values (mean ± SE) are superimposed on regression lines (Adapted from Ebert et al. [48]. With permission from the American Physiological Society)
Endocrine Changes with Aging
The renin-angiotensin system (RAS) is central to physiologic control of sodium and water homeostasis. The RAS exists not only as an endocrine system but also as a local network in different organs, especially in the heart and brain. There, local conversion of angiotensinogen to angiotensin by regional angiotensin converting enzyme occurs. Angiotensin (AT) II principally mediates its effect through AT-I and AT-II receptors. AT-I receptors mediate fibrosis, oxidative stress, and myocardial hypertrophy among other effects. Although aging decreases overall RAS activity via decreased levels of systemic renin-angiotensin, increased local RAS activity has been observed in the heart. In addition, both AT-I receptors and AT-II receptors are upregulated. These changes in the RAS system contribute to age-related changes with cardiac remodeling [64]. Aging also affects sodium balance in the kidney. This results in decreased ability to conserve sodium in the face of sodium restriction as well as a decreased sodium excretion in the presence of increased sodium load. Despite the increased sympathetic activity accompanying old age, the elderly experiences a decrease in plasma and renal levels of renin. Plasma renin activity is diminished in the supine position, and physiologic stimuli such as hemorrhage, sodium restriction, and orthostasis are followed by attenuated increases in renin release and consequently lower concentrations of angiotensin in the circulation [65]. Although renin-angiotensin levels are decreased in the elderly, the aging population shows an enhanced vasoconstriction in response to angiotensin I and angiotensin II. The above finding helps to explain the key role that angiotensin-converting enzyme inhibitors and angiotensin II receptor blockers have in improving renal structure and function in the elderly [66].
In the elderly, there seems to be an elevation in plasma vasopressin levels under basal conditions and a heightened response to an osmotic challenge such as water deprivation. Surprisingly, after a water restriction period, older subjects demonstrate a relatively low spontaneous fluid consumption as well as diminished thirst [65]. In addition, by age 80, the total body water content has declined to 50% of body mass from the average content of 60% in younger persons [67]. Such decreases in thirst mechanism, total body water, and fluid consumption in combination with an age-related decrease in glomerular function cause older persons to be increasingly vulnerable to water imbalance.
Another group of hormones that are important in volume regulation are the natriuretic peptides. Atrial natriuretic peptide (ANP) is secreted primarily by the cardiac atria in response to atrial stretch, while brain natriuretic peptide (BNP) is secreted by both atrial and ventricular myocardial cells. Natriuretic peptides are primarily counter-regulatory hormones. They antagonize the effects of a number of sympathetic hormones and the renin-angiotensin-aldosterone system [68]. Seventy percent of all cardiac BNP is derived from the ventricles under normal conditions. In pathological conditions, the proportion of BNP derived from ventricles increases significantly [68]. Activation of these systems is seen most frequently in congestive heart failure, although many other conditions can also stimulate the release of NPs [68]. For example, increased levels of BNPs have been associated with aging, renal insufficiency, and anemia [69]. The changes in peptide levels are impressive enough to warrant higher cutoff levels of BNPs for diagnostic and prognostic purposes in the elderly [70].
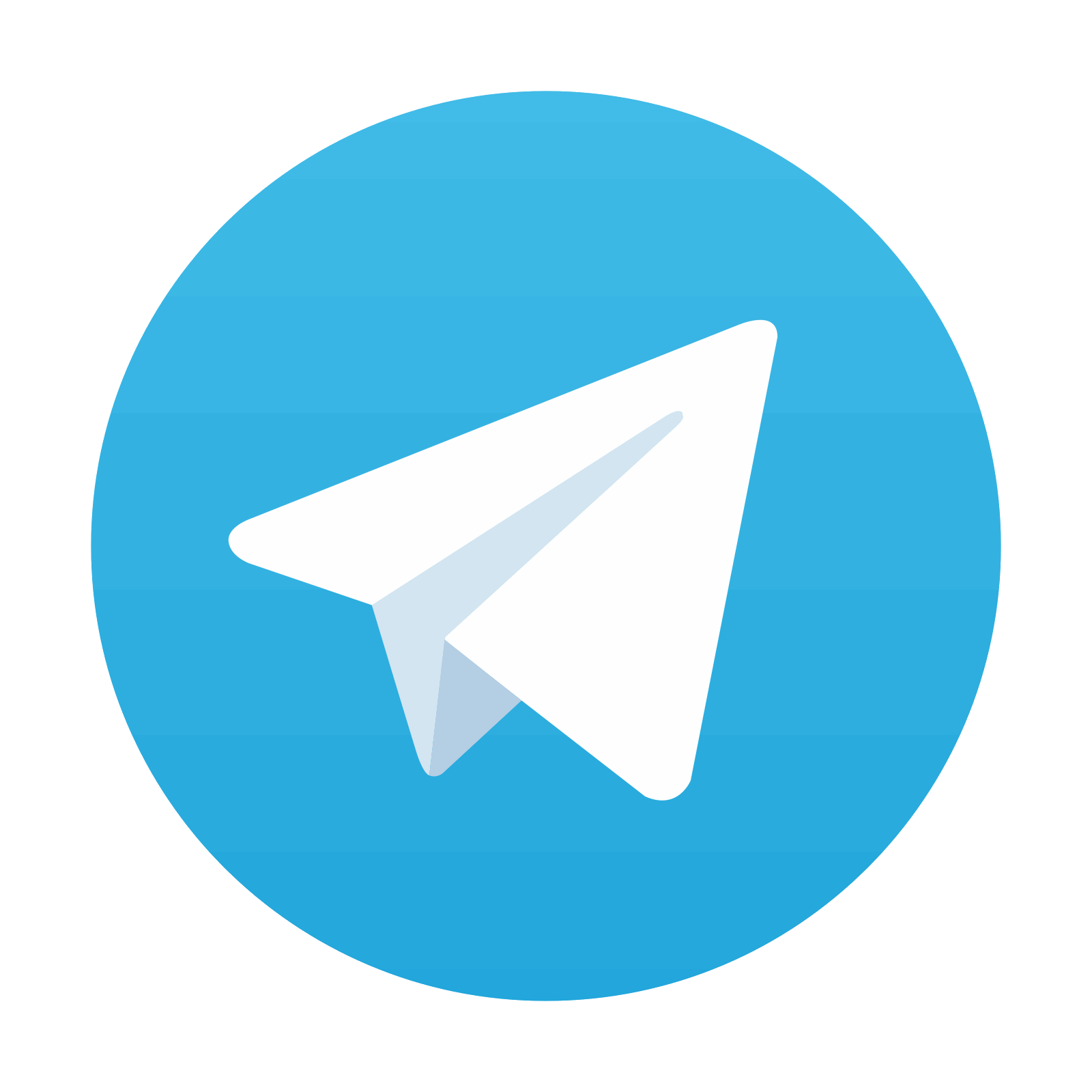
Stay updated, free articles. Join our Telegram channel

Full access? Get Clinical Tree
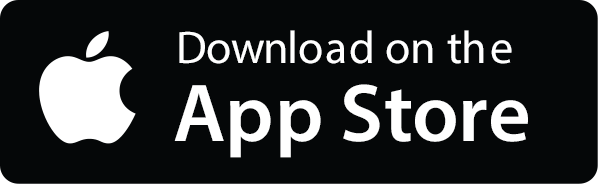
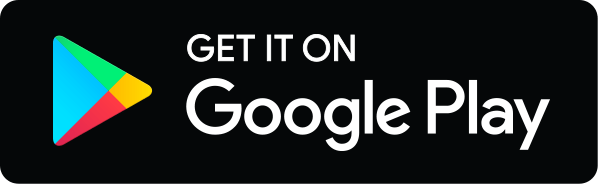