An illustration of the concept of the lung structure maintenance program. There are several homeostatic balances that contribute to lung cell growth and repair in response to environmental challenges.
Although gene deletion strategies designed to knock out VEGF or its receptors confirmed the central role of VEGF in vascular biology (6), it was not obvious that the lung depends critically on intact signal transduction mechanisms, which follow binding of VEGF to its cognate receptors and phosphorylation of its intracellular tyrosine kinase (7). The first clue was the marked abundance of the VEGF gene expression in the adult lung (7), which led to questions regarding the potential functional roles of VEGF signaling in the adult lung, which in contrast with rapid lung growth during development does not grow during adulthood. One potential answer to this question was that VEGF was necessary for “organ maintenance.” This straightforward paradigm has recently been both enriched and complicated by the discovery of multiple VEGF isoforms and soluble receptors, which can influence VEGF signal transmission (8,9). In addition, many cell types can generate and secrete VEGF in the lung, including epithelial cells, vascular smooth muscle cells, lymphocytes, mast cells and macrophages, and important, lung microvascular endothelial cells from which VEGF acts on these endothelial cells in an autocrine fashion (10). Thus, the lung microvascular endothelium generates its own survival/maintenance factor and may further modulate airspace structure as well (Figure 17-2). In the following sections we will relate the failure of the adult lung structure maintenance program to the loss of lung capillaries and airspace enlargement in COPD/emphysema.
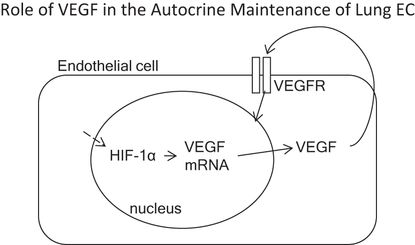
VEGF is of central importance for the survival of lung vascular endothelial cells. Microvascular lung endothelial cells generate and secrete large amounts of VEGF protein, which signals in an autocrine fashion.
Development of the Lung Vessels
Lung development follows the highly coordinated, transcriptionally controlled complex interactions between cells and cells and matrix. A “blueprint” for pattern formation and branching morphogenesis exists in the primitive lung bud. Alveolarization and formation of the capillary network during late fetal and postnatal life require diverse factors such as platelet-derived growth factor (PDGF), VEGF, and retinoic acid (11). Epithelial–endothelial signal exchange is critical for the building of a functional blood/gas interface.
In fact, vascular ablation results in a perturbed branching stereotypy and a flat branching morphology (12). Interestingly, the design-controlling role of the vasculature is independent of perfusion, and apparently loss of vasculature perturbs the spatial expression pattern of the key lung branching mediator, Fibroblast Growth Factor 10 (FGF 10) (12). VEGF 164 stimulates mouse branching morphogenesis and increases the number of Flk-1 + mesenchymal cells as well as the expression of bone morphogenetic protein 4 (BMP-4) and surfactant protein C (13).
Remarkably, the pulmonary arterial wall is constructed radially, from the inside out, and there is evidence for the formation of a capillary plexus via distal angiogenesis (14,15) (Figure 17-3). The intricate and simultaneous development of airways and vessels during fetal life and, most important, their interdependence is illustrated by the fact that epithelial branches are invested with an endothelial network that develops under the control of epithelially secreted VEGF. Reduction of available VEGF using a soluble VEGF receptor disrupts both capillary network and epithelial branching, whereas increased VEGF signaling stimulates vessel formation and airway branching (16).
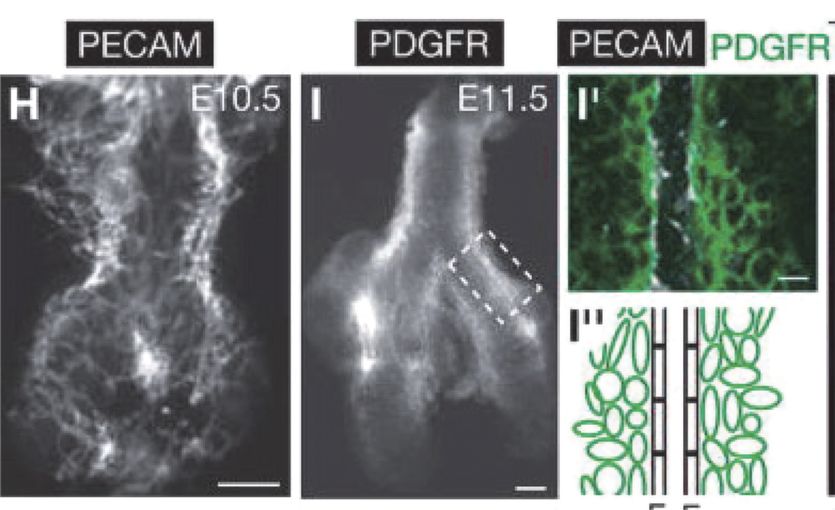
Development of pulmonary arteries in the mouse. Embryonic day 10.5 shows a vascular plexus that gives rise to the pulmonary arterial tube.
At low fetal lung oxygen tension (23 torr), mammalian target of rapamycin complex 1 (mTORC1) amplifies epithelial hypoxia-inducible factor 1 alpha (HIF-1alpha)-induced epithelial VEGF production and vasculogenic activity (17). Another transcription factor upstream from VEGF is peroxisome proliferator–activated receptor gamma coactivator (PGC-1alpha) (18). Whether PGC-1alpha is involved in hypoxic stimulation of VEGF transcription in the fetal lung is unknown; however, it has been reported that FOXF1 is required for the formation of the embryonic vasculature through regulation of VEGF signaling in endothelial cells (19). Regardless, it is clear that HIF-1alpha, HIF-2alpha, and VEGF play central and critical roles during lung development. As discussed later, these same actors play active roles in tissue destruction and the pathobiology of emphysema in the adult lung.
Recently, Dr. Mark Krasnow’s laboratory discovered alveolar progenitor/stem cells that coexpress alveolar type 1 (AT1) and alveolar type 2 (AT2) cell markers, and that these bipotent progenitors are the source of most, if not all, AT1 and AT2 cells during lung development. Thereafter, mature AT2 cells function as stem cells that are intermittently activated for alveolar renewal and repair (20). There are functional endothelial colony-forming cells (ECFC) in human fetal and rat neonatal lungs that have repair potential (21). c-Kit+/CD34+/VEGFR2+/Tie-2+ cells have also been described as a putative endothelial progenitor cell population in developing human lungs (22).
Can the Adult Lung Structure Maintenance Program Fail?
To further advance the concept of the lung structure maintenance program, we need to provide an experimental underpinning and search for “experiments of nature,” such as genetic and epigenetic conditions, that support this concept. The search for failure mechanisms began with the remark of a pathologist who, while examining lung tissue from a patient with severe COPD/emphysema, stated: “Emphysema is boring, there is just nothing there!” This “nothing there” or loss of alveolar structures and hole formation in the lung led to the hypothesis that alveolar cells undergo apoptosis and disappear in emphysema (23). We had been guided by a statement by the late Gordon Snider that “elastin degradation gives rise to emphysema, but not all airspace enlargements occur on such a background. Airspace enlargement may result from a connective tissue repair or growth process that is unable to preserve the normal alveolar structure of the lungs” (23). But the most important fact that argued for the existence of an adult lung structure maintenance program is the occurrence of emphysema in patients that never smoked (24). A recent study from Stockholm showed that low birth weight and preterm birth are risk factors for adult women to develop COPD (25). In this study, women born before thirty-two weeks of gestation had a hazard ration for obstructive lung disease of 2.7 (25). Although cigarette smoke is toxic to lung cells, airspace enlargement occurs in nonsmokers because the usually very robust response to endogenous oxidant stress factors or/and the response to low-grade environment stress such as air pollution is weakened.
The Histone Deacetylase (HDAC)/HIF-1alpha/VEGF Axis
The availability of a novel small molecule inhibitor of the intracellular tyrosine kinase section of the VEGF receptors 1 and 2 provided the opportunity to directly test the hypothesis that VEGF was required for the maintenance of the lung structure. This hypothesis was first tested in adult (1) and neonatal (3) rats. Animals were given the highly lipophilic drug, Sugen 5416 (semaxinib), as a single subcutaneous injection of a slurry. Four weeks later, treated animals showed impressive airspace enlargement and a dramatic loss of lung vessels when assessed by angiography (1). Semaxinib is presently in clinical use for the treatment of renal cell carcinoma. The important findings were that SU5416 decreased lung expression of VEGFR2 and Akt-1 and that cotreatment of the rats with a pan-caspase inhibitor prevented the development of SU5416-induced emphysema (1). In neonatal rat studies, animals were treated on the first day after birth with this VEGFR inhibitor, which markedly reduced alveolarization as well as pulmonary arterial density (3). This study was the first to demonstrate that angiogenesis is required for normal postnatal alveolarization during development, suggesting that impaired endothelial survival or function may contribute to developmental disorders of lung hypoplasia, such as bronchopulmonary dysplasia (3).
VEGF also commands antioxidant activity and is antiapoptotic, as VEGF treatment upregulates MnSOD and BCL-2 expression in endothelial cells (26, 27). Kasahara et al. translated the experimental findings to patient lung tissue samples and found decreased VEGF and VEGF receptor expression in lung tissue from patients with end-stage COPD/emphysema (28). These findings suggested that a first component or factor that was both necessary and required for the maintenance of the lung structure (e.g., VEGF) had been identified. This finding was surprising in view of the fact that the major transcription factor controlling VEGF gene expression is HIF-1alpha, and that HIF-1 alpha protein is stabilized by hypoxia. As many end-stage COPD patients are hypoxic, we speculated that there was either a transcriptional block, assuming that the expression of HIF-1alpha protein was elevated in the emphysematous lungs, or alternatively, that HIF-1alpha protein expression was paradoxically decreased in the hypoxic lung. Subsequent studies of tissue samples from banked human COPD lungs demonstrated marked reductions in VEGF and HIF-1 alpha gene and protein expression in the emphysematous lung tissue samples (29; Figure 17-4). Figure 17-5 shows the decreased expression of VEGF and phosho-Akt, reflecting decreased signaling.
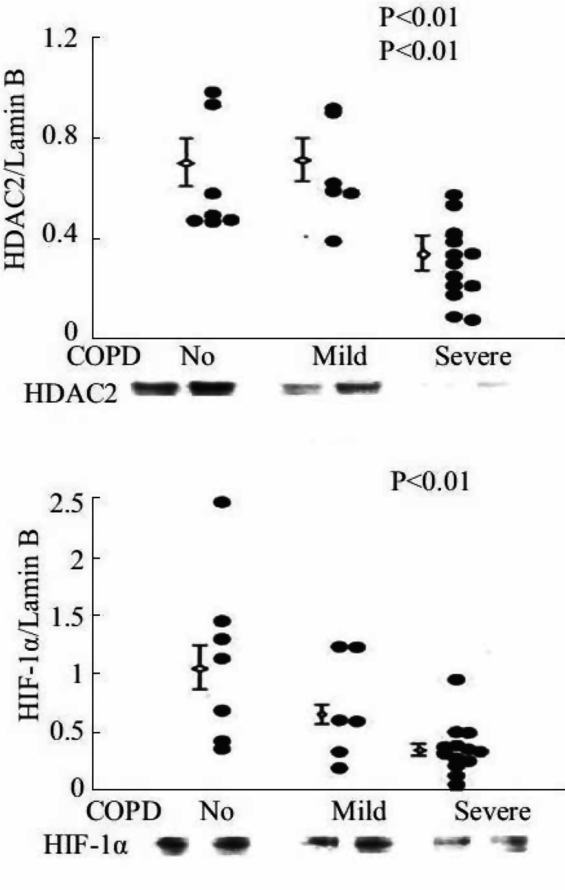
Expression of HDAC2 and HIF-1 alpha proteins in lung tissue samples of patients with severe COPD/emphysema.
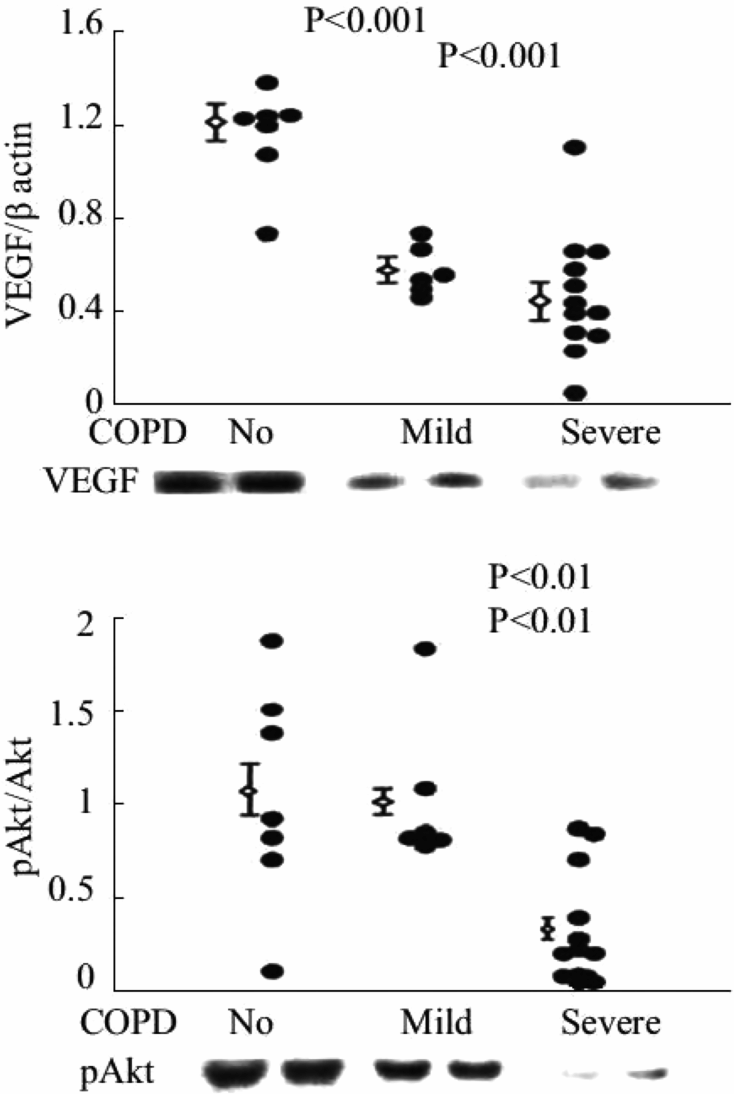
Expression of VEGF protein and the ratio of phosphorylated/non-phosphorylated Akt in lung tissue samples from patients with severe COPD/emphysema.
Thus, the diminished expression of lung tissue HIF-1 alpha, despite marked tissue hypoxia, was paradoxical and begged for a mechanistic explanation. Based on past studies suggesting key interactions between p53 (the “guardian of the genome”), HDAC and HIF-1alpha, HDAC 2 (Figure 17-4), and p53 protein expression were measured in COPD lung tissue samples (29). Ito and coworkers had examined lung samples from human COPD/emphysema patients and found a decreased expression and activity of HDAC proteins (30). This in turn led to the question whether experimental chronic inhibition of HDAC activity would lead to experimental emphysema. This indeed was shown in adult rats by Mizuno et al. (31). As histone modifications are part of the epigenetic repertoire, cigarette smoke exposure of lung cells, including endothelial cells, causes epigenetic changes that are partly dependent on HDAC expression and activity. One consequence of decreased HDAC expression in emphysematous lungs may be the decreased HIF-1alpha gene expression from end-stage COPD patients. If so, HDAC is situated upstream in the hierarchy of components that make up the adult lung structure maintenance program (Figure 17-6). Indeed, when the expression of the HDAC2 gene was experimentally reduced in cultured lung cells, the expression of HIF-1alpha was downregulated, further supporting the role for a HDAC → HIF-1alpha → VEGF axis (29).
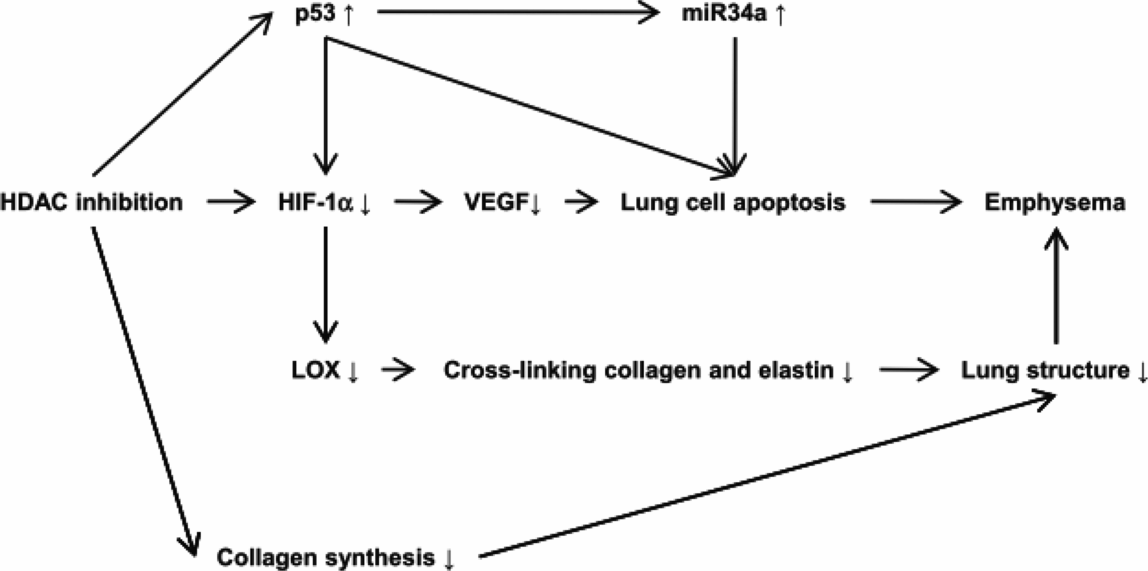
Proposed roles of HDAC 2, HIf-1alpha and VEGF in the lung structure maintenance program.
Copper and Sphingolipids
An experiment of nature, Menkes’ disease, which is based on a mutation of the gene encoding a copper transporter and impairs lung growth in infants, inspired our experimental work on the potential role for copper in the lung structure maintenance program. A further inspiration was the finding that the “blotchy mouse” carries the identical gene mutation as found in children with Menkes’ disease and that these mice develop emphysema without additional challenges (32). Copper is required for multiple metabolic activities, such as angiogenesis, collagen cross-linking (the activity of lysyl oxidase), antioxidant defenses (e.g., the activity of CuZn SOD), and transcriptional activity of HIF-1alpha. A copper-depleted diet or treatment with a copper chelator induced severe emphysema and decreased transcriptional activity of HIF-1 alpha in adult rats (32). Thus, copper is likely an essential component of the adult lung structure maintenance program. A study of dietary habits suggests that a section of the population is copper deficient (33), and there is also a study that shows smokers are copper deficient (34), as are patients with alpha-1 antitrypsin deficiency (35).
Spiegel and her group have provided evidence supporting the importance of sphingolipids in several diseases and introduced the concept of a sphingosine 1-phosphate /ceramide “rheostat.” (36) In addition, Petrache and coworkers established that lung tissue from COPD/emphysema patients are enriched with ceramides, that smoking induces human lung endothelial cell adaptation to apoptotic stress, and that ceramide reduces cell growth and induces apoptosis of lung endothelial cells (37,38). Yasuo et al. (39) treated adult rats with fenretinide, a synthetic derivative of retinoic acid that enhances ceramide production, and found that fenretinide caused emphysema. Interestingly, these structural changes were associated with an increase in the number of caspase-expressing lung cells and a decrease in lung VEGF protein expression. Concomitant treatment with sphingosine-1 phosphate injections reduced emphysema and restored lung HIF-1alpha, HDAC2, and VEGF protein expression. Overall, these experiments illustrate the importance of a balanced sphingosine metabolism for cell survival and growth in adult lung models of emphysema.
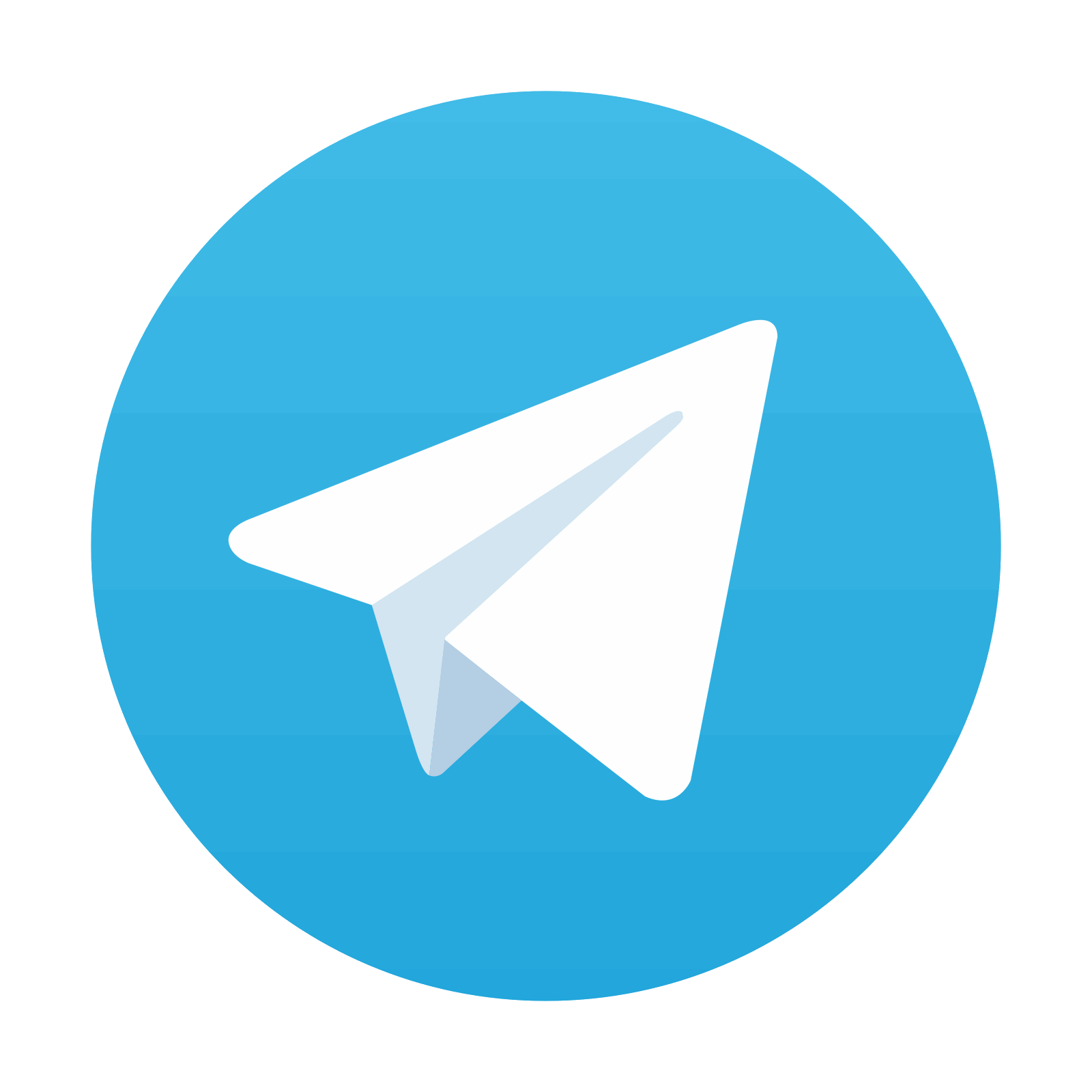
Stay updated, free articles. Join our Telegram channel

Full access? Get Clinical Tree
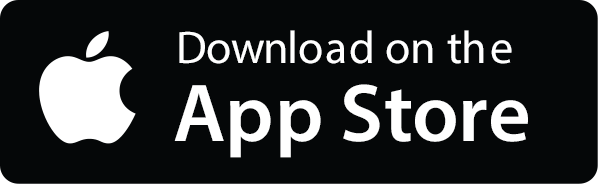
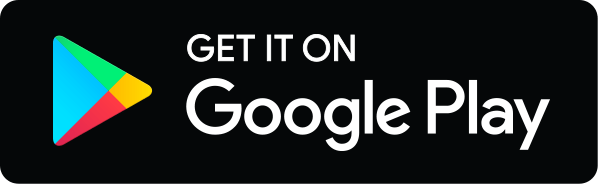