Reciprocal interactions between the developing lung endoderm and mesenchyme promote growth and branching of the airways. The developing lung airways are patterned in a proximal–distal manner with mesenchymal factors such as Fgf10 promoting growth and branching at the distal tip. Fgf10 and other mesenchymal factors act in concert with endodermally expressed signaling pathways such as BMP, Shh, and Wnt/β-catenin, which are all required for proper branching and growth of the developing airways.
Fgf Signaling
Fibroblast growth factors (Fgfs) and their receptors (Fgfrs) regulate a wide range of biological functions, including cellular proliferation, migration, and differentiation. Despite the numerous Fgfs expressed in the lung, Fgf10 in the mesenchyme and its receptor, Fgfr2b in the epithelium, have emerged as the primary Fgf ligand/receptor interaction required for branching morphogenesis in the lung (53). Fgf10 mutant lungs recapitulate the Fgfr2b mutants, with both mutants displaying complete lung agenesis distal to the trachea (54–56). Analysis of Fgf10 hypomorphs revealed fewer and shorter branches with loss of Fgf10 and complete loss of the accessory lobe. In addition, conditional loss of Fgf10 in the lung mesenchyme resulted in secondary branching defects (57). Together these results demonstrate a requirement for Fgf10 signaling in the initial budding of the lung from the trachea and strongly suggest a role for Fgf10 in later branching processes. Fgf9 is another important signaling ligand in lung development, as Fgf9 mutants showed decreased lung branching and changes in epithelial cell morphology (58).
Wnt Signaling
Nineteen Wnts are expressed in vertebrates, and the expression patterns of 5 Wnts have been characterized in the lung: Wnt2, Wnt2b, Wnt7b, Wnt5a, and Wnt11. Wnt2 and Wnt2b are expressed exclusively in the lung mesenchyme, whereas Wnt7b is expressed exclusively in the developing lung endoderm. Wnt5a is expressed in both the developing mesenchyme and endoderm of the lung in a temporal specific fashion (59,60). Loss of Wnt2 and Wnt2b, Wnt7b, and Wnt5a, as well as loss of β−catenin (a downstream effector of Wnt signaling), all cause various defects in lung development, establishing an important role for this pathway in lung development (61). The Wnt signaling pathway is activated when a Wnt ligand binds the Wnt coreceptors, including frizzleds and Lrp5/6. There have been at least five frizzled genes reported in the mouse lung (62). Once the ligand–receptor interaction has occurred, the canonical, or β-catenin-dependent pathway and the noncanonical, or β-catenin-independent pathways are activated. Canonical Wnt signaling frequently effects cell proliferation or cell fate specification; in contrast, the noncanonical Wnt pathways control components of cell migration and polarity that have effects on tissue morphogenesis.
Wnt2 and Wnt2b are required in a combinatorial fashion for lung endoderm specification. Loss of Wnt2/2b leads to complete respiratory organ agenesis, including loss of both trachea and lung development and loss of Nkx2-1 expression (63). Loss of both Wnt2 and Wnt7b causes loss of branching morphogenesis in the lungs after their initial formation (64). Moreover, Wnt2/7b double knockouts have significantly reduced smooth muscle development. Additional work is required to establish whether the defect in branching morphogenesis with loss of Wnt2/Wnt7b is due to the loss of smooth muscle or due to unreported defects in the epithelium (64). Wnt5a mutant animals have a very distinct defect in lung development characterized by lung hyperplasia, increased proliferation, and overbranching of the distal airways, without changes in cell specification (60). Overexpression of Wnt5a in the lung epithelium causes decreased branching, shortened outgrowth of new buds, and complete loss of the accessory lobe (60).
The Wnt receptor Fzd2 plays a key role in regulating epithelial cell behavior and tube morphology necessary for formation of new branch points during airway morphogenesis. Fzd2 is essential for regulating changes in epithelial cell shape and cell lengthening along the apical–basal axis, which is critical for formation of new domain branch points and maintaining proper airway tube shape in the developing lung (65). Loss of Fzd2 leads to decreased apical expression of phospho-myosin light chain 2 indicative of decreased Rho signaling, which is required for thickening of the lung epithelium prior to new branch formation (65).
Hedgehog Signaling
The canonical Hedgehog signaling pathway involves binding of the hedgehog ligand to one of the patched (Ptc) twelve-pass membrane protein receptors. Upon binding of hedgehog ligand to Ptc, inhibition of smoothened by Ptc is reversed, and Smo activates the Hedgehog pathway. Sonic hedgehog (Shh) signaling is required for branching morphogenesis and serves as a paracrine signal that regulates smooth muscle differentiation in the lung mesenchyme (66,67). Shh−/−mice have tracheoesophageal fistula and simple cyst-like lung sacs that fail to branch, although they exhibit some cell type specific differentiation (66, 68).
BMP Pathway
Evidence from normal and targeted misexpression studies in mice further suggests that BMP4 a TGF-β family peptide, plays a role in embryonic lung morphogenesis (69). Misexpression of BMP4 in Sftpc+ distal lung epithelium during development results in lungs that are smaller than normal with grossly distended terminal buds and large air-filled sacs at birth (69). Developmental loss of the BMP4 receptor BMPR1a leads to defects in distal lung epithelial proliferation and survival, and some of these defects are mimicked by loss of BMP4 in the developing lung epithelium (70).
Influence of Extracellular Matrix on Branching Morphogenesis
The ECM (extracellular matrix) is defined as the diverse collection of proteins and sugars that surrounds cells in all solid tissues. This tissue compartment provides structural support by maintaining an insoluble scaffold, and this in turn helps to define the characteristic shape and dimensions of organs and complex tissues. The lung ECM is primarily composed of collagen IV, laminins, nidogen, and proteoglycans (71,72). Although the ECM has historically been perceived as performing a primarily structural and hence biomechanical role, the ability of the ECM to provide the contextual information responsible for controlling both individual and collective cellular behavior has been recognized in recent years. Basement membrane components also play a dynamic role as a barrier and reservoir of growth factors, which in turn regulate epithelial and mesenchymal cell proliferation and differentiation. Absence or inhibition of the interaction of epithelial cells with the basement membrane has a direct consequence in the failure of normal lung development (73,74). Moreover, proper expression and function of extracellular matrix (ECM) molecules are necessary for branching to occur normally. However, little is known about the role of epithelial cell surface molecules that mediate epithelial-matrix interactions during this process.
Collagens
Collagens are a large family of structural proteins that are widespread throughout the body and are important for a broad range of functions, including tissue scaffolding, cell adhesion, cell migration, cancer, angiogenesis, tissue morphogenesis, and tissue repair. Previous work has shown that treatment with collagenase or inhibitors of pro-collagen synthesis disrupts lung epithelial branching (75–77). Furthermore, Collagen IV, the predominant collagen in the developing lung, is frequently found to be downregulated in mouse models where lung branching morphogenesis is perturbed (78). Together these data suggest a role for collagens in promoting and maintaining branching morphogenesis, and the direct mechanisms behind this influence are currently an active area of investigation.
Laminins
Laminins (LNs) are a family of extracellular matrix glycoproteins involved in cell adhesion, migration, proliferation, and differentiation during tissue development. LNs are composed of three chains, one central (α) and two lateral (β and γ) that are linked by disulfide bonds to form a cross-shaped molecule (79). To date five α, three β, and three γ chain isoforms have been described, which suggests that their combination can lead to approximately 30 variants of LN (80–85). Mouse embryos with a mutated LN α5 chain isoform die by E17.5, and their lungs show poor lobe septation and bronchiolar branching, suggesting that this LN α chain is indispensable for lung branching morphogenesis (86–88). Antilaminin antibodies were also shown to inhibit branching morphogenesis of lung explants (89). The mechanisms by which the branching epithelium interacts with the extracellular matrix to bring about this morphogenetic event are still unknown. Several integrin cell surface receptors that bind laminins may mediate the interaction between the matrix molecules and the epithelium to promote lung branching morphogenesis (90)
Integrins
Integrins are heterodimeric cell surface receptors composed of a single α and β peptide subunit (91). The extracellular domains of integrins interact with the ECM or other cell surface molecules, and some cytoplasmic domains have been shown to interact with the cytoskeleton (92,93). Within an integrin subfamily, a single β subunit is able to form heterodimers with several α integrins (91). The extracellular domain of the α integrin confers the binding specificity for the heterodimer, and the particular biological response to binding is determined by the α subunit cytoplasmic domain (94–96). Upon integrin binding of components of the ECM, signals are transduced that control diverse cell behaviors such as cell adhesion and migration (97). Loss of integrin alpha 3, which primarily associates with laminins, results in reduced branching with the large bronchi extending to the periphery of the lung (78). Loss of integrin beta 1, which primarily associates with collagens, results in branching defects and a loss of proper formation of a single-layered epithelium in the distal branch points of the airway tree (98,99). These results implicate a role for integrin receptors in basement membrane organization and lung-branching morphogenesis.
Summary and the Future
In this chapter we have given a brief overview of the various genetic factors, signaling molecules, and extracellular matrix components that contribute to branching morphogenesis in the lung. Despite the increased understanding of the molecular processes that regulate branching morphogenesis and early lung development, the physical processes that drive lung branching morphogenesis are just beginning to be elucidated (100). New advancements in imaging techniques, cell lineage specific reporter tools, and development of computational models for branching morphogenesis will allow researchers to explore the dynamic nature of this process in far greater detail than in previous studies (101,102). Together these new avenues of research will help unravel the systems biology of branching morphogenesis at the both the molecular and mechanical levels.
References
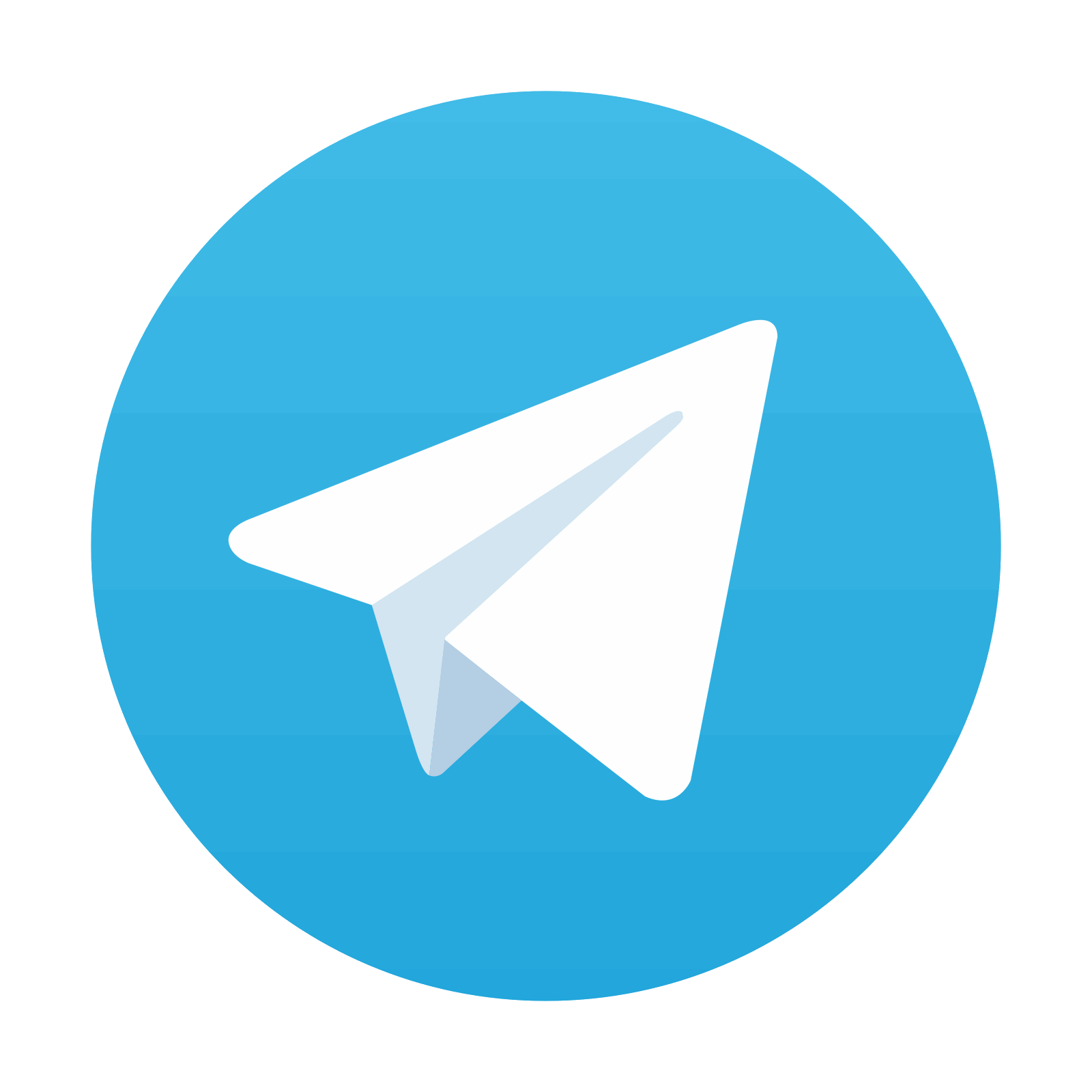
Stay updated, free articles. Join our Telegram channel

Full access? Get Clinical Tree
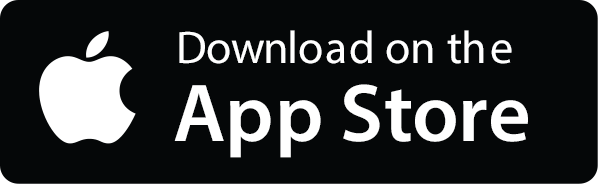
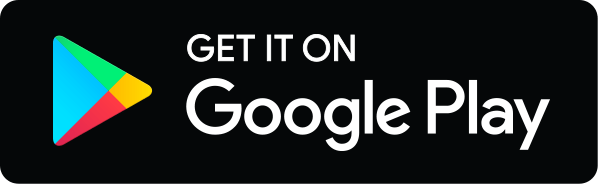