Hypertension is a polygenetic disorder provoked by remediable (e.g., sodium intake) as well as unmodifiable factors (e.g., aging). It accounts for up to half of cardiovascular events and is the leading risk factor for morbidity and mortality worldwide. To combat this public health epidemic, a number of lifestyle interventions (e.g., reduced sodium intake) have been extensively studied and proven over the years to effectively lower blood pressure (BP). As such, a central aspect of hypertension management endorsed by all guidelines is to identify and alleviate these established modifiable risk factors in individual patients. Conversely, little attention has been paid to another important as well as potentially remediable contributor to high BP— environmental exposures. Mounting evidence supports that colder ambient temperatures, winter season, higher altitudes, excessive noises, and air pollutants are capable of raising BP. Although the pressor effects are typically modest (5 to 15 mm Hg), billions of people are impacted on a daily basis. Some exposures also tend to overlap in certain settings such as in cities (e.g., noise plus air pollution) and travel destinations (e.g., high altitude plus cold). The full public health burden of environmental exposures remains to be established. However, it is likely to be enormous given their omnipresent nature. This chapter reviews the evidence linking environmental factors with high BP as well as the implications for clinical practice.
Environmental Risk Factors for High Blood Pressure
Colder Ambient Temperature and Winter Season
Colder temperatures increase BP over hours to days as well as over more prolonged seasonal periods. Studies across a wide range of populations and climates have demonstrated an inverse association between BP and ambient temperature during the same and/or preceding few days. In one of the largest studies (n > 500,000) conducted across China, a colder temperature of 10° C was associated with a 5.7 mm Hg increase in systolic BP. The impact was even more robust among older adults, those with smaller body mass indices; but was obviated by household central heating. Systolic BP was also on average 10 mm Hg higher during winter compared with summer. These results resemble our findings among 2078 cardiac rehabilitation patients in Michigan whereby reductions in outdoor temperatures by 10.4° C during the prior 1 to 7 days promoted a 3.6 mm Hg increase in systolic BP. Moreover, in both studies temperatures below 5° C did not prompt further elevations in BP. Similar inverse associations between outdoor temperature and BP have also been reported in other recent studies including patients with cardiovascular disease, individuals living in rural China (e.g., 13% lower hypertension control rate during winter), in a large Dutch population (n = 101,377), and across several locations in Italy.
Independent effects of both winter season and cold temperatures on BP have been reported. Cold exposures measured using personal monitors were shown to be associated with higher systolic BP levels during the daytime, even after adjusting for changes in daylight hours (i.e., season). Conversely, nighttime BP was higher during summer (i.e., warmer days) compared with winter months in this as well as in a few other studies. We recently demonstrated similar findings using personal monitoring. Warmer nights (independent of season) led to higher BP levels several hours later during the following afternoon. Along with a few prior studies, these observations support that there is a highly complex interrelationship involving several exposure-related factors (time of day, duration, indoor versus outdoor temperature levels) that determine the true nature of the ensuing BP changes.
Brief exposure to cold induces a rapid thermoregulatory vasoconstriction, thus raising BP. Although the mechanisms responsible for the more persistent pressor responses during winter are likely similar, they may not be entirely identical ( Table 8.1 ). Further physiologic adaptations (e.g., lower vitamin D, weight gain, reduced activity, and changes in diet/fluid balance) likely play additional roles. Conversely, changes in other meteorologic factors such as humidity and barometric pressure have not been consistently associated with BP.
Environmental Factor | Effect of Exposure on Blood Pressure | Possible Mechanism(S) |
---|---|---|
Temperature
| Overall effect: Inverse association Colder outdoor/indoor ambient temperature associated with higher BP Cold increases BP variability and central aortic pulse pressure Acute heat (e.g., sauna treatment) lowers BP Warmer daytime associated with higher nocturnal BP Warmer nighttime temperature associated with higher next day BP | Direct thermoregulation-mediated vasoconstriction HPAA and SNS activation, sodium/volume retention Impaired endothelial-dependent vasodilatation Reverse of cold mechanisms Possibly reduced sleep quality |
Season
| Overall effect: Winter season associated with higher BP levels Reduced temperature may be primarily responsible; however, winter may have some additional independent effects | Cold-induced mechanisms plus chronic alterations may play additive roles: lower vitamin D levels, reduced activity, weight gain, shifts in fluid balance (aldosterone increase), and increased arterial stiffness. |
Geography
| Overall effect: Higher altitude (>2500 m) raises BP Ascent to higher altitudes raises BP (variable interindividual responses) May be affected by race, acclimatization, rate of climb, or duration of exposure. Long-term population studies are limited in ability to determine effect and show heterogeneous results on chronic BP levels because of many confounding variables. | Altitude-induced hypoxemia activates the chemoreflex along with compensatory responses causing increased SNS and adrenal activity. Long-term acclimatization may lead to differing responsible responses. Other associated factors such as colder temperatures and stress may also play a role. Long-term increases in red blood cell mass may contribute |
Loud noises | Overall effect: Exposure to loud noises raises BP Numerous conditions implicated (ambient, traffic, airports) | Acute SNS activation, HPAA activation, endothelial dysfunction Possibly impaired sleep quality because of nocturnal noise |
Pollutants
| Overall effect: Exposure to pollutants raises BP Short and long-term PM exposures related to higher BP and hypertension Multiple size ranges (fine, coarse, ultrafine) of PM and sources (urban, rural, biomass, and personal-level) of exposures related to higher BP levels SHS exposure raises BP Lead, cadmium, arsenic, mercury, POP, bisphenol A, strong odors, phthalates | Acute activation of the SNS via pulmonary autonomic reflexes rapidly raises BP. PM constituents reaching the systemic vasculature and promoting vasoconstriction may also play a role Chronic exposures likely alter vascular tone via endothelial dysfunction or reduced arterial compliance (reduced nitric oxide and higher endothelins) because of PM-mediated systemic inflammation and oxidative stress in the vasculature as well as in the central nervous system |
The overall evidence supports that both colder ambient temperatures (over a few hours to days) as well as winter seasons (over more prolonged periods) lead to clinically-meaningful elevations in BP. It is possible that this plays a role in the known increase in cardiovascular events during winter. From a clinical standpoint, patients with hypertension should be more carefully monitored during colder weather to ensure adequate BP control. In one study, 38% of patients required added antihypertensive medications during winter. Although some epidemiologic evidence suggests that residential heating might mitigate the prohypertensive effects of cold, further studies are needed before making definite recommendations in this regard for the sole purposes of preventing winter-induced hypertension. What if any other practical steps (e.g., space heaters, warmer clothes) individuals with hypertension can take to lessen the ill effects of cold exposures on BP require further investigation.
Noise
A diverse array of loud conditions has been implicated in raising BP including traffic, airplanes, and occupational noises. Brief exposures can increase BP within minutes. Additional studies show that residing at locales chronically impacted by loud noise (e.g., traffic and airplanes) increases the risk for overt hypertension. Given the variety of conditions implicated and the linear association between decibel intensity and BP elevation, the source appears to be of secondary importance. Nevertheless, some studies suggest that nighttime exposures (i.e., aircraft noise) may have a particularly detrimental impact.
Recent reports have furthered our understanding of the adverse effects of noise on promoting hypertension. A metaanalysis of 24 cross-sectional studies demonstrated a 7% increase in the prevalence of hypertension per 10 decibel increase in a 16-hour average traffic noise exposure. Residing within 50 meters of a major roadway has also been independently linked to a 13% higher incidence of hypertension in a large cohort of women (n > 38,000) across the United States. Additional research has attempted to dissociate the ill effects of noise from other coexposures (e.g., traffic noise versus air pollution). Although many studies support that noise is independently linked to higher BP and/or hypertension, others have found it difficult to disentangle the individual contributions.
The mechanisms whereby noise triggers an acute increase in BP and promotes chronic hypertension have begun to be elucidated ( Table 8.1 ). The main pathways include an activation of the sympathetic nervous system, the release of stress hormones, and stimulation of the hypothalamic pituitary adrenal axis. Recent experiments show that nocturnal exposures can disrupt sleep quality and impair vascular endothelial function, even when patients are not consciously aware or awoken by the noise.
More than half of the global population now lives in cities. Roughly 40% of the European population is exposed to excess traffic noise. It has also been estimated that about 150 million people in the United States are exposed to noise levels placing them at excess risk for hypertension. The health importance of the documented BP-raising effect of noise is not entirely clear; however, it may play an important role in the mechanisms linking traffic and aircraft noise with acute cardiovascular events. Large-scale policies regarding urban planning (e.g., roadway and airport proximities) as well as local ordinances that seek to reduce nuisance noises (e.g., construction) can mitigate exposures. It is unclear whether health care providers should recommend patients to take action at work (e.g., wear protective ear muffs), home (e.g., close windows, use noise silencers), or in the health care environment (i.e., hospitalization) to reduce exposures to excessive noise for the sole purposes of lowering BP.
Higher Altitude
During ascent to higher altitudes BP increases over a few days independent of the effect of colder temperatures. The magnitude of responses varies within and between individuals and may even differ between races. However, the acuity of exposure appears to be an important factor, as those who have acclimatized over weeks tend to exhibit smaller changes. Although the duration of response is not fully elucidated, some studies show that it may persist for weeks to months if remaining at higher altitude. The overall evidence strongly supports an adverse effect of altitude on BP among individuals undergoing short-term ascents above 2500 meters ; whereas a few studies suggest that even lower altitudes (1200 m) may also present some risk. Among the various mechanisms responsible, a key factor is hypoxia-induced activation of the chemoreflex and an ensuing augmentation of sympathetic outflow (see Table 8.1 ). Other pathways include increased arterial stiffness, endothelin release and heightened blood viscosity. The impact of acclimatization rates, exposure durations, peak altitude, and patient susceptibilities (e.g., black race) require more investigation.
The literature regarding living at higher altitudes and developing chronic hypertension as well as the risk for excess cardiovascular events is more mixed. This may reflect confounding because of common coexposures (e.g., cold, stress), as well as differences in other ecologic, genetic, and lifestyle variables between populations. However, a recent metaanalysis of eight studies in Tibet (n = 16,913) among individuals living at 3000 to 4300 m above sea level showed a positive relationship between hypertension and higher altitude. Every 100 m increase in altitude was independently associated with a 2% increase in hypertension prevalence. More studies are required given the public health importance of this issue.
Recent studies have also evaluated the effectiveness of pharmacologic interventions to prevent the effects of high altitude on BP. Twenty-four hour BP levels were elevated after staying 12 days at Mt. Everest base camp (n = 45). There was a progressive increase in systolic BP (10 to 15 mm Hg) and plasma norepinephrine levels from 3400 m and up to 5400 m that started immediately and persisted throughout prolonged altitude exposure. Moreover, BP normalized upon return to sea level. Angiotensin receptor blockade (ARB) was not capable of mitigating the pressor response to high altitude; whereas it slightly lowered absolute BP levels (4 mm Hg) compared with placebo at 3400 m, but it was ineffective in this regard at the higher altitude (5400 m). This is consistent with the findings that circulating markers of renin-angiotensin activity were suppressed at higher altitudes. Similar results were reported in a study of 89 patients with mild hypertension climbing 3260 m in the Andes. Combined treatment with an ARB plus calcium channel blocker (CCB) did not blunt the magnitude of high altitude-induced increases in BP (10 to 15 mm Hg systolic). However, absolute BP levels remained significantly lower during combination therapy compared with placebo at all altitudes.
From a clinical standpoint, guidelines for managing high BP as well as cardiovascular risk in patients ascending to higher altitudes (typically above 2500 m) have been published. This issue is of growing public health importance given the roughly 35 million people per year who travel worldwide above this altitude. Recommendations include proper preparation and acclimatization (when warranted) along with careful BP monitoring during time spent at higher altitudes. This may even include periods of travel (or seasonal relocation) to relatively lower altitudes (e.g., 1200 m) among at-risk or susceptible patients. A return to lower altitudes may even be justified in some situations, such as for severe or refractory high BP. Beta blockers and ARBs do not prevent the pressor response; whereas combination ARB plus CCB therapy has shown some efficacy in controlling absolute BP levels at high altitude. The optimal regimen and appropriate clinical scenario when to modify hypertension management (e.g., increase or add medications) to control altitude-induced BP elevations remains to be clarified.
Air Pollution
Air pollution is a leading global risk factor for morbidity and mortality. One of the most important pollutants is fine particulate matter (PM) 2.5 μm, commonly derived from the combustion of fossil fuels (e.g., coal) from a host of modern-day activities (e.g., traffic, power generation, industry). Over the past decade a growing body of studies has demonstrated that exposure to PM 2.5 , along with several different air pollutants, is capable of raising BP. As an example, we recently showed among 2078 patients living in southeast Michigan that day-to-day variations of ambient PM 2.5 levels (8.2 μg/m 3 ) during the prior few days were independently associated with significant increases in BP by 2.1 to 3.5/1.7 to 1.8 mm Hg. This occurred despite the fact that patients were well-treated using contemporary secondary prevention medications (e.g., statins, beta blockers) and the air quality was excellent from a global perspective. In fact, mean PM 2.5 levels (12.6 μg/m 3 ) were well within daily United States National Ambient Air Quality Standards (<35 μg/m 3 ). At the other end of the dose spectrum, more extreme PM 2.5 levels (from 50 to >550 μg/m 3 ) in cities such as Beijing are also linked to short-term as well as more chronic elevations in BP. A recent metaanalysis of up to 25 studies from across the world concluded that an increase in PM 2.5 by 10 μg/m 3 during the prior few days is associated with an elevation in BP of 1.4/0.9 mm Hg. Chronic exposures over the prior year resulted in even more robust responses (7.3/9.5 mm Hg). In support of these findings, several randomized double-blind controlled trials of exposures to fine, coarse (2.5 to 10 μm) and diesel exhaust (10 to 100 nm) have shown that the acute inhalation of PM across a wide range of size fractions and derived from a variety of sources are capable of rapidly raising BP (2 to 10 mm Hg) over a few hours.
Beyond the acute pressor response, exposure to PM 2.5 on a chronic basis is linked to development of overt hypertension. In a cohort of 33,303 adults living a clean environment (Ontario, Canada), a long-term increase of PM 2.5 levels of 10 μg/m 3 was associated with a 13% higher incidence of hypertension. Similarly, a 14% increase in the onset of hypertension was found in association with chronic exposure to traffic-related pollutants in black women living in Los Angeles. There is even evidence that living in regions across the United States with higher ambient PM 2.5 levels increases hypertension-related mortality.
A wide array biologic mechanisms have been shown to be involved in air pollution-mediated BP elevations. PM 2.5 inhalation activates the sympathetic nervous system via autonomic reflexes following stimulation of a variety of receptors (e.g., transient receptor potential channels) throughout the pulmonary tree. Another important pathway is the genesis of systemic inflammation resulting from the “spill-over” of numerous circulating factors (cytokines, activated immune cells, oxidized lipoproteins) from the lungs. Thereafter, this can adversely impact the entire cardiovascular system by triggering vasoconstriction and endothelial dysfunction. Finally, there is accruing evidence that some prooxidative constituents of inhaled particles (e.g., nanoparticles, metals, organic compounds) may be capable of reaching the systemic circulation and thereby directly impact the cardiovascular system.
Beyond regional (e.g., industry) and point-sources (e.g., traffic) of widespread ambient air pollution, a more localized source, secondhand smoke (SHS) from cigarettes, can also raise BP. SHS increases the risk of elevated home BP values as well as the prevalence of masked hypertension. We have shown by controlled exposures as well as personal monitoring that short-term SHS inhalation causes elevations in BP over a few hours-to-days. Perhaps even more important, a number of studies have also began to show that long-term exposures are capable of promoting the development of chronic hypertension.
The negative influence of air pollution on BP is of clinical relevance. Emergency department visits for hypertension have been shown to be increased following more air polluted days in several countries including Canada (low levels) and China (extremely high levels). Hypertension-related mortality has also been linked to chronic PM 2.5 exposures. Reductions in citywide and national ambient air pollution levels because of governmental regulations provide substantial improvements in cardiovascular health as well as reductions in all-cause mortality. From a clinical practice standpoint, some studies have shown that several personal-level interventions can be effective in reducing harmful exposures and thereby blunt or mitigate the prohypertensive responses. These include wearing high efficiency filter (HEPA) facemasks while outdoors and/or closing outside windows while in heavily polluted cities, as well as using household and automobile cabin HEPA filtration systems. Decreased exposure to SHS also results in substantial reductions (10% to 20%) in cardiovascular events within a few months of instituting smoking ordinances. Clinical recommendations regarding practical methods to assess and reduce the cardiovascular risks related to air pollution have been outlined in detail.
Other Environmental Factors
Several additional environmental factors common to modern societies have proven capable of increasing BP including exposures to persistent organic pollutants, strong odors (e.g., nearby farm animals), certain metals (i.e., lead, cadmium, mercury, arsenic), and several endocrine-disrupting chemicals used in plastics such as beverage bottles (e.g., bisphenol A) and food wraps (e.g., phthalates). Facial immersion in cold water also acutely raises BP (i.e., diving reflex). There is additional evidence that living at more extreme northern or southern latitudes is associated with higher BP (possibly related to lower vitamin D). Finally, extraordinarily rare exposures shown in a few case reports to significantly alter BP include the inhalation of moon dust by astronauts (elevates BP) and zero gravity space flight (lowers BP because of vasodilatation despite increased cardiac output).
Summary of Evidence
The main findings in the published literature and the biologic mechanisms linking environmental exposures to BP changes are summarized in Table 8.1 . The majority of studies report average systolic BP elevations between 5 and 15 mm Hg following relevant exposures either commonly or plausibly encountered in real-world scenarios. Note that some patients may experience even larger responses and extreme exposures may produce even greater pressor responses than typically reported.
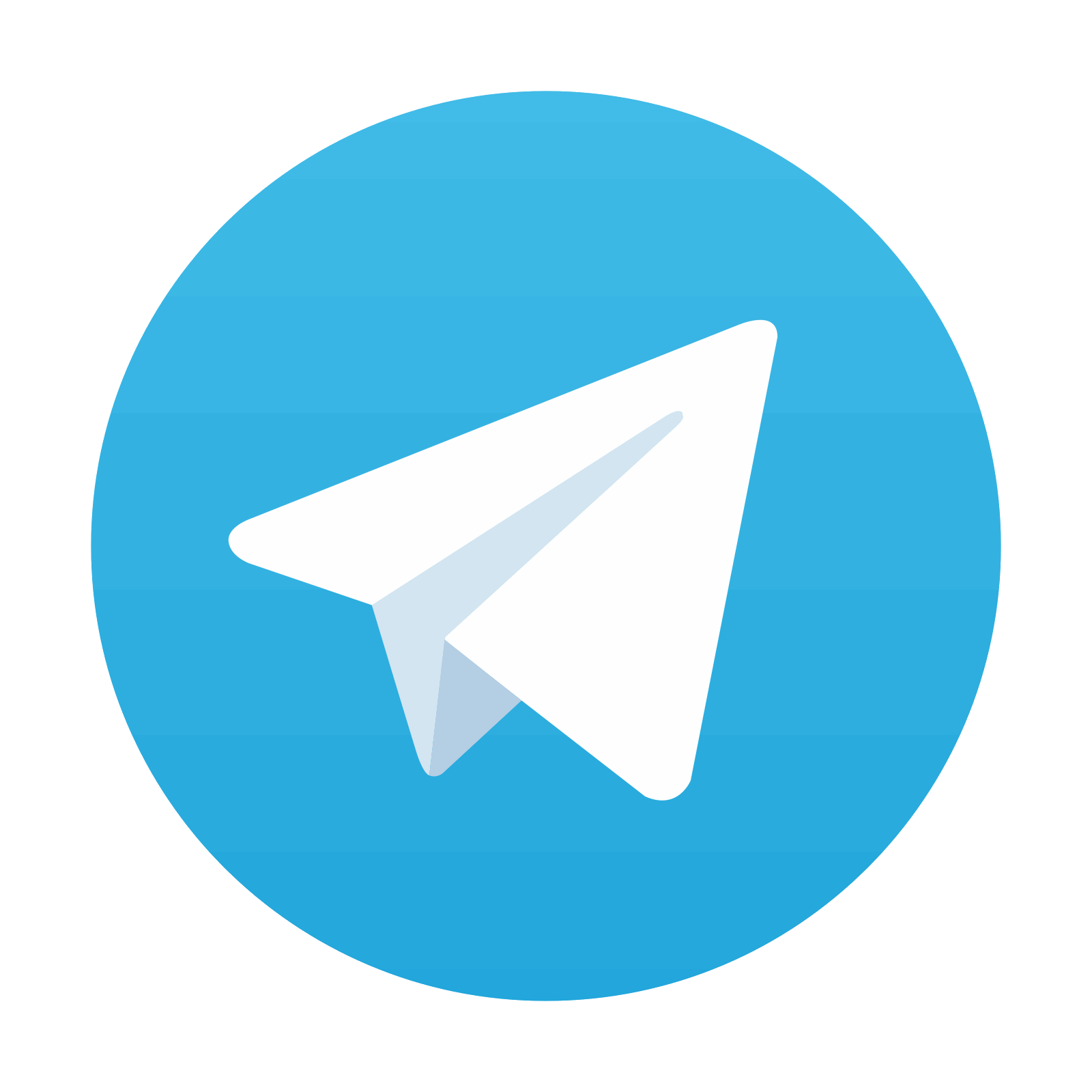
Stay updated, free articles. Join our Telegram channel

Full access? Get Clinical Tree
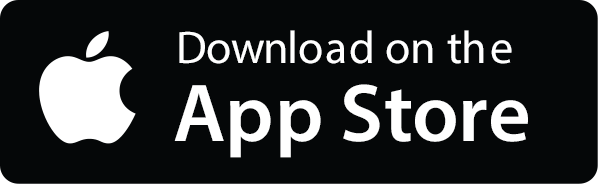
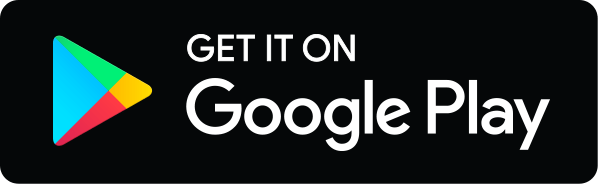