The burden of heart failure remains ever present with a long-standing, inexorable association with hypertension. Though advances in evidence-based medical therapy for reduced ejection fraction heart failure have led to impressive reductions in morbidity and mortality, the residual burden of disease remains significant.
Heart failure is recognized as any condition characterized by a mismatched relationship between metabolic, exercise, and/or cognitive needs and cardiac performance. Several important phenotypes have been clearly identified: heart failure with reduced ejection fraction (HFrEF); heart failure with preserved ejection fraction (HFpEF); and heart failure with either “improved” or “borderline” ejection fraction. Especially for HFrEF, a cogent pathophysiology has been well established. Neurohormonal activation, likely initiated by ventricular deformation from acute or chronic ventricular injury, causes a cascade of maladaptive biologic responses typically characterized by renin-angiotensin-aldosterone and sympathetic nervous system activation. These perturbed systems (and other neurohormonal circuits) perpetuate left ventricular dysfunction through progressive remodeling, that is, changes in both shape and size, followed by a deterioration of systolic performance. Disease severity varies from the complete absence of any symptoms of heart failure (New York Heart Association, or NYHA, class I) through progressive degrees of limitation including symptoms at rest (NYHA class II to IV).
The causes of heart failure are protean. Traditional putative etiologies of left ventricular dysfunction, systolic or diastolic, include coronary artery disease, valvular heart disease, various specific cardiomyopathies, and concomitant arrhythmias leading to tachycardia-induced left ventricular dysfunction. Less frequent but still important causes include metabolic disturbances, such as diabetes and thyroid disease; myocarditis; toxic conditions mostly attributed to chemotherapeutic agents, alcohol, and illicit drugs; and human immunodeficiency virus (HIV). There are many other notable conditions leading to heart failure and like all of the foregoing considerations, there is reasonable evidence of a true causal effect.
Hypertension has traditionally been associated with heart failure, and it has been relatively easy to infer empirically a cause and effect relationship. However, although the evidence is irrefutable that hypertension is a risk factor for heart failure it has been less clear that hypertension is a causal factor for heart failure. Further, it is important to recognize the unique contribution of hypertension to HFpEF, a phenotype of heart failure which is now the predominant clinical syndrome recognized in hospital settings and responsible for more than 50% of all acute heart failure admissions. Unlike HFrEF where clarity of the pathophysiology exists, the cellular and molecular aspects of the pathophysiology of HFpEF remain elusive. Prevailing considerations implicate fibrosis, ventricular noncompliance, hypertrophy, and ischemia; all of which can be impacted by hypertension. Likely, there is no overarching maladaptive pathway that is the root cause of this important condition, but hypertension when aligned with coronary artery disease, obesity, diabetes and atrial fibrillation, explains the majority of concomitant comorbidities associated with clinical HFpEF.
Therapeutic considerations are clear for HFrEF, but less certain for HFpEF. Management of HFpEF is hindered by the absence of a clear mechanism of left ventricular dysfunction and by the heterogeneity of persons with HFpEF. There are reasonable but not definitive data suggesting a potential benefit of mineralocorticoid receptor antagonists (MCRAs) and early signals that neprilysin inhibition in combination with renin-angiotensin-aldosterone blockade may be helpful. The best guidance continues to prompt a unique focus on concomitant comorbidities, including hypertension, for which evidence-based clinical practice guidelines exist.
For HFrEF, defined treatment algorithms are available and are populated with evidence-based therapies proven to improve outcomes. Recent American College of Cardiology (ACC)/American Heart Association (AHA)/Heart Failure Society of America (HFSA) clinical practice guidelines make clear the importance of both prevailing standard bearers of therapy: angiotensin-converting enzyme (ACE) inhibitors, angiotensin receptor II blocker (ARBs), evidence-based beta-blockers, mineralocorticoid receptor antagonists (MCRAs), hydralazine and isosorbide dinitrate (ISDN), and implantable cardioverter defibrillator/cardiac resynchronization therapy (ICD/CRT); and newer therapies: valsartan/sacubitril and ivabradine. The expected outcomes of optimal therapy for HFrEF are now substantially better than historical expectations. Yet, whether the condition of heart failure is HFrEF, HFpEF or even HF with improved or borderline ejection fraction, it remains clear that prevention is the more preferable intervention.
The American College of Cardiology Foundation (ACCF)/(AHA) guideline for the management of heart failure has adopted a stepwise progression to characterize the natural history of heart failure. This framework organizes treatment strategies for preventing and controlling risk factors like hypertension (stage A), treating subclinical structural and functional changes like LV hypertrophy and mechanical dysfunction (stage B), and reducing morbidity and mortality in symptomatic heart failure (stages C and D) ( Fig. 32.1 ). This framework emphasizes the importance of intervening early in the progression of heart failure during stages A and B before development of symptoms.

Of all potential strategies that might reduce the incidence of heart failure, none appears to have higher yield than the treatment of hypertension. Thus, a greater exploration of the association of hypertension and heart failure is warranted. In this chapter, we report on: epidemiologic analyses that establish the strong association between hypertension and heart failure; longitudinal and experimental studies that elucidate mechanisms by which hypertension leads to clinical heart failure; and landmark trials in patients with and without clinical heart failure that demonstrate the potent effect of antihypertensive therapy on the prevention of heart failure events in individuals with or at risk for heart failure.
The Heart Failure Epidemic
Worldwide there are 37 million people living with heart failure. In the United States, approximately 5.7 million Americans live with heart failure and prevalence increases with older age ( Fig. 32.2 ). Moreover, each year more than 650,000 new heart failure cases are diagnosed. Although advances in heart failure treatment have improved survival, approximately 50% of people diagnosed with heart failure will die within 5 years. The economic consequences of heart failure are also staggering. Heart failure accounts for more than 1 million hospitalizations each year, and many of the patients with heart failure are at high risk for repeat hospitalization with 30-day readmission rates of 25%. In 2012, total costs for heart failure was estimated to be $30.7 billion. If current trends continue, projections forecast that by 2030, prevalence will increase to more than 8 million individuals, and total costs will increase to $70 billion.

Hypertension in the Development of Heart Failure
Population-based cohorts have played a fundamental role in establishing hypertension as the dominant, modifiable risk factor for heart failure in the general population. The Framingham Heart Study was the first study to describe the association between hypertension and heart failure. During the first 16 years of follow-up in the original Framingham cohort, antecedent hypertension was reported in 75% of the 142 individuals with a new case of heart failure. Subsequent analyses in Framingham reported a two-fold greater hazard for developing heart failure in men with hypertension compared with those without hypertension (hazard ratio [HR] 2.07, 95% confidence interval [CI] 1.34 to 3.20) and a three-fold greater hazard in women (HR 3.35, 95% CI 1.67 to 6.73) ( Table 32.1 ). When coupled with the high prevalence of hypertension in the population, hypertension explained 39% of the population attributable fraction of heart failure in men and 59% in women. Longer-term analyses by Lloyd-Jones et al also highlighted the contribution of hypertension to the lifetime risk of heart failure. Among men and women in Framingham, the lifetime risk of heart failure was approximately 1 in 5 but was twice as great in individuals with blood pressures 160/100 or more mm Hg compared with those with blood pressures less than 140/90 mm Hg ( Fig. 32.3 ).
Men | Women | |||
---|---|---|---|---|
Risk Factor | Adjusted Hazard Ratio (95% CI) | Population-Attributable Risk, % | Adjusted Hazard Ratio (95% CI) | Population-Attributable Risk, % |
Hypertension | 2.07 (1.34-3.20) | 39 | 3.35 (1.67-6.73) | 59 |
Myocardial infarction | 6.34 (4.61-8.72) | 34 | 6.01 (4.37-8.28) | 13 |
Angina pectoris | 1.43 (1.03-1.98) | 5 | 1.68 (1.23-2.30) | 5 |
Diabetes mellitus | 1.82 (1.28-2.58) | 6 | 3.73 (2.71-5.15) | 12 |
Left ventricular hypertrophy | 2.19 (1.49-3.21) | 4 | 2.85 (1.97-4.12) | 5 |
Valvular heart disease | 2.47 (1.70-3.60) | 7 | 2.13 (1.54-2.94) | 8 |

Cohort analyses have also demonstrated the unique contribution of hypertension to heart failure risk in women. In Lloyd-Jones and colleagues’ lifetime risk analysis, the risk of heart failure in men was markedly lower for those without antecedent myocardial infarction. However, lifetime risk of heart failure in women was similar regardless of the history of myocardial infarction. Importantly this observation emphasizes the nonatherosclerotic mechanisms through which heart failure likely occurs in women. Analyses comparing risk factors for HFpEF with HFrEF support these observations by revealing that female sex and systolic blood pressure are both associated with increased odds of HFpEF over HFrEF (odds ratio [OR] 2.29, 95% CI 1.35 to 3.90 and OR 1.13 per 10 mm Hg, 95% CI 1.04 to 1.22, respectively).
In African Americans, hypertension has been implicated as a key risk factor in the racial disparities seen with heart failure. In both the Atherosclerosis Risk in Communities (ARIC) study and the Multi-Ethnic Study of Atherosclerosis (MESA), heart failure incidence was higher in African Americans compared with other race/ethnic groups but differences were attenuated, yet not eliminated, after adjustment for the higher prevalence and poorer treatment of traditional risk factors like hypertension ( Table 32.2 ). These findings meet the definition of a true health care disparity in African Americans with hypertension. Baseline characteristics from some of the earliest heart failure trials such as the Veterans Administration Vasodilator Heart Failure Trials (V-HeFT I and II) and the Studies on the Left Ventricular Dysfunction (SOLVD) also highlight the comparatively stronger association of hypertension and weaker association of myocardial infarction with systolic dysfunction in African Americans compared with whites. An even more definitive set of observations emerged from the African American Heart Failure Trial (A-HeFT). In this study of NYHA class II-IV heart failure completed in 1050 African Americans, approximately 50% of participants provided an antecedent history of hypertension whereas fewer than 25% had an overt history of ischemic heart disease as a cause of left ventricular systolic dysfunction.
Incidence Rates Per 1000 Person-Years of Follow-Up | Unadjusted Hazard Ratio for Heart Failure (95% CI) | Adjusted Hazard RATIO for Heart Failure (95% CI) | ||
---|---|---|---|---|
African Americans | Whites | |||
Men | 9.1 | 6.0 | 1.38 (1.16-1.63) | 0.86 (0.70-1.06) |
Women | 8.1 | 3.4 | 1.96 (1.04-3.67) | 0.93 (0.46-1.90) |
Data from the Coronary Artery Risk Development in Young Adults study (CARDIA), a population-based cohort of young adults, further underscore how earlier onset and poorer control of hypertension contribute to early heart failure risk in African Americans. Among the 5115 African American and white adults age 18 to 30 years in CARDIA, 26 out of the 27 incident heart failure events at 20 years of follow-up occurred in African Americans. As an additional observation, for every 10 mm Hg higher diastolic blood pressure there was an associated doubling in the hazard for early-onset of heart failure in African Americans (HR 2.1, 95% CI 1.4 to 3.1). These profound data solidify the unique contribution of hypertension to early-onset heart failure in African Americans.
Hypertensive Heart Disease
Hypertensive heart disease describes a spectrum of conditions related to hypertension that progresses from subclinical structural, mechanical, cellular, and extracellular myocardial changes all the way to clinical symptoms of heart failure. In this paradigm, the hemodynamic load caused by elevated blood pressure increases left ventricular (LV) wall stress leading to a compensatory thickening of the LV wall and an increase in LV mass. Factors like race, sex, neurohormones, cytokines, and growth factors modulate this hypertrophic response, resulting in fibrosis, myocardial stiffness, mechanical dysfunction, and eventually heart failure ( Fig. 32.4 ).

Left Ventricular Hypertrophy and Remodeling
Although LV hypertrophy can precede hypertension, it is generally considered to be the first step in the development of hypertensive heart disease. Data from the Framingham Heart Study first demonstrated the association between electrocardiogram (ECG)-defined LV hypertrophy and subsequent cardiovascular events. As imaging modalities improved, direct measurement of LV wall thickness and mass with echocardiogram and magnetic resonance imaging (MRI) confirmed the direct and linear association between blood pressure and LV mass, an association that was even stronger using longer-term ambulatory blood pressure monitoring. Epidemiologic studies then demonstrated the association between LV mass and incident cardiovascular disease. For example, in the Cardiovascular Health Study (CHS), higher LV mass index on echocardiogram was associated with systolic and diastolic dysfunction and future heart failure risk, independent of the prevalence of incident myocardial infarction. In MESA, Bluemke et al used cardiac MRI to demonstrate the association between higher levels of LV mass and incident heart failure (HR 1.4 per 10% increment, 95% CI 1.2 to 1.5), a risk that primarily occurred in individuals with LV hypertrophy.
Although the stages of hypertensive heart disease suggests a unidirectional progression, randomized trials like the Losartan Intervention for Endpoint Reduction in Hypertension (LIFE) trial have demonstrated that antihypertensive agents can reduce LV mass, a finding that is also associated with improved outcomes in post hoc analyses. In a meta-analysis of 80 trials comparing the efficacy of different antihypertensive drug classes for reversing LV hypertrophy in hypertensive patients, Klingbiel et al identified that LV mass index decreased by 13% reduction with ARBs, 11% with calcium channel blockers, and 10% with ACE inhibitors.
Systolic and Diastolic Dysfunction
Echocardiography has also played an essential role in elucidating the effects of high blood pressure on cardiac mechanical function leading to clinical heart failure. Although the direct link between hypertension and systolic dysfunction is often complicated by concomitant cardiovascular disease, analyses like those from CHS have demonstrated the direct and stepwise relationship between LV mass and systolic dysfunction, independent of the prevalence of incident myocardial infarction.
Diastolic dysfunction, referring to abnormalities in LV relaxation and filling, is a hallmark of hypertensive heart disease. Because the LV remodels in response to hypertension, cardiac myocytes hypertrophy, and fibrotic changes occur that increase LV stiffness and alter cardiac mechanical properties. In a cross-sectional survey of residents from Australia, diastolic dysfunction was prevalent in 34.7% of adults aged 60 to 86 years and was independently associated with a diagnosis of hypertension (OR 1.5, 95% CI 1.2 to 2.0), an observation that has also been reported in residents from Olmsted County, Minnesota. More recently, Santos et al analyzed data from 4871 participants in ARIC to demonstrate that abnormalities in LV thickness and diastolic parameters even occur in participants with prehypertension (blood pressure of 120 to 139 mm Hg systolic and/or 80 to 89 mm Hg diastolic). In these analyses, prehypertensive participants had higher LV mass indices and higher rates of mild, moderate, and severe diastolic dysfunction compared with those with optimal blood pressures (blood pressure < 120/80 mm Hg).
In recent years, speckle-tracking echocardiography, which uses computer algorithms to track pixels of imaging data, has emerged as a novel technique to directly measure and quantify myocardial displacement, velocity, and deformation (stretch or contraction). Abnormalities in these measures of cardiac mechanicals have been shown to be precursors of heart failure. Choi et al demonstrated in MESA that circumferential strain was associated with future heart failure risk in asymptomatic individuals, even after adjusting for age, diabetes, hypertension, myocardial infarction, LV mass, and LV ejection fraction (HR 1.15 per 1%, 95% CI 1.01 to 1.31). Blood pressure can adversely affect myocardial strain. In CARDIA, Kishi et al demonstrated that cumulative exposure to nonoptimal systolic and diastolic blood pressure in young adulthood was associated with lower longitudinal strain rate and lower early diastolic longitudinal peak strain rate, two preclinical signs of heart failure, at 25 years of follow-up. Few participants in these analyses had blood pressures exceeding conventional treatment thresholds at any point during follow-up, highlighting the implications of blood pressure exposure during the lifespan and the importance of primordial prevention, that is, the prevention of hypertension per se as a means to prevent more overt cardiovascular disease including heart failure.
Cellular and Extracellular Changes
In addition to structural and mechanical changes from hypertension, multiple studies have revealed cellular changes that develop in the setting of hypertension and LV hypertrophy. In animal models of pressure-hypertrophied myocardium, stress loading increased microtubule density leading to abnormalities in cell microarchitecture that impaired myocyte contractile function. Transverse (t)-tubules, cell structures that regulate calcium cycling for normal myocyte contractions, have also been linked to hypertensive heart disease. In one study, Wei et al demonstrated that thoracic aortic banding led to t-tubule remodeling early in the development of hypertrophy. This remodeling was present before echocardiographic evidence of LV dysfunction and worsened as heart failure progressed ( Fig. 32.5 ). These findings were also confirmed by Shah et al in hearts from spontaneously hypertensive rats. In response to chronic exposure to elevated blood pressures, t-tubules became disorganized, leading to impairments in intracellular calcium cycling and abnormal myocardial strain before overt evidence of LV dysfunction on echocardiogram. These studies provide a biologic underpinning for ultrastructural cellular changes that lead to abnormal myocardial mechanicals and eventually precede clinical heart failure.

Changes in the extracellular matrix have also been shown to play an important role in the progression from hypertensive heart disease to heart failure. Exogenous administration of deoxycorticosterone acetate, a mineralocorticoid, has been shown in animal models of hypertension and LV-pressure hypertrophy to increase myocardial fibrosis, oxidative stress, diastolic stiffness, and filling pressures. Abnormalities in levels of matrix metalloproteinases (MMP) and tissue inhibitors of MMP (TIMP) have also been implicated in the progression of hypertensive heart disease. In one study that compared patients with LV hypertrophy to controls, those with hypertension and normal LV structure had normal MMP levels, whereas those with hypertension and LV hypertrophy had an MMP/TIMP profile that favored extracellular matrix degradation and collagen accumulation (low MMP-2 and MMP-13 levels, high MMP-9 levels, and high TIMP-1 levels). Endomyocardial biopsies have confirmed the association between adverse MMP/TIMP levels and cardiac fibrosis and LV dilatation.
Genetic
Given the substantial individual and race/ethnic variability in hypertensive heart disease, there is burgeoning interest in identifying genetic determinants of LV hypertrophy. In both the Hypertension Genetic Epidemiology Network study (HyperGEN) and the Dallas Heart Study, African-American adults with and without hypertension had a two-fold to three-fold greater odds of LV hypertrophy, even after adjusting for cardiovascular risk factors and body mass. Although heritability analyses from family-based cohorts such as Framingham and HyperGEN have confirmed heritability of LV mass in both whites and African Americans, the correlations are modest and explain only a small portion of the variance in LV mass. Candidate gene-based studies have identified genes that encode proteins involved in LV performance as well as proteins that modify cell signaling, myocyte growth, calcium metabolism, and blood pressure. However, many of these studies are small and include subjects with vastly different ethnicities, limiting reproducibility. Recently, large genome-wide association studies have been used to identify genetic loci associated with LV mass, LV wall thickness, and ECG-evidence of LV hypertrophy. Although these genetic investigations offer much hope for targeted, molecular interventions to treat and prevent hypertensive heart disease, much remains to be discovered about the causal variants at or near candidate loci and their functional significance. These epidemiologic, imaging, and mechanistic studies provide a compelling rationale for identifying hypertension as a key risk factor in the progression of heart failure. However, it is the potent and consistent improvement in heart failure outcomes seen in clinical trials with blood pressure-lowering agents that singles out hypertension treatment as a fundamental target of any heart failure prevention strategy.
Landmark Hypertension Trials to Prevent Symptomatic Heart Failure
There are significant data to suggest that the incidence of heart failure may be favorably modified through optimal management of hypertension, especially in those with a higher burden of cardiovascular risk ( Table 32.3 ). The earliest clinical trials testing antihypertensive drugs from the Veterans Administration Cooperative Study Groups reported reductions in heart failure events, but sample size was small and events were few. The Systolic Hypertension in Elderly Program (SHEP) was one of the first hypertension trials to include a prespecified endpoint examining the efficacy of antihypertensive therapy (chlorthalidone 12.5 to 25 mg plus atenolol 25 to 50 mg, if needed) in prevention of heart failure. Participants randomized to diuretic-based stepped care had a 49% reduction in fatal and nonfatal heart failure events during an average follow-up of 4.5 years (2.3% versus 4.4%; relative risk [RR] 0.51, 95% CI 0.37 to 0.71). Similarly, in the Hypertension in the Very Elderly Trial (HYVET), randomization to the indapamide plus perindopril (as needed to achieve a target blood pressure of 150/80 mm Hg) group was associated with a 64% reduction in heart failure events (5.3% versus 14.8%; RR 0.36, 95% CI 0.22 to 0.58) compared with placebo at 2 years. Trials such as the Heart Outcomes Prevention Evaluation (HOPE) study demonstrated that ACE inhibitors could also reduce heart failure events in high-risk participants. In the HOPE study, among participants with diabetes mellitus or established vascular disease, ramipril treatment was associated with a 23% reduction in heart failure events (9.0% versus 11.5%, RR 0.77, 95% CI 0.67 to 0.87) after a mean of 4.5 years of follow-up.
Study | Number of Participants | Inclusion Criteria | Intervention | Duration (Years) | Mean BP Difference Between Groups (mm Hg) | Absolute Rates of Heart Failure (Intervention Versus Comparator) | Relative Risk of Heart Failure (95% CI) |
---|---|---|---|---|---|---|---|
SHEP 1997 | 4736 | Age ≥ 60 years; SBP ≥ 160 mm Hg | Chlorthalidone ± atenolol | 4.5 | −26.0/−8.9 | 2.3% versus 4.4% | RR 0.51 (0.37-0.71) |
HYVET 2008 | 3845 | Age ≥ 80 years; SBP ≥ 160 mm Hg | Indapamide ± perindopril | 2.1 | −15.0/−6.1 | 5.3% versus 14.8% | RR 0.36 (0.22-0.58) |
HOPE 2000 | 9297 | Age ≥ 55 years; vascular disease or DM + 1 CV risk factor | Ramipril | 4.5 | −3/−2 | 9.0% versus 11.5% | RR 0.77 (0.67-0.87) |
ALLHAT 2000, 2002 | 33,357 | Age ≥ 55 years; HTN + 1 CV risk factor | Chlorthalidone versus amlodipine; Chlorthalidone versus lisinopril | 4.9 | −0.8/+0.8 −2.0/0 | 7.7% versus 10.2% 7.7% versus 8.7% | RR 0.62 (0.48-0.75) RR 0.81 (0.69-0.93) |
SPRINT 2015 | 9361 | Age ≥ 50 years; SBP ≥ 130 mm Hg; high CVD risk without DM | SBP target <120 mm Hg versus SBP target <140 mm Hg | 3.3 | −18.2/−9.4 | 1.3%/years versus 2.1%/years | HR 0.62 (0.45-0.84) |
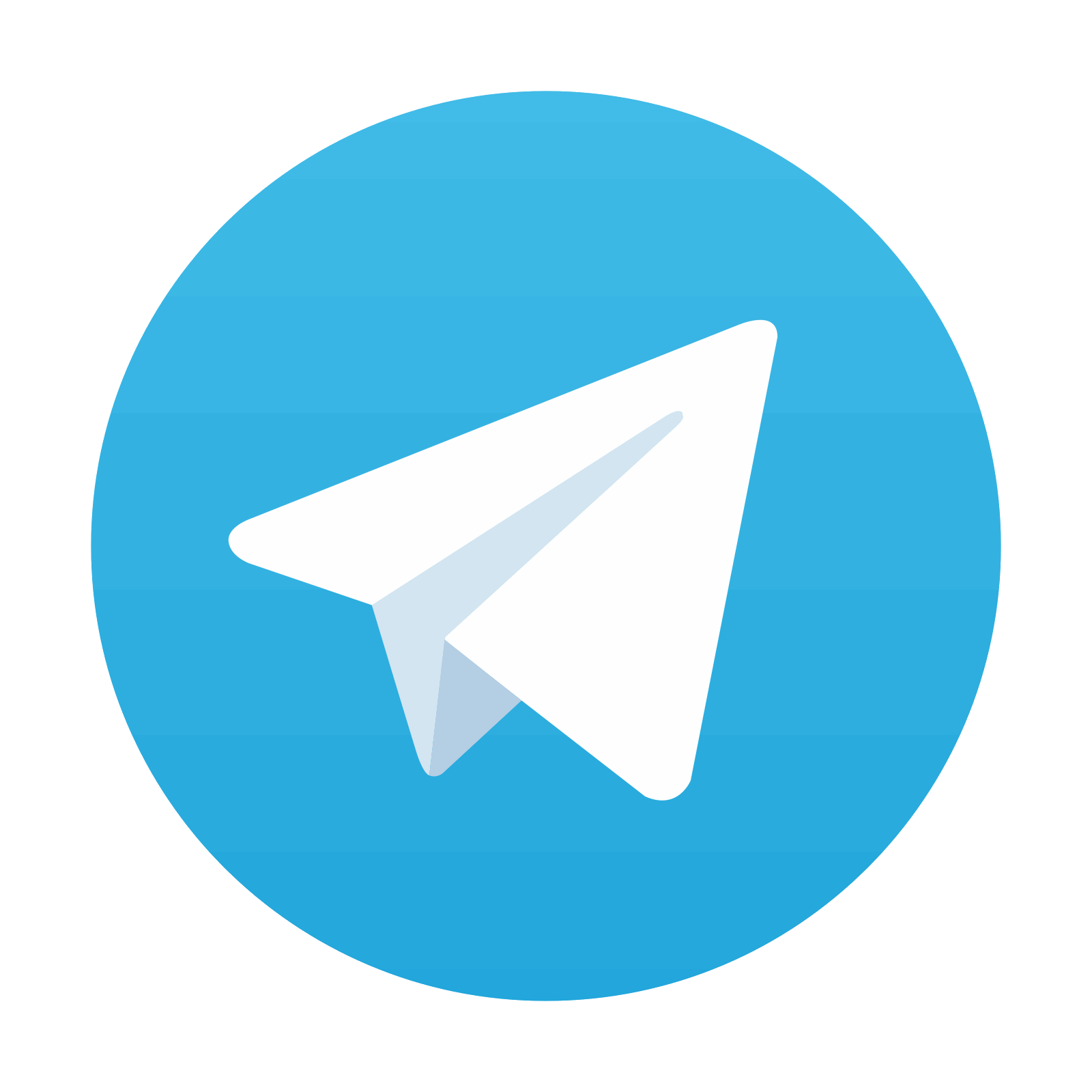
Stay updated, free articles. Join our Telegram channel

Full access? Get Clinical Tree
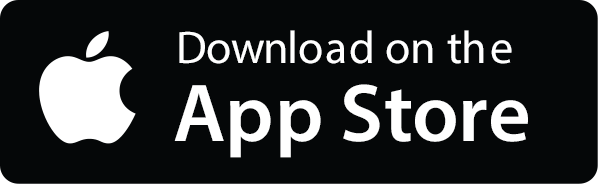
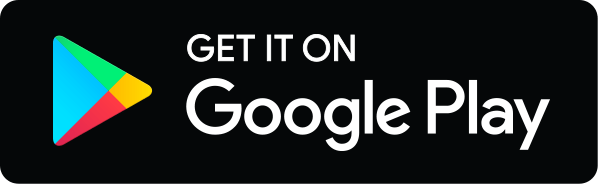
