6 The Electrophysiology Laboratory and Electrophysiologic Procedures
The electrophysiology study (EPS) is an invasive procedure that involves the placement of multipolar catheter electrodes at various intracardiac sites. Electrode catheters are routinely placed in the right atrium, across the tricuspid valve annulus in the area of the atrioventricular (AV) node and His bundle (a special part of the conduction system), in the right ventricle, in the coronary sinus, and sometimes in the left ventricle (Fig. 6-1). The general purposes of EPS are to characterize the electrophysiologic properties of the conduction system, induce and analyze the mechanism of arrhythmias, and evaluate the effects of therapeutic interventions. Invasive electrophysiology techniques and procedures are routinely used in the clinical management of patients who have supraventricular and ventricular arrhythmias (Table 6-1). In today’s laboratory, the use of computer-generated “electroanatomical maps” are very much a part of the jargon and even a novice must be able to recognize the color-coded activation patterns of common arrhythmias shown later in this chapter. Individuals seeking a more in-depth discussion of the procedures and concepts described should refer to the Suggested Readings section.
Table 6-1 Clinical Applications of Electrophysiologic Studies
Diagnostic |
Therapeutic |
• Select appropriate candidates for cardioverter-defibrillator and anti-tachy cardiac pacing therapy |
Interventional |
Prognostic |
AV, Atrioventricular; SVT, supraventricular tachycardia; VT, ventricular tachycardia; WPW, Wolff-Parkinson-White.
Personnel
The most important aspects for the performance of safe and effective electrophysiologic procedures (especially for catheter ablations) are the presence and participation of dedicated personnel functioning as a seamless team, taking direction from the clinical electrophysiologist performing the procedure. The minimum personnel requirements include at least one physician, one to two nurses and technicians, an anesthesiologist on site or on standby, and a biomedical engineer (on the premises) to troubleshoot and repair equipment.
The clinical electrophysiologist is ultimately responsible for all aspects of the procedure and should be fully trained in an accredited electrophysiology fellowship program in all aspects of clinical cardiac electrophysiology. He or she should be a cardiologist who has spent preferably 2 years of additional training in an active electrophysiology laboratory and has met criteria for certification. The guidelines for training in clinical cardiac electrophysiology were developed by the American Heart Association and the American College of Cardiology in collaboration with the Heart Rhythm Society. The criterion for certification in this subspecialty has been established by the American Board of Internal Medicine. The skills clinical electrophysiologists possess should include the ability to implant devices, perform transseptal punctures, extract chronic indwelling pacemaker and defibrillator leads, and cannulate the pericardial space to map and ablate ventricular arrhythmias.
Equipment
An electrophysiology laboratory is equipped with radiographic imaging systems, a recording and monitoring system, a stimulator, and all drugs and equipment required for ACLS (Fig. 6-2). Although a dedicated laboratory would be preferable, in many institutions, these procedures are carried out in the cardiac hemodynamic-angiographic catheterization laboratory. If used for pacemaker and defibrillator implantation procedures, the room should have air filtering equivalent to a surgical operating room. It is important that the EP laboratory have appropriate radiographic imaging equipment with an image intensifier capable of cinefluoroscopy and coronary angiography. Pulsed fluoroscopic systems allow for the production of radiation in short bursts instead of in a continuous manner. Use of pulsed fluoroscopy is a necessity to keep radiation exposure to a minimum. The fluoroscopic unit should be equipped with a cumulative timer that will remind the operator of each 5-minute period of fluoroscopy exposure and the total amount. To reduce radiation exposure, especially as the procedures get longer and more complex, there is a trend across all laboratories to increasingly rely on nonfluoroscopic imaging such as electroanatomical mapping and ultrasound imaging. The equipment must be capable of obtaining views in multiple planes with an installed biplane mode when possible.
Electrode Catheters
Diagnostic Catheters
The hallmark feature of an electrophysiology catheter is the presence of at least two ring electrodes that can be used for bipolar and unipolar pacing and recording of local myocardial electrical activity. The material used to construct these catheters may be of the woven Dacron variety or synthetic materials such as plastic or polyurethane. The number of electrodes in these catheters can vary from 2 to 20 and the inter-electrode spacing can vary from 2 to 20 mm and the thickness can vary from 4 F to 7 F. The shape of these catheters can vary on the basis of the structures they are designed to map: the crista terminalis, the His bundle, the coronary sinus, or the pulmonary vein ostium. Typical electrophysiology catheters are shown in Figure 6-3.
Ablation Catheters
Ablation catheters of various designs allow the operator to map and to delivery energy in a very precise manner. The ablation catheters vary with respect to the length of the ablation/tip electrode ranging from 3.5 to 10 mm in length. Figure 6-4 shows commonly used ablation catheters. Notice that the tip of the catheter can be deflected to allow for the arrhythmogenic myocardium to be reached. Conventionally, the tip of the ablation catheter is longer than the electrode of a diagnostic catheter. This is to prevent overheating of the ablation electrode with consequent coagulum formation. Prevention of overheating of the ablation electrode can also be achieved by active cooling with saline irrigation. Catheters that deliver microwave, cryothermal, or ultrasound energy to destroy tissue are also being developed.
Electroanatomical Mapping Catheters
In the mid 1990s, a novel technology termed “nonfluoroscopic electroanatomical mapping” revolutionized the practice of interventional electrophysiology. Electroanatomical mapping systems integrate three functionalities, namely (1) nonfluoroscopic catheter localization in 3D space, (2) 3D display of activation sequences and of electrogram voltage, and (3) integration of this “electroanatomical” information with noninvasive images of the heart, i.e., computed tomography or magnetic resonance images or ultrasound images (image fusion). There are two leading mapping systems available and most laboratories use one or both of these. They are (1) the CARTO system manufactured by Biosense-Webster Inc and (2) the NAVX system manufactured by St Jude Medical Inc.
Carto
In the mid 1990s, a catheter was created that has the appearance of a standard ablation catheter with a magnetic sensor within the shaft near the tip (Biosense-Webster Inc). Together with a reference sensor, it can be used to precisely map the 3D spatial location of the catheter. The electroanatomical mapping system is called the CARTO system and it consists of the reference and catheter sensor, an external ultralow magnetic emitter (Figs. 6-6 and 6-7), and a processing unit. The amplitude, frequency, and phase of the sensed magnetic fields contain information to solve the algebraic equations yielding the precise locations (see Fig. 6-6) in three dimensions (x, y, and z axes) and orientation of the catheter tip sensor (roll, pitch, and yaw). An electrogram can also be recorded simultaneously in space, and thus an electroanatomical map can be generated. The catheter can also be moved without fluoroscopy thus decreasing radiation exposure. An example of atrial tachycardia that was arising from a focal point that was mapped and ablated successfully with the use of the CARTO system is shown in Figure 6-7 for focal tachycardia. A left anterior oblique (LAO) of an electroanatomical map of the right and left atria as well as the coronary sinus is shown. The activation data seen with the color scale show the arrhythmia to arise from the ostium of the coronary sinus.
Localisa and NAVX
This system was initially marketed under the name of Localisa and was subsequently integrated into the NAVX system. It uses three low-amplitude high-frequency current fields that are generated in three axes over the patient’s thorax to compute the position of an electrode in the thorax relative to a reference electrode that can be placed in the heart or on the patient’s thorax. Based on these measurements, the system then displays the position of any electrophysiology catheter (NAVX). The advantage of the Localisa or NAVX system is that multiple catheters can be displayed, and, unlike the CARTO system, they are not limited to the products of a single manufacturer. An example of an LAO cranial view of an electroanatomical map of the left atrium (blue shell) along with the four pulmonary veins and the left atrial appendage (green) constructed with NAVX is shown in Figure 6-8. Also seen are the coronary sinus catheter and the tip of the ablation catheter and a circular mapping catheter. This map was performed in a patient undergoing catheter ablation of atrial fibrillation (AF).
Junction Box and Recording Apparatus
This equipment consists of pairs of numbered multiple pole switches matched to each recording and stimulation channel and permits the ready selection of any pair of electrodes for stimulation or recording. Current computer junction boxes come in banks of 8 or 16. Nowadays, the signal processor (filters and amplifier), visualization screen, and recording apparatus are incorporated as a single unit in the form of a computerized system. The popular systems are manufactured by Prucka (GE), EP Medical (SJM), or Bard. Eight to 14 amplifiers should be available to process surface electrocardiogram (ECG) leads simultaneously with multiple intracardiac electrograms. The number of amplifiers can be as many as 128 in some systems. Intracardiac recordings must be displayed simultaneously with at least three surface ECG leads. Most computers allow several pages to be stored with one page displaying a 12-lead ECG. Thus one can always have a 12-lead ECG recorded simultaneously while the operator is observing intracardiac electrogram data. The amplifiers used for recording intracardiac electrograms must have the ability to have gain modification and alter both high and low band pass filters to permit appropriate attenuation of the incoming signals. For example, the His bundle electrogram is most clearly visualized when the signal is filtered between 30 or 40 Hz (high pass) and 400 or 500 Hz (low pass, Fig. 6-9). In addition, assessing unipolar electrograms also requires acquiring open filters (0.05 to 500 Hz). In our institution, we use a Prucka Cardiolab EP system.
Stimulation Apparatus
Most electrophysiology studies require a complex programmable stimulator that has (1) a constant current source, (2) minimal current leakage, (3) the ability to pace at a wide range of cycle lengths (100 to 2000 ms) from at least two simultaneous sites, (4) the ability to introduce multiple extrastimuli, and (5) the ability to synchronize the stimulator to appropriate electrograms during spontaneous and paced rhythms. The stimulator is equipped with dials or switches by which the pacing intervals and coupling intervals of the extrastimuli may be adjusted (Fig. 6-10). A junction box that interfaces with the recording system and stimulator facilitates changes in pacing site without the need to disconnect catheters. The stimulator should be able to deliver variable currents that can be accurately controlled, with a range from 0.1 to 10 mA. The ability to change pulse widths is also useful. The results of programmed stimulation can be influenced by the delivered current, and, for consistency and safety, stimulation is generally carried out at two and a half times diastolic threshold.
It is preferable that the stimulator, computerized data recorder, and other devices used in EP are permanently installed. In most laboratories, a stimulator and a computer system that modifies all input signals and stores them in an optical disc is used. All equipment must be grounded and other aspects of electrical safety must be ensured because even small amounts of leakage current can pass to the patient and can potentially induce arrhythmias. The equipment must be checked by a technical engineer so that leakage current remains less than 10 mA. A cartoon illustrating the organization of the relevant equipment during an EPS is illustrated in Figure 6-2.
Defibrillator
A functioning defibrillator should be available at the patient’s side throughout all electrophysiology studies. A backup defibrillator is optimal in case of a rare but potentially disastrous failure of one defibrillator. The defibrillators should be tested before each study and equipped with an emergency power source. Many laboratories use commercially available R-2 pads, which are placed on the patient before the EPS procedure is begun. One pad is placed under the right scapula and the other on the anterior chest over the left ventricular (LV) apex and connected to the defibrillator with an adaptor. In rare instances in which transthoracic defibrillation fails to convert induced ventricular fibrillation, emergency defibrillation through an intracardiac electrode catheter may be effective in terminating the arrhythmia (Fig. 6-11). It is our practice to have biphasic defibrillators in our laboratories.
Remote Navigation Systems
With the advent of catheter ablation for AF, the duration of catheter ablation procedures saw a steep rise and operator fatigue became a significant issue. The ability to manipulate catheters in a precise manner during these procedures also began to gain importance. These factors led to the development of technology that allows the physician to manipulate the catheters in a very precise manner reproducibly and from a distance and thus possibly accelerate the workflow. The two leading technologies to achieve remote catheter navigation are the magnetic navigation system developed by Stereotaxis Inc and the robotic navigation system from Hansen Medical Inc. Most dedicated electrophysiology laboratories have acquired one of these two technologies and therefore a familiarity with these is essential.
Magnetic Navigation
Because the working tip of the disposable interventional device is directly controlled by these external magnetic fields, the physician has the same degree of control regardless of the number or type of turns, or the distance traveled, by the working tip to arrive at its position in the blood vessels or chambers of the heart, which results in highly precise digital control of the working tip of the catheter. A picture of the Stereotaxis system from the University of California Los Angeles with one of the floor-mounted magnets is shown in Figure 6-12. Installing a magnetic navigation system requires that the catheterization laboratory be equipped with steel plates and specialized equipment to prevent the magnetic fields from interfering with other equipment.
Robotic Sheath Navigation
The Hansen Catheter Control System is designed to provide precise catheter control and 3D navigation. By bringing together robotic technology with computer movement algorithms, the remote control of catheters is made possible from a physician workstation in a location where radiation exposure is decreased. The advantage of this system is that the system is transportable and does not require construction of a specialized room. A key aspect of the system is an electromechanical catheter manipulator that responds to physician inputs from a workstation. A picture of an operator sitting at a Hansen workstation is shown in Figure 6-13.
Implantation of Pacemakers, Defibrillators, and Resynchronization Therapy Devices
Facilities, Personnel, and Equipment
In the early days, pacemaker implantation was performed by surgeons in the operating room. As the device underwent progressive miniaturization, the procedure could be performed without a thoracotomy and moved to the catheterization laboratory. The skills required also evolved to the ability to cannulate a central vein and the ability to place the lead against the appropriate myocardial location. With the advent of implantable defibrillators and resynchronization therapy devices, the operating physician had to be adept at cannulating the coronary sinus and other coronary venous branches, manipulating “over the wire” leads, and comfortable inducing ventricular fibrillation multiple times during a procedure. The need to perform high-quality coronary venograms in multiple planes reinforced the shift from the operating room to the EP laboratory.
Surgical Type of Sterile Environment
Early concerns about sterility are well founded. A defibrillator or pacemaker is a foreign body that is going to remain in place for several years, and, hence, infection is a primary concern. Generally, the operating room is considered to be a high sterile area and offers the best protection from infection. The catheterization laboratory is considered an intermediate sterile area and is a high traffic area but offers the advantage of high-quality radiography with high-resolution images, multiple projections, and image magnifications, along with the fact that these laboratories are fully equipped with all the necessary catheters, sheaths, and wires that may be required. The right combination for device implantation is a dedicated EP laboratory where the ventilation system meets standards for operating rooms and a rigid protocol for aseptic techniques is followed.
Clinical Evaluations of the Patient before Electrophysiology Procedures
The operating physician must comprehensively evaluate the patient before the study and plan the procedure on the basis of the specific needs of the individual patient. Whenever possible, ECG documentation of the clinical event should be reviewed. Some or all of the procedures listed in Table 6-2 may be included in this evaluation.
Table 6-2 Possible Components of Evaluation Before Electrophysiologic Testing∗
Procedure | Purpose |
---|---|
History and physical examination | |
Neurologic evaluation (if history and physical suggest) | |
Electroencephalogram | Rule out seizure disorder |
Computed tomography/magnetic resonance imaging | Identify focal lesion |
Carotid ultrasound | Identify significant cerebrovascular disease |
12-lead ECG | |
24- to 48-hr ambulatory ECG | |
Event recorder | Correlation of symptoms with ECG events |
Head-up tilt-table testing | Diagnose vasovagal/vasodepressor syncope |
Echocardiogram and radionucleotide ventriculography | |
Stress test (with or without perfusion scanning) | |
Cardiac catheterization | Definition of coronary anatomy |
ECG, Electrocardiogram.
∗ Selected procedures may vary depending on the clinical presentation.
Any potentially reversible arrhythmogenic factors, such as electrolyte abnormalities or decompensated congestive heart failure,
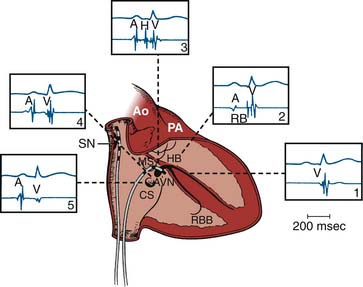
(From Grossman W: Cardiac catheterization and angiography, ed 2, Philadelphia, 1974, Lea & Febiger.)
should be corrected before the study is performed. All antiarrhythmic medications should be discontinued for at least five half-lives before the baseline study. For supraventricular tachycardia studies, medications influencing AV nodal conduction (e.g., beta-blockers, digoxin, and calcium channel blockers) should be discontinued.