Myocardial ischemia can occur through two different pathophysiological mechanisms, in the majority of cases related to the presence of atherothrombosis. These mechanisms are: Other types of ACS–MI will be discussed later in the section on ECG changes due to decreased blood flow not related to atherothrombosis (Table 20.1). There are two types (Myocardial Infarction Type I, Thygesen et al. 2019): Coronarography shows complete or nearly complete thrombotic occlusion in the patient with a ruptured plaque and reduced blood flow (TIMI 0/1). The presence of total or near total occlusion may be intermittent and associated with coronary spasm and/or new thrombosis. However, sometimes after removal of the thrombus, the coronary stenosis is not very significant (<50%). There is complete transmural involvement of at least part of one left ventricular wall, often in myocardium previously relatively unaffected by the ischemia. In general, very little collateral circulation or preconditioning (mechanism of adaptation to ischemia mediated by ATP‐sensitive potassium channels and adenosine) is present (Tomai et al. 1999). The biomarkers are positive, and usually evolve to Q wave MI. According to the guidelines, if the patient presents with angina that has started within the last 12 hours, treatment to open the artery (fibrinolysis or percutaneous coronary intervention (PCI) if possible) should be started immediately in order to avoid or minimize development of Q wave MI (“time is muscle”). Table 20.1 Classification of ECG changes in clinical settings due to myocardial ischemia The ECG shows ST segment elevation usually occurring after a transient period of a peaked, tall, and positive T wave (see Figures 13.5B and 20.4B) (see later and Table 13.1). Although the ST elevation is the most striking ECG manifestation, some leads with ST depression as a mirror pattern are also observed. Correlating the ups and downs of ST is crucial to identifying the culprit artery, the location of the obstruction, and the area at risk in the case of STEMI (see Chapter 13 and Figures 13.38–13.52), and later: ECG changes in ACS due to decreased blood flow (STEMI). These cases occur due to ulcerated plaque often with rupture and, in around 40% of cases, thrombus but with patent artery (non‐complete occlusion). Patients with NSTEMI may present with either elevated biomarkers (non‐Q wave MI or true NSTEMI) or not increased biomarkers (unstable angina). The average diameter of stenosis is slightly larger than in STEMI but the thrombus usually does not occlude the artery completely. However, if the artery is occluded—which occurs in around 20–25% of cases (Dixon 2008; Pride et al. 2010)—there is no complete homogeneous transmural involvement because of the presence of collateral circulation and/or preconditioning. The involvement of left ventricular walls by the ischemia is not completely transmural and occurs usually in the presence of already previous evident impairment of subendocardial flow and increased left ventricular telediastolic pressure. The coronary flow is decreased (TIMI flow 2 or sometimes 3). Table 20.2 Summary of ECG, clinical, angiographic, and pathologic findings in ST elevation myocardial infarction (STEMI) and non‐ST elevation myocardial infarction (NSTEMI) a The rest (20%) are cases of ACS with confounding factors (see text). ACS: acute coronary syndrome; MI: myocardial infarction; NSTEMI: non‐ST elevation myocardial infarction; PCI: percutaneous coronary intervention; STEMI: ST elevation myocardial infarction. Angiographic studies of NSTEMI have demonstrated that compared with STEMI, they are more likely to present with a more spontaneous patent artery, better flow (TIMI > 1), and less median stenosis (Abbas et al. 2004). The culprit artery may be LMT or any of three coronary arteries or their main branches at all levels. Frequently, there is two‐ or three‐vessel disease but usually only one culprit artery. The patient may present with pain upon arrival at the Emergency Department. This depends on whether the coronary flow is sufficiently open (spontaneously or with treatment) for blood to reach the ischemic zone. However, antithrombotic treatment must be urgently applied to stop the progression of the disease. The decision to perform PCI is usually not emergent, especially if pain ceases, but often an urgent, as soon as possible, PCI is recommended if feasible. The ECG changes include ST depression and/or post‐ischemic flat/negative T wave, or even unmodified or normal ECG. Hence, the name non‐ST elevation acute coronary syndrome (non‐STEMI) (Thygesen et al. 2019). However, ST elevation is often present in some leads, especially in aVR and V1 (mirror pattern) (see further discussion about all the ECG changes found in NSTEMI). In the following sections, ECG changes in various clinical settings will be fully discussed. We know that some aspects have already been mentioned, but we consider that it is convenient to reinforce its importance. In the past, many cases of STEMI evolved to an MI, which was usually of Q wave type (Figures 20.1A). If modern treatments to open the artery are available, more Q wave MIs can now be aborted (see Figure 20.1B). The typical ECG pattern is ST segment elevation. Other patterns may also be seen (see later). The ST segment elevation criteria for ischemia according to the new universal definition of MI (Thygesen et al. 2019) includes New ST elevation at the J‐point in two contiguous leads with the cut‐point: ≥1 mm in all leads other than leads V2–V3 where the following cut‐points apply: ≥2 mm in men ≥40 years; ≥2.5 mm in men <40 years, or ≥1.5 mm in women regardless of age. This type of ST segment elevation of these characteristics is considered abnormal and evidence of acute ischemia in the clinical setting of ACS (presence of precordial pain or the equivalents). Whenever possible, it is important to compare this pattern with previous ECGs (Table 13.1). Table 20.3 ECG patterns in ST elevation myocardial infarction (occluded/nearly occluded artery with homogeneous transmural involvement) (see text) a Based on our experience (Sant Pau Hospital, Barcelona) with the ECG at arrival to Emergency Department (≈30% STEMI, 50% NSTEMI—see Table 20.4—and the rest ≈20% ECG with confounding factors (Fiol‐Sala et al. 2020). ACS: acute coronary syndrome; MI: myocardial infarction. The morphology of the ST segment elevation may show a concave or convex shape with respect to the isoelectric line or just a rectified line. Often, the shape of the morphology changes at follow‐up with a greater concave shape after a hyperacute period. In this phase, the height of the ST elevation may be considered, causing the S waves to be pulled up (Figure 20.2C). This decreases during the subacute phase (Figures 13.32 and 13.34). The evolution of ST segment elevation, if the MI is not treated, leads to Q wave MI with a progressive resolution of ST elevation. It is considered MI if the ST remains elevated after 20 m. The degree of ST elevation rapidly reaches a peak elevation that usually occurs at 1 hour after the onset of pain. The plateau last up 12 hours. Afterward, a progressive decline takes place that lasts a few days. In the pre‐fibrinolytic era, complete resolution was found in 95% of cases of inferior MI and in 40% of cases in anterior MI after two weeks (Mills et al. 1975). Currently, the course is very different and the resolution of ST elevation is often very rapid with fibrinolysis and especially PCI (see Figure 20.1). The ST resolution is accompanied by the progressive appearance of Q wave and T wave inversion indicating transmural involvement, or at least involvement of some layers of left ventricular wall outside the subendocardium (see Figures 13.32 and 13.61). In fact, the appearance of a negative T in the evolving process of Q wave MI is due to the LV intraventricular pattern (window phenomenon of Wilson) (Figures 13.6E and 13.61). The ST may remain slightly elevated even in the chronic phase. Sometimes this ECG pattern of Q wave MI diminishes or even disappears (see Figures 13.32 and 20.35). Figure 20.1 Comparison of the evolution of an STEMI of the anteroapical zone in 1970 before thrombolysis and percutaneous coronary intervention (PCI) and currently. In 1970, from A to D, we may see the evolution to a large Q wave myocardial infarction. In 2010, the patient with STEMI (A) is submitted to successful PCI (B), and a post‐ischemic negative T wave appears. Later on, (C) the patient presented with pain again and the ECG pseudonormalizes. A new coronarography demonstrates thrombosis of the stent and new PCI solves the problem, and again a negative T wave appears (D). The Q‐wave infarction was aborted. Figure 20.2 (1): The three types of repolarization abnormalities that may be seen in an acute phase of myocardial infarction involving the inferolateral zone: (A) tall and/or wide T waves in inferior leads; (B) abnormal ST segment elevation, with no changes of the final part of QRS; (C) important ST segment elevation and distortion of the final part of QRS. (2): The three types of repolarization abnormalities that may be seen in an acute phase of myocardial infarction involving the anteroseptal zone: (A) tall and/or wide T waves especially seen in right precordial leads; (B) abnormal ST segment elevation, with no changes of the final part of QRS; (C) important ST segment elevation and distortion of the final part of QRS (see text). ST elevation prevails in the leads facing the affected zone, producing a direct ECG pattern. In some opposing leads, the ST segment depression is seen as an indirect mirror ECG pattern (see Figures 13.35–13.37). These same features also occur in the chronic phase, with a Q wave producing the direct pattern and an R wave in V1–V2 as the mirror pattern (see Figure 13.73). In STEMI, the global evaluation of direct and reciprocal changes (ups and downs of ST) is important for detecting which artery is the culprit (the RCA or the LCX in case of ST elevation in II, III, and aVF) (see Figure 13.39), as well as to find the location of the LAD occlusion in cases of ST elevation in the precordial leads (Figure 13.38). An ST segment elevation may be observed as a normal variant in V1–V2, especially in males including 1–2 mm of ST elevation with an slightly convex shape with respect to isoelectric line, an ascending ST slope, and asymmetric T waves (Figure 7.13H). ST elevation may also be seen in many other normal situations such as in athletes or thoracic abnormalities (pectus excavatum) (see Figure 7.16). Abnormal ST elevation due to other causes may be seen in Table 13.4 and Figure 13.59. Figures 20.3 Patient with STEMI and very bad hemodynamic status in cardiogenic shock, due to complete occlusion of the left main trunk (LMT). Observe the right bundle branch block (RBBB) with superoanterior hemiblock (SAH) and the ST shifts with ST elevation in I, aVL, and from V2 to V6, and ST depression in II, III, aVF. V1 and aVR are isoelectric due to the influence of LCX involvement that counteracts the ST elevation in V1 and aVR that usually is seen in case of proximal to S1 LAD occlusion (except in case of great conal artery—see text). The following ECG patterns without ST elevation may be noted as the most striking ECG change in patients with the clinical ECG angiographic syndrome of STEMI (occluded or near occluded coronary artery with transmural involvement). We have discussed previously the great importance of recognizing this pattern as a case of STEMI (see mistakes in the interpretation of ACS). In some phases of STEMI, ECG patterns other than ST elevation may be seen, including the following: Figure 20.4 Atypical patterns of STE‐ACS: Pattern A: STEMI equivalent. In the case of LCX occlusion (involvement of LCX). Pattern B: Three patterns of tall and wide T wave as a first sign of ischemia. B‐1: Transient pattern followed by ST elevation (STEMI) after a few minutes. B‐2 and B‐3: Examples of persistent tall T wave (several hours), before complete occlusion and transmural involvement evolving to Q wave MI (see text). Pattern C: deep negative T wave when the pain disappears in case of STEMI (post‐ischemic change). However, the pain may appear again (new occlusion due to new thrombus or coronary spasm), and the T wave first pseudonormalizes and later evolves to ST elevation. Figure 20.5 (A) ECG recorded during chest pain in a patient with acute occlusion of the LCX coronary artery. No significant lesions were present in the other coronary arteries. The ECG shows ST depression in leads V1–V4 and only minor ST elevation, not fulfilling (STEMI) criteria, in leads I, aVL, and V6. (B) A similar case in which ST depression in many precordial leads is seen (V1–V5) with mild ST elevation in II, III, aVF. The recording of V7–V9 visualizes the STEMI better. However, it may be diagnosed already by 12‐lead ECG and corresponds to true STEMI equivalent. Therefore, patients with deep negative T waves in the right precordial leads who have had anginal pain in the hours previously should be submitted to coronarography as soon as possible. This situation is not, however, considered an emergency, unlike the case of a clear ST elevation, because the presence of a deep negative T wave indicates that the myocardial tissue is at this moment more or less perfused (open artery). Furthermore, once the acute phase has passed, this pattern may remain for a long time, even years, without requiring urgent study. The resolution of the patterns to a normal positive T wave, however, is a sign of good prognosis (Tamura et al. 1999). Recently, it has been demonstrated in a few cases by correlation with cardiovascular magnetic resonance (CMR) that in these cases of deep negative T waves in right precordial leads considered an atypical pattern of STEMI (Figure 20.4C), there is transmural evidence of myocardial edema in certain parts of the left ventricular wall (Migliore et al. 2011) (Figure 13.7E). Figure 20.6 Two cases of STE‐ACS with persistent pattern of tall T wave in V1 to V3–V4 in (A) without evident ST shifts (as cases of Sagie et al. 1989), and in (B) with some ST depression in many precordials (as cases of Dressler and Roesler 1947 and De Winter et al. 2008). Both present with proximal occlusion of the LAD without important collateral circulation and non‐important involvement in RC and LCX. The implantation of one stent in LAD proximal solves the problem in both cases but the patient of case B finally presented with a QS pattern of myocardial infarction in right precordials (see below). Figure 20.7 Left: Patient with acute chest pain and evolving anterior wall myocardial infarction. (A) During prolonged chest pain showing positive peaked T waves without ST segment elevation. (B and C) ECG recorded 12 hours (ST elevation) and 24 hours (negative T wave) later. (D) ECG one week later with QS and deep negative T wave (Sagie et al. 1989. Reproduced with permission from the American College of Chest Physicians). Right: Patient with acute chest pain. (A) Three hours after the onset of chest pain. See ST depression plus very tall T wave. (B) Changes in QRS (QS and ST elevation) were not present until 18 hours after the onset of symptoms. (C and D) Progressive inversion of the previously high T waves (Reproduced with permission from Dressler and Roesler 1947) (see text). Figure 20.8 Top panel. ECG corresponding to a patient who reports prolonged anginal pain before hospital admission but who is currently asymptomatic. It is a typical post reperfusion pattern of the long anterior descending artery (the negative T wave also affects the inferior wall). Lower panel. ECG recorded during pain recurrence. An occlusion pattern of the anterior descending artery is observed. In the case that STEMI evolves to Q wave MI, obviously the pattern of deep negative T wave is observed, but with QS pattern and the negativity of T wave is due to recording of LV intraventricular pattern (see before) (Figure 13.6E). It is important to remember that without clinical information, even expert cardiologists will have problems in diagnosis of some cases of ST elevation (Jayroe et al. 2009). One of the most important problems is related to differential diagnosis of the asymptomatic cases with mild ST elevation and often with “r” in V1–V2. These cases may be seen in the Brugada syndrome, pectus excavatum, and in athletes (Figure 7.16). The characteristics of the ST elevation and mirror patterns are relevant when localizing the occlusion and area at risk, quantifying of the ischemic burden, and detecting the grade of severity of ischemia. Many authors (Birnbaum et al. 1993; Engelen et al. 1999; Tierala et al. 2009; Kanei et al. 2010), in addition to our group (Fiol et al. 2004, 2009, 2020) among others, have demonstrated the usefulness of ECG‐coronarography correlations to locate the place of culprit artery and the site of occlusion. This has been discussed in detail in Chapter 13. Figure 13.38 shows the algorithm for predicting the LAD occlusion site in cases of an ACS with STE in the precordial leads and Figure 13.39 represents the algorithm to follow in cases of ACS with STE in the inferior leads to distinguish between RCA and LCX occlusion. Figures 13.40–13.52 show examples of different ECG patterns according to the coronary artery involved and the site of the occlusion. In our experience, these ECG criteria have high statistical value (see Figures 11.37 and 11.38), as has also been demonstrated by other investigators for the same or other algorithms (see Chapter 13). Usually, the involvement of the LMT is expressed by subocclusion of the artery because acute complete occlusion in general rapidly triggers cardiogenic shock and ventricular fibrillation, and the patient dies before reaching the Emergency Department. However, some cases of complete occlusion of the LMT if arrive at Hospital very early may survive. Fiol et al. (2012) have documented 9 patients, 8 with ECG of STEMI, and 1 resembling the ECG of LMT subocclusion as a result of great collateral circulation. Four survived (Figure 20.3). The ECG pattern of STEMI is similar to proximal occlusion (before S1) of very long LAD, often with right bundle branch block (RBBB) and ST elevation in precordials and leads I and aVL, and ST depression in the inferior leads. Usually, in V1 and aVR, there is no ST elevation due to the involvement of LCX that depresses the ST in these leads and compensates the ST deviation (Figure 20.3), that usually is found in case of proximal LAD occlusion (see later), except in cases of large conal artery that also perfuses the high septum (Ben‐Gal et al. 1997). In LMT occlusion, the hemodynamic state is poorest, especially in the absence of very dominant RCA. All these criteria, that allows us to localize the culprit artery and even the place of occlusion in LAD, RCA, LCX, and LMT, have some limitations (Huang et al. 2011) that are a consequence of the varied anatomy of the coronary arteries, the cancellation of ST vectors, the quick change of location of occlusion during treatment, and also in cases of LAD occlusion the incorrect placement of precordial electrodes because the ECG pattern may change from one location to another (see Figure 13.79). Therefore, before trying to localize the culprit artery with the ECG, we have to take into account the following considerations: Although the location of the occlusion may indicate the approximate area at risk, an understanding of how to quantify the ischemic burden and calculate the size of area at risk using different risk scores is important. ST deviations are an important parameter (TIMI score, PREDICT score, GUSTO score, GRACE score, etc.) (Fiol‐Sala et al. 2020). A score based on the percentage of left ventricle area infarcted according to Selvester score has also been reported (Aldrich et al. 1988). The TIMI risk score is the most commonly used system for prognosis and includes ST deviations as one of the parameters to be assessed (Antman et al. 2000; Morrow et al. 2000). The score derived from the GUSTO trial (Hathaway et al. 1998) for estimating 30‐day mortality from initial clinical and ECG variables shows that despite the fact that some limitations exist, as seen in proximal RCA occlusion that may mask the spread of ischemia because of isodiphasic ST present in V1, the sum of the ST changes in all leads may estimate the degree myocardium at risk. More than 15 mm of ST deviation usually represents an important area at risk. Figure 20.9 (A) Left: ECG of a patient in hyperacute phase of acute coronary syndrome (ACS) with great involvement of anteroseptal zone. Observe ST segment elevation from V1 to V5. Right: After an hour of fibrinolytic treatment, the area at risk decreases significantly, being limited exclusively to septum (ST segment elevation from V1 to V2 with ST segment depression in V5–V6). (B) In a chronic phase, the patient presented with an anteroapical infarction. The morphology of QRS‐ST may indicate the degree of ischemia. According to the Birnbaum–Sclarovsky ischemic grading system (Birnbaum et al. 1993), grade I ischemia presents with a tall persistent T wave, generally without mild ST depression; grade 2 presents with an ST elevation without distortion of the QRS complex; and grade 3 presents with an ST elevation that sweeps the QRS complex upward and provokes a distortion of it and with ratio J point/R wave >0.5 (see Figure 20.36) (Figure 20.2). The cases described by De Winter et al. (2008) that also present with a tall T wave as the most evident ECG change (predominant subendocardial involvement) are most likely more than grade I of ischemia. The presence of associated mild ST depression that often occurs before the tall T wave may be due to a higher grade of subendocardial ischemia than just tall T waves (Sagie et al. 1989). The in‐hospital prognosis of both STEMI and NSTEMI has improved considerably with new thrombolytic and antiplatelet drugs and primary PCI. In both types of ACS, the GRACE score based on clinical ECG and blood tests (Eagle et al. 2004) has been recommended for risk stratification and the evaluation of best management. We will now summarize the most important ECG parameters that are useful for global prognosis. When an STEMI occurs in clinical practice, a critical occlusion has often developed in just one culprit artery although in many cases, multivessel disease is present. There is no general agreement how to proceed, but it seems the best approach is to open only the culprit artery in the first procedure (Widimsky and Holmes 2011). However, in the case of hemodynamic impairment or the presence of more than one critical occlusion, opening more than one artery may be indicated. What is most important in the catheterization laboratory, when faced with STEMI and multivessel disease, is that the interventional cardiologist can, thanks to the correct and quick interpretation of the ECG, correctly select the coronary arteries in which the PCI must be performed. With the coronaroangiographic results at hand, the ECG provides important information for the identification of the culprit artery in these cases. Unfortunately, this information is often underused in clinical decision‐making (Nikus et al. 2004). Figure 20.10 shows one example of the relevance of this approach. Figure 20.10 Case of right coronary artery (RCA) occlusion (ST depression, ST elevation III > II). However, there is ST depression from V1 to V6 that is unusual in isolated RCA occlusion. This suggests LAD involvement (95% proximal to D1). Therefore, in this case, it is advisable to perform double percutaneous coronary intervention (PCI) (RCA + LAD), although the culprit artery is the RCA because the LAD has a critical occlusion. Close collaboration between clinicians, experts in ECG, and interventional cardiologists should be emphasized. Nowadays, with modern technology, it is possible to obtain an expert opinion on an ECG interpretation from a distance in seconds (Leibrandt et al. 2000). The transmission of ECGs directly to a consulting center with webbrowsing capabilities may facilitate immediately starting a revascularization procedure in the appropriate culprit artery (Adams et al. 2006; Sejersten et al. 2008; Ting et al. 2008; Ducas et al. 2012; Al‐Zaiti et al. 2013; Kerem et al. 2014). During fibrinolysis, the most typical ECG changes are the following: The most typical ECG changes are the following: Figure 20.11 A 45‐year‐old man with occlusion of the posterior descending coronary artery. During the PCI procedure, intraluminal thrombus and the appearance on the 12‐lead‐ECG of accelerated idioventricular rhythm with frequent fusion beats. Note how in the narrower QRS complexes there is elevation of ST‐T in II, III, aVF and a mirror image in V1–V3. Figure 20.12 (A) Single ECG complex from lead V2 obtained under baseline conditions, before the start of percutaneous coronary intervention (PCI) of the left anterior descending coronary artery (LAD). (B) Single ECG complex from the same lead shown in (A) is displayed 25 sec after balloon inflation in the proximal LAD. The QT interval is seen to prolong by 44 ms. (C) The two complexes seen in (A) and (B) are superimposed to better illustrate ischemia‐induced changes showing prolongation of QT interval and symmetric T wave (Reproduced with permission from Kenigsberg et al. 2007). Figure 20.13 This patient presents with a negative T wave just during percutaneous coronary intervention (PCI) that pseudonormalizes the T wave during the inflation period (see text). Figure 20.14 ECG recorded on precordial leads and intracoronary ECG that shows the small changes of T wave in precordial leads (see after inflation 1, peaked T wave that after successive inflations is less evident). However, the decrease of ST changes due to preconditioning after several inflations is much better seen in intracoronary ECG (IC‐ECG) (Reproduced with permission from Koning et al. 1993). Obviously, all atypical cases of STEMI that present with non‐STE changes have to be excluded (see Figure 20.4). The depth of the negative T wave in atypical ECG patterns of STEMI, especially in case of LAD occlusion, is usually much more significant than in NSTEMI (compare Figures 13.11 with 13.12 and 20.15). According the new universal definition of MI (Thygesen et al. 2019), the ECG manifestations of acute ischemia in patients with NSTEMI include a new horizontal or downsloping ST depression ≥0.5 mm in two contiguous leads and/or new T wave inversion ≥1 mm in two contiguous leads with a prominent R wave, or R/S ratio > 1 and/or negative T wave in two or more contiguous leads (see Table 13.1). We would like to make some comments on this statement (see also Chapter 13, Changes of repolarization: T wave). We consider two types according to the number of leads with ST depression: extensive involvement and regional involvement. Table 20.4 ECG patterns in non‐ST elevation myocardial infarction (50% of ACS). Non‐STEMI: non‐occluded artery and non‐transmural involvement. Without pain, the ECG may change sometimes dramatically and even may be normal (Figure 20.17) Figure 20.15 A 46‐year‐old patient with previous precordial pain. The ECG (B) without pain showed very discrete changes in V2–V3 leads (slightly negative U wave with somewhat positive T wave) (see V2–V3). These small changes are significant when compared with the previous ECG taken few months ago (A). The exercise stress test was positive and the coronary angiography showed proximal LAD stenosis resolved by percutaneous coronary intervention (PCI). The subsequent ECG was the same as the initial one (A). Figure 20.16 (A and B) Changes of ST/T during angina crisis in the case of left main trunk (LMT) subocclusion (A) and severe three‐vessel disease (B). See the differences in (A) and (B) before and after pain. It is evident, especially in LMT subocclusion, that the increase of ST depression is accompanied by negative or nearly negative final T wave. Figure 20.17 (A) ECG without pain is practically normal. (B) ECG during pain shows ST segment depression and inverted T waves in more than eight leads, maximally in leads V4–V5 where there is no positive T wave and ST segment elevation >1 mm in lead aVR (circumferential subendocardial involvement). (C) Coronary angiography shows tight stenosis in the left main coronary artery before and after primary percutaneous coronary intervention (PCI). This ECG pattern may be seen in LMT subocclusion, three‐vessel disease subocclusion, and isolated but tight and proximal LAD and rarely in proximal subocclusion of large LCX. The ST depression is seen especially in V3–V6 and I, II, and aVF and/or aVL; ST elevation in aVR exists in severe LMT/3VD, usually >1 mm (Figures 20.16–20.20). If there is an increased heart rate, the upsloping ST depression may be influenced by the tachycardia (Birnbaum et al. 2011). Although there is no definitive statement clarifying the culprit artery in the case of diffuse ST depression in >7 leads with ST elevation in aVR, we suggest, based on recent papers (Kosuge et al. 2011) and our experience, the following (Table 20.4). Figure 20.18 The ECG taken during pain shows ST segment depression not very prominent (<2 mm) in V2–V6 and I and aVL but with clear (>1 mm) ST elevation in aVR. The patient presented with severe left main trunk (LMT) subocclusion. The ECG after pain shows flat T wave in leads with previous ST depression. Figure 20.19 The ECG of a patient with an NSTE‐ACS and three‐vessel disease with proximal subocclusion of LAD and LCX that shows during angina a huge ST segment depression in many leads more prominent in precordial leads V3–V5 with tall positive T wave and with ST segment elevation in aVR and V1. This case is similar to that shown in Figure 20.21, but the presence of ST elevation in aVR > 1 mm in this case is in favor of proximal three‐vessel disease. Figure 20.20 (A) ECG showing diffuse ST segment depressions with positive T waves in the inferior leads II, III, and aVF and in leads V2–V6 besides ST segment elevation in lead aVR. ST elevation in lead aVR not >1 mm. The ST segment in lead V1 cannot be reliably analyzed because of technical artifacts. (B) Right coronary artery with a large posterior descending (PD). No significant stenoses are present. (C) Left anterior oblique caudal angiographic view shows a very tight stenosis in the proximal LAD (arrow). (D) Discharge ECG of the patient showing Q wave in V1, V2, and inverted T waves in leads V2–V6 and in leads I and aVL. Probably the patient presented with STEMI with finally residual “q” in V1–V2 and a deep negative T wave in V2–V5 due to final transmural involvement (Adapted with permission from Gul and Nikus 2011). Table 20.4 shows all these patterns. However, in spite of all these considerations, their predictive accuracy still has to be confirmed in a larger trial. In cases of regional involvement, the culprit artery is in general a subocclusion of a proximal short LAD, distal LAD, or LCX/RCA. However, sometimes two‐ or three‐vessel disease is responsible. Table 20.4 shows the three groups of pattern that may be found: In all the cases of regional involvement, it is practically impossible to identify the culprit artery with high predictive accuracy (Figures 20.23–20.25). The negative T wave is usually not deep (<2 mm) and is not diffuse. It is more frequently seen in leads with a dominant R wave (Figure 20.26A) but may be also seen in V1–V3 (rS pattern) (Figures 13.12 and 20.15). This type of T wave may become an ST depression with pain or exercise test (Figure 20.25B). As previously mentioned, the prognosis is better than in cases of ST depression because it usually appears after the cessation of pain (post‐ischemic change). If pain reappears, the prognosis depends on whether the ECG pattern remains unmodified (better prognosis) or an ST depression appears. However, non‐emergency PCI is recommended in the presence of a T wave of these characteristics in the subacute phase of ACS because a coronary subocclusion often exists that sometimes may be tight (see above) (Figures 13.12 and 20.15). In general, biomarkers are negative or in resolution. Figure 20.21 ECG of a patient with NSTE‐ACS with ST depression in seven leads (V3–V6, I, II, aVF). This corresponds to the type D of Table 20.4 that is usually seen, as in this case, due to proximal subocclusion (95%) of the large left circumflex artery (LCX). Figure 20.22 Patients of the Triton TIMI 38 trial presenting with isolated ST depression in V1–V4. Note the type of occluded artery, TIMI flow, and troponin levels (positive or negative) and presumably the ACS type, according to the results of the study (Adapted from Pride et al. 2010). The information about the presence or not of final positive T wave in the presence of ST depression and in which leads ST depression is more evident is lacking. Probably the cases of LCX or RC total occlusion are true STEMI and need emergent PCI. UA: unstable angina. Sometimes in case of NSTEMI, the flat/mild negative T wave is accompanied by a negative U wave (Figure 20.15). Figure 20.23 Patient with NSTE‐ACS with ST depression in <7 leads. In this case, it is difficult to identify the culprit artery that is the right coronary artery (RCA) (95% occlusion). If a patient who has presented with precordial pain arrives within a few hours at the Emergency Department with a very deep negative T wave without Q wave MI, and with normal or borderline biomarkers, but without pain at entrance, the most probable explanation is that it corresponds to atypical pattern of STEMI and not to an NSTEMI (Figure 20.26A). Sometimes, if pain reappears, the negative T wave pseudonormalizes and negative U wave may appear (Figure 20.26B–D). A patient with ACS and a normal ECG at entrance can later evolve to a clear ACS even with LMT subocclusion (Figure 20.17). The ECG may remain normal in 5–10% of cases during an ACS even during crisis of pain. Sometimes the changes of T wave are very subtle, and may be advisable to compare, if possible, with previous ECG (Figure 20.15). The cases that maintain a normal ECG usually have a good prognosis. Abnormal ST depression may be seen in many other circumstances (see Table 13.5 and Figure 13.60). Flat or usually mild negative T waves may also be seen under many other conditions (see Table 13.3 and Figure 13.16). It is important to emphasize that except in cases of perimyocarditis, in which the affected zone may be limited to the subepicardium, in cases such as ischemia or other causes, the ECG pattern of flat/negative T waves is a result of a transmural affected area of the left ventricle presenting with a delayed transmural repolarization (longer TAP) compared with the rest of the left ventricle (see Figures 13.6F and 13.7D). Figure 20.24 In this case that is similar to Figure 20.24, the culprit artery is 95% proximal occlusion of the left circumflex artery (LCX). Figure 20.25 (A) ECG without pain. (B) ECG during pain in the course of NSTE‐ACS. During pain, the ECG shows ST‐segment depression (<3 mm) in V2–V6, I, and aVL. As there is no significant ST‐segment depression in II, there is no ST elevation in aVR. (C) Coronary angiography shows three‐vessel disease (left: RCA, right: left coronary artery). The most important ECG signs of risk are as follows: Figure 20.26 (A) Basal ECG (V1–V6) with ECG pattern of very deep negative T wave in a 65‐year‐old patient with daily crisis of variant angina that always appeared at the same hour. During a crisis (B, C), there is pseudonormalization of the ST segment with an evident negative U wave. A few seconds later, the ECG returns to the original situation (D). The five morphologies in the fourth strip are samples taken minute by minute, with a total duration of pain of 5 min that shows the changes in V3 from positive, T negative U wave during pain to negative T wave after the crises. The ECG signs of low risk are as follows: We have explained that the ECG changes of STEMI are due to transmural homogeneous involvement usually with a completely occluded artery and that the ECG findings of NSTEMI are due to incomplete transmural homogeneous involvement with usually non‐total, although it may be tight, occlusion. If, for any reason, a patient with predominant ST depression due to NSTEMI, often with positive terminal T wave, presents spontaneously (acute thrombosis, coronary spasm, or occlusion of a stent) with total occlusion and there is no collateral circulation/preconditioning, an absolute transmural involvement exists and the ECG pattern changes from NSTE to STE (see Figure 20.27). In fact, in the first minutes of STEMI, the only ECG change is a tall/wider T wave that evolves quickly to ST elevation (transmural homogeneous involvement). However, sometimes the subendocardial involvement that is important persists because the artery, usually the LAD, is not completely occluded, and the ECG not only presents with a tall T wave but also some ST segment depression. We have already mentioned that this pattern, which was described many decades ago by Dressler and Roesler (1947), usually evolves to STEMI (Figure 20.6B). We know that this change from NSTEMI to STEMI occurs when transmural and homogeneous ischemia exists (Figures 20.6B, 20.7, 20.20, and 20.27). In contrast, sometimes a patient with ACS presents in the first phase with an ECG pattern of STEMI (Figure 20.28) (tall T wave in V1–V3 and ST elevation in I, aVL, and V2). In the first instance, only antithrombotic treatment was given and the patient was sent to a reference center. On arrival, the treatment has partially opened the artery and the tall T wave in the precordials is now converted to clear ST depression (NSTEMI). Finally, the artery is opened with PCI and the ECG normalizes, showing only a mild negative T wave (Figure 20.28). Figure 20.27 (1‐A) ECG taken in a patient with NSTEMI and three‐vessel disease. (1‐B) Occlusion of RCA + LCX + LAD distal to big diagonal. Note ST depression from V3 to V6, I, II, aVF with a final positive T wave that is very tall in V3–V5 and ST elevation >1 mm in aVR (see Table 20.4). (2‐A) ECG recorded during chest pain 18 hours after stent implantation in LAD. Note ST elevation in V1–V5 consistent with STEMI due to LAD occlusion (stent thrombosis), as demonstrated by coronarography (2‐B) (see arrow) (Reproduced with permission from Stankovic et al. 2011). These cases correspond to 20% of all ACS. In cases of LVH with a strain pattern and/or wide QRS complex, the diagnosis of ACS is more difficult, especially if concurrent with NSTE‐ACS. Performing the recording of a sequential pattern may be useful (see Figure 13.58). The presence of bundle branch block in patients with ACS is not frequent (≈2–3%), and usually indicates a bad prognosis, mostly LBBB. This is especially true: (i) when the bundle branch block has developed during the ACS; (ii) when the duration of QRS is very wide; (iii) if ST segment elevation resolution is delayed; and (iv) if the ST deviations are very striking. The presence of new LBBB is less frequent than RBBB because the left bundle presents with double perfusion (LAD + LCX). In contrast, the right bundle receives perfusion only from the first septal branch. Therefore, an appearance of RBBB is a sign of a proximal LAD occlusion in the clinical setting of ACS. Figure 20.28 (A) A 59‐year‐old patient with acute coronary syndrome (ACS) and oppressive chest pain that lasts for more than 1 hour. The ECG corresponds to a first‐stage STEMI (ST elevation in I and aVL and tall wide T wave from V2 to V5 of the hyperacute phase). The patient received antithrombotic treatment and was sent to reference hospital for percutaneous coronary intervention (PCI). On arrival (B), the patient still presented with precordial pain of lower intensity and the ECG has changed very much. Now the ST in I and aVL is only mildly elevated and in the precordial leads, there is a pattern of NSTE similar to the ECG pattern that may be seen in isolated tight subocclusion of LAD (ST depression V2–V5). In fact, the patient presents with tight subocclusion of the big first diagonal with LAD involvement that has been partially opened after treatment. The ST depression in the precordials means that at this moment there is no any part of the left ventricular walls with transmural involvement and that predominates subendocardial involvement (NSTEMI). Finally, after PCI, the ECG only presents negative T waves (C). In the presence of RBBB, the diagnosis of STEMI is possible in a similar way to that in normal situations. RBBB does not interfere with the diagnosis of acute STEMI, and the ECG criteria for STEMI are the same as for patients with a narrow QRS. Diagnosing ST elevation in the anterior, inferior, and lateral leads can easily be done in patients with RBBB. However, the diagnosis of inferolateral STEMI equivalent in patients with RBBB and baseline ST depression in V1–V3 is a challenge, and prospective studies are needed to define the threshold for ST deviations in this setting. Remember that in the presence of RBBB, the presence of symmetric negative T wave in precordial leads is always suspicious of abnormality (Figure 20.29). Figure 20.29 A 46‐year‐old man who presented to a health center for chest pain that started one hour before (at 9 AM). An ECG sequence is shown before reperfusion therapy. The indication of coronary angiography is in the second ECG that shows inferior ST‐segment elevation (mainly in III) with reciprocal ST depression in I and aVL and appearance of RBBB with a wide symmetric T wave in the precordial leads. New RBBB is not commonly seen with RCA infarction, unless this is rate dependent. In some cases, the ECG sequence may end with the appearance of Q waves without a clear ST‐segment elevation being observed. Coronary angiography shows a proximal total occlusion of the LAD (arrow) and diffuse disease in the LCX. In the LCX, there is also sub‐total occlusion at the origin of the OM branch (star), which received collateral circulation from the LAD. Upon occlusion of the LAD, ischemia also appeared (elevated ST) in the territory irrigated by the LCX. The left bundle branch (LBB) is a larger and less vulnerable structure than the RBB. The blood supply to the LBB is clearly of dual origin as the anterior fascicle is supplied mainly by anterior septal branches of the LAD and the posterior fascicle from the AV nodal artery, which originates from the RCA or the LCX depending on coronary artery dominance. Accordingly, severe two‐ or three‐vessel disease should typically be present for a new LBBB to develop. Sgarbossa et al. (1996) introduced three criteria to help in identifying acute MI in patients with LBBB (Sgarbossa et al. 1996). They were: (i) ST‐segment elevation ≥1 mm and concordant with the QRS complex (5 points); (ii) ST‐segment depression ≥1 mm in leads V1, V2, or V3 (3 points); and (iii) ST‐segment elevation ≥5 mm and discordant with the QRS complex (2 points). A total score of three or more points was considered diagnostic of acute MI, whereas a score of 2 points suggests an acute MI. A meta‐analysis reported the Sgarbossa criteria to be specific (98%) but with low sensitivity (20%) (Jain et al. 2011; Tabas et al. 2008). ST‐segment elevation has proved to be the single‐most specific criterion for the diagnosis of acute MI in the presence of LBBB, and improves the detection of this “STEMI equivalent” (Larson et al. 2007; Lopes et al. 2011). An adaptation of the Sgarbossa criteria, including ST elevation to S‐wave ratio may be too complicated for use in the emergency setting (Smith et al. 2012). In a study from the Minneapolis Heart Institute STEMI protocol (n = 3903), new or presumably new LBBB was present in 3.3% of the patients (Pera et al. 2018). The authors tested a hierarchical algorithm based on hemodynamic instability and Sgarbossa concordance criteria. The algorithm yielded high sensitivity (97%) and negative predictive value (94%) for identification of a culprit lesion, while specificity was 48%. Another study from the United States reported new or presumably new LBBB in 69/802 (8.6%) in the hospital primary PCI laboratory activation database (Mehta et al. 2012). Less than 30% of the patients with new or presumably new LBBB had troponin elevation, and 54% underwent emergent coronary angiography. Of these, 22% had a culprit vessel occlusion; the emergent revascularization rate was 11.6%. The fourth Universal definition of myocardial infarction (Thygesen et al. 2019) states that in patients with LBBB, ST‐segment elevation ≥1 mm concordant with the QRS complex in any lead may be an indicator of acute myocardial ischemia. The conclusion was that since the detection of ischemia by the ECG in LBBB is difficult, decisions concerning urgent reperfusion therapy should be based mainly on symptoms and hemodynamic parameters. The presence of concordant ST‐segment elevation (i.e. in leads with positive QRS deflections) appears to be one of the best indicators of ongoing MI with an occluded infarct artery. Patients with a clinical suspicion of ongoing myocardial ischaemia and LBBB should be managed in a way similar to STEMI patients, regardless of whether the LBBB is previously known. It is important to remark that the presence of a (presumed) new LBBB does not predict an MI per se. In contrast, the diagnosis of concurrent NSTEMI in the presence of confounding factors may be even more difficult because there are no visible changes in the ECG, or the changes may be confused with basal ECG changes (see Figure 13.58). In our opinion, the most frequent mistakes made in the interpretation of ECG in the course of ACS are the following: In the hiperacute phase of ACS, it is very important to perform the differential diagnosis between STEMI (LCX occlusion) and NSTEMI (LAD subocclusion) in the presence of ST depression in V1–V3–V4. In the first case (LCX occlusion), it is an emergency to activate the catheterization laboratory. The ECG usually solves the problem because in case of LCX occlusion presents ST depression already in V1, and there is not final positive T wave (or very small). On the contrary, in cases of LAD subocclusion, the ST depression in V1 is mild and in V2–V4, there is final evident positive T wave (see Figures 20.5 and 20.20). During the evolving process of LCX occlusion, a RS pattern with less ST depression and final positive T wave appears as a mirror pattern of lateral wall involvement. Figure 20.30 The importance of acute ischemia is a trigger of sudden death: clinical ECG, angiographic, and pathological evidence (see text) (Adapted from Marcus et al. 1988; de Vreede‐Swagemakers et al. 1997; Bayés de Luna et al. 1989; Spaulding et al. 1997). We do not discuss this topic in depth in this book (consult Fiol‐Sala et al. 2020 and Bayés de Luna and Baranchuk 2017). However, it is necessary to point out that acute ischemia frequently triggers ventricular arrhythmias which may lead to ventricular fibrillation and sudden death (Figure 20.30 and Chapter 16, Ventricular fibrillation). In fact, in nearly 50% of cases of sudden death, acute ischemia is the trigger of the fatal event (Figure 20.30), and ischemic heart disease is the most frequently associated disease. From an experimental point of view, ventricular arrhythmias have been shown to develop after ligature of a coronary artery in two phases. The first phase occurs after a few seconds, and is probably induced by a reentry mechanism; the second phase occurs after a few hours, and is most likely explained by post‐potential (Janse 1982). Severe ventricular arrhythmias, runs of ventricular tachycardia, and, occasionally, ventricular fibrillation appear in relation to most severe degrees of ischemia. This is especially true in case of long‐lasting ischemia and hearts with poor ventricular function (Bayés de Luna and Baranchuk 2017). Cases with the most severe ischemia, such as a coronary spasm with total occlusion of a large epicardial vessel (Prinzmetal angina or ACS with grade III of ischemia), is accompanied by significant ST segment elevation or even a TAP morphology (Figure 20.45). In the most extreme cases, T wave or ST segment alternans is observed (Figure 20.48). In general, severe ventricular arrhythmias do not appear immediately after the acute occlusion, but rather after a few minutes. Therefore, the danger of ventricular fibrillation is much more frequent in STE‐ACS evolving to MI than in coronary spasm. Acute coronary syndromes, especially STEMI, often trigger sudden death. Nowadays, with current treatments available, the number of cases with long‐term complications of MI, such as heart failure and low EF, is much lower than previously. However, acute and chronic ischemic heart disease is still often associated with sudden cardiac death, and this is probably the most important challenge in modern cardiology. In the United States, ischemic heart disease is present in 80–90% of cases of sudden death, but this association in the Mediterranean area is lower (Subirana et al. 2011). Globally (as is shown in Figure 20.30), in fewer than 50% of cases of sudden death are there clinical (presence of angina) (Marcus et al. 1988; de Vreede‐Swagemakers et al. 1997), electrocardiographic (ST changes in Holter recording) (Bayés de Luna et al. 1989), or coronarographic (acute thrombus) (Spaulding et al. 1997) findings that acute ischemia is the trigger of sudden death. Furthermore, in fewer than 50% of these cases is there pathologic evidence of acute infarction (fresh thrombus) (Burke et al. 1997; Subirana et al. 2011). These conclusions clearly clarify that around 50% of cases of sudden death are caused by acute ischemic attack. In the majority of other cases of sudden death in patients with IHD, the cause of death is sustained ventricular tachycardia/ventricular fibrillation (VT/VF) triggered by reentry around an old MI scar. It is interesting to point out that in the Mediterranean area, a higher incidence of cases of sudden death associated with left ventricular hypertrophy has been found than in the United States (Subirana et al. 2011). Figure 20.31 Patient with STE‐ACS due to proximal occlusion of dominant RCA (ST depression in I, ST elevation III > II, ST isodiphasic in V1 and ST elevation in V6). The patient presented with 2 × 1 atrioventricular (AV) block and suddenly advanced AV block. The most frequent supraventricular arrhythmia to occur during ACS is atrial fibrillation. Atrial fibrillation is a relatively frequent supraventricular arrhythmia (10–12% of cases of STE‐ACS and arrhythmia), as other supraventricular arrhythmias are typically related to atrial involvement (Liu et al. 1961; Zimerman et al. 1968) and/or pericarditis. Atrial fibrillation usually occurs in the most extensive ACSs. However, in patients with ACS due to RCA occlusion, it may be explained by vagal overdrive and may be accompanied by AV block. Age, presence of abnormal P wave (Agrawal et al. 2014), chronic obstructive pulmonary disease (COPD), and heart failure are triggering factors. The incidence of atrial fibrillation has decreased in the post‐thrombolytic era. Atrial fibrillation is usually self‐limited; thus, electric cardioversion is advised only when the heart rate is rapid and causes hemodynamic impairment. P‐wave analysis on signal‐averaged ECG may aid in predicting atrial fibrillation in ACS (Rosiak et al. 2002). Also all types of passive arrhythmias (sinus bradycardia, sinoatrial, and AV block–RCA occlusion) may be found. Figure 20.31 shows a third‐degree AV block that has a different significance according to the location of the MI: inferior or anteroapical. When it occurs in association with inferior MI, it usually evolves from a first‐degree block, the QRS complex is narrow, and the block is supra‐hisian. Thus, the prognosis is relatively good, though the mortality rate is higher than in cases with no advanced AV block with reperfusion therapy (Alnsasra et al. 2018). Fortunately, with the introduction of new treatments of acute phase, mechanical complications are much less frequent these days. Free‐wall rupture accompanied by cardiogenic shock may take place during the evolution of a Q wave acute MI. The persistence of ST segment elevation at the end of the first week without evolving to negative T wave as a sign of regional pericarditis is an indirect risk marker of cardiac rupture (Color Plate 5) (Reeder and Gersh 2000). This persistence of ST segment elevation, which is generally more persistent than significant, is seen more frequently in inferior and/or lateral wall MI than in anterior wall MI (Oliva et al. 1993). In general, the RCA and the LCX are more frequently involved than the LAD, and the lack of well‐developed collateral circulation favors the occurrence of transmural MI (Q wave or equivalent) with transmural homogeneous involvement of the entire wall. In patients with an evolving inferior acute MI, the presence of PR segment depression ≥1.2 mm in II, III, and aVF is associated with a higher risk of in‐hospital mortality and free‐wall cardiac rupture and/or atrial rupture when compared with patients without PR segment deviations (Jim et al. 2006). Figure 20.32 (A) A 55‐year‐old patient with previous myocardial infarction (MI) due to LCX occlusion who presents with a nearly normal ECG with low‐voltage R wave in V5–V6 with evident “slurrings.” (B) A 60‐year‐old patient with MI eight months previously. The coronary angiography demonstrates occlusion of the LCX. There are striking final “slurrings” in II, III, aVF, aVL, and the right precordial leads (V2–V3). The Q wave in the inferior leads and the “r” wave in V1 are narrow, and additionally the R/S ratio <0.5. This is a case of MI shown by striking final “slurrings” of QRS. (C) A 65‐year old woman in atrial fibrillation with two previous MIs due to RCA and LCX occlusion who presented with a typical fragmented QRS pattern. The ST elevation in the chronic phase is very suggestive of left ventricular inferoapical aneurysm. Septal rupture usually occurs, in turn, in larger infarctions, especially due to a proximal LAD occlusion. A systolic murmur may be heard, suggestive of a ventricular septal defect. Frequently, the patient presents with cardiogenic shock if surgery is not carried out urgently. The ECG may also show the persistence of ST segment elevation (Figure 22.11) with a higher incidence compared to Q wave infarction controls and the existence of RBBB with or without added superoanterior hemiblock (SAH), advanced AV block, and atrial fibrillation. Finally, papillary muscle infarction is more frequent than previously thought (40% of STEMI), although its prognosis is better than expected in the absence of ruptures (Tanimoto et al. 2010). The rupture that occurs currently very rarely causes acute mitral regurgitation frequently with acute pulmonary edema. Urgent surgery is often required. The posteromedial papillary muscle is more frequently involved, since its perfusion is derived only from the posterior descending artery (RCA or LCX), while the anterolateral papillary muscle has double perfusion (LAD and LCX). In contrast to free‐wall cardiac rupture, the infarct is frequently of the non‐Q wave type (>50% of cases). With regard to ventricular aneurysms, their presence has been classically suggested by the persistence of ST segment elevation. In chronic patients, the sensitivity of this sign is poor. Just 10% of the patients in the post‐infarction setting with ventricular aneurysm exhibit a higher than 0.1 mV ST segment elevation (East and Oran 1952). Also, it has been described that some changes of the mid‐late part of QRS, including rsR′ in left surface leads (Sherif 1970) and other morphologies (fractioned QRS), are very specific of ventricular aneurysm (Reddy et al. 2006) (Figure 20.32C). The deviations of the PR interval are especially seen in pericarditis (Figures 9.35) and atrial involvement in cases of MI (Figure 9.33). The diagnosis of atrial infarction is based especially on the presence of PR segment deviations (elevation or depression in different leads) associated with reciprocal changes in other leads (especially ST segment elevation in aVR and ST segment depression in II) and any atrial arrhythmias (Liu et al. 1961). Recently, it has been shown (Jim et al. 2006) (see above) that PR segment depressions ≥1.2 mm in patients with inferior STE‐ACS (ST segment elevation in II, III, and aVF) are a marker for high risk of AV block, supraventricular arrhythmias, cardiac rupture, and in‐hospital mortality. ACS with ST segment elevation and severe ischemia often present changes in the final portion of the QRS complex (distortion of QRS) (see Figure 20.2C). Severe ischemia may also induce QRS widening with or without classic patterns of intraventricular block. The importance of some of these changes from a prognostic point of view has already been discussed (see above). During the acute phase of ischemia, a lengthening of the QT interval (TAP) is the first manifestation of ischemia (Kenigsberg et al. 2007) (Figure 20.12) (see ECG changes during fibrinolysis and percutaneous coronary intervention). However, the change with respect to basal QT is difficult to identify. The presence of an evident long QTc interval at admission in a patient with ACS has been shown to be a marker of poor prognosis. In addition, patients with non‐Q wave infarction, in comparison with those with unstable angina, have longer QTc intervals overall (Rukshin et al. 2002). However, this is not useful when applied to an individual patient. Therefore, the troponin levels remain as the best way to distinguish whether an ACS with no ST segment elevation has evolved to a non‐Q wave infarction or has remained as an unstable angina. A normal U wave is always positive in the presence of a positive T wave and, under normal conditions, it is negative only in aVR. In patients with different clinical settings of IHD, U wave abnormalities may be recorded, generally as a negative U wave, while the T wave may be negative, positive, or flattened. The U wave may be positive when the T wave is negative (T–U discordance) (Reinig et al. 2005). When the negative T wave is recorded in precordial leads in patients with IHD, it is highly probable that the LAD is involved. A negative T wave may be present as acute ischemic events (STEMI or coronary spasm) (Figure 20.26) or during the post‐ischemic phase usually in NSTEMI (Figure 20.15 and Table 20.4). It should be noted that: (i) a negative U wave may be the only ECG sign of ischemia; (ii) it sometimes precedes ST segment changes; and (iii) it may increase the sensitivity of the exercise stress test (Correale et al. 2004). Repolarization abnormalities may be more visible in the PVCs than in normal ones and sometimes even are seen in PVC only (Figure 20.33). In addition, the ST segment depression in the PVCs in the exercise stress test has been shown to be more useful than the ST segment depression in normal complexes for predicting myocardial ischemia. The ST segment depression in the PVCs higher than 10% of the R wave amplitude in V4–V6 has a 95% sensitivity and a 67% specificity for predicting ischemia (Rasouli and Ellestad 2001). However, cases of repolarization abnormalities exist in the PVCs of healthy patients, and thus the test specificity is not very high. Figure 20.33 One example of exercise test in a patient with ischemic heart disease that demonstrates the presence of significant ST segment changes in premature ventricular complexes (see V3–V4) that were not so evident in normal sinus complexes. Figure 20.34 Recurrent acute coronary syndrome (ACS). A 75‐year‐old man with old anterior myocardial infarction complicated by right bundle branch block (RBBB), who presented with heart attack. Note the old Q wave from V1–V4, sinus tachycardia (pulmonary edema), and ST segment elevation in the inferior leads and ST segment depression in the right precordial leads due to occlusion of LCx. Furthermore, a meticulous study of the QRS complex or the T wave morphology of the PVCs may suggest an old infarction (qR morphology, with a wide q wave or slurred QS complex and sometimes a symmetric T wave). Recurrence refers to repeated episodes of ACS with or without ST segment elevation usually evolving into MI. It is not an infrequent event and usually appears after 2–3 years after the index MI, often in a different location and with the same type of ACS, suggesting that some patients present with factors that predispose to repeated episodes of STE or non‐STE‐ACS (Figure 20.34) (Fiol‐Sala et al. 2020). Before the era of reperfusion, it was relatively easy to predict the final Q wave infarction pattern according to the acute phase of STE‐ACS. With the current treatment strategies, if the treatment is started on time, the infarction may be faded (Figure 20.1). In patients with chronic Q wave MI, it is currently impossible to determine, from the correlation with the Q wave pattern, the exact degree and location of the occlusion of the culprit artery at the moment of an acute attack, because the area at risk is initially larger and become smaller as reperfusion treatment is successfully applied (Figure 20.9). Therefore, in the chronic phase of Q wave MI, what may be presumed is the location of the occlusion that produced the Q wave, but not the place of the occlusion when the ACS starts. In over 50% of cases of MI, the QRS complex does not show a Q wave of necrosis or equivalent (R in V1–V2). The MI without Q wave encompasses the classic non‐Q wave MI and many other types, as shown in Table 20.5, including the masked MI (wide QRS with LBBB and others) that sometimes may present with a Q wave. Furthermore, in 25–30% of cases, more in inferior MI, the Q wave disappears at follow‐up due to the presence of collaterals (Figure 20.35), the appearance of new necrosis (Color Plate 4), or new bundle branch block. Until relatively recently, it was thought that MIs in an exclusive subendocardial location existed and were electrically mute. However, the concept that MI with Q wave is transmural and non‐Q wave MI only involves subendocardial compromise is false. Now, it has been demonstrated from a pathologic point of view that infarctions usually do not have an exclusive subendocardial location and that infarctions with predominant subendocardial involvement may or may not develop a Q wave. Furthermore, some transmural MIs, such as those involving the basal areas of the left ventricle, may not exhibit a Q wave (Moon et al. 2004). Table 20.5 Myocardial infarction without Q wave or equivalent Contrast‐enhanced cardiovascular magnetic resonance (CE‐CMR) is the ideal technique for infarct identification, transmural characterization, and infarct localization and quantification. CE‐CMR has demonstrated that the Q wave MIs are larger than non‐Q wave ones, although they do not always have a deeper transmural extension. Therefore, the terms Q wave and non‐Q wave MIs are useful in the chronic phase from a prognostic point of view but not for determining whether a MI is or is not transmural (see Chapter 13, Q wave of necrosis). Since the early days of the ECG, the pattern of established transmural infarction has been associated with the presence of pathologic Q wave or its equivalents. Figure 13.32 shows the evolutionary appearance of the Q wave and negative T wave in cases of Q wave MI without modern treatment. Today, the evolution of the ECG may be very different if quick current treatment is established (see Figures 20.1 and 20.36). Figure 20.35 Patient with extensive anterior infarction. Note ECG normalization with Q wave disappearing and T wave becoming positive during 18‐month follow‐up. A to F. The changes associated with prior MIs are described in Table 13.1. Table 13.6 shows the ECG criteria of pathologic (necrosis) Q wave that in the appropriate clinical context may be used to diagnose prior MI (Thygesen et al. 2019). The presence of fractioned QRS (Das et al. 2006) must be considered abnormal and in the adequate clinical setting suggestive of prior MI. Remember that there are many pathologic Q waves not secondary to MI (see Table 13.9).
Chapter 20
The ECG in Different Clinical Set of Ischemic Heart Disease
Introduction
From exercise angina to acute coronary syndrome, and myocardial infarction
Abrupt decrease in blood flow: acute coronary syndrome–myocardial infarction (Table 20.2)
Acute coronary syndrome due to total or near total occlusive thrombosis and with complete transmural involvement: STEMI (Table 20.2)
Pathophysiology and clinical setting
Summary of ECG changes (see later)
Acute coronary syndrome usually with patent artery and non‐complete transmural involvement: NSTEMI (Table 20.2)
Pathophysiology and clinical setting
ECG
Clinical
Angiographic
Pathology
Treatment
ACS with narrow QRS STEMI (30% of total)
Rest pain or equivalents
After some time of subendocardial involvement there is a transmural homogeneous involvement
NSTEMI (50% of total) a (During pain)
With or without permanent rest pain
Summary of ECG changes
ECG patterns in acute coronary syndrome with ST segment elevation (STEMI) (Table 20.3, Figure 20.1) with narrow QRS
Typical ECG patterns (Chapter 13, ST segment in ACS, and Figures 20.1–20.4 and 13.38–13.52 )
Typical pattern
ST elevation with mirror pattern (≈ 30% of all ACS)a
Equivalent pattern
Mirror pattern in V1–V3–V4 with ST depression (LCX occlusion) (≈5%). Often, some ST elevation in inferior/lateral leads (Figure 20.4A)
Patterns that may be seen during the acute process
At early phase: Tall and usually very transient T wave (Figure 20.4 B1) (<2%). Sometimes the tall T wave is persistent (hours) and may present ST depression (Figures 20.4B2 and B3) (<2%)
After resolution of ST elevation without evolution to Q wave MI: deep negative T wave, especially in LAD occlusion (V1–V2 to V4–V5) (≈5%) (Figure 20.4C)
Atypical ECG patterns (Figure 20.4, Table 20.3)
Differential diagnosis
Clinical and prognostic implications
Location of occlusion
Global prognostic value of ECG changes at admission (Fiol‐Sala et al. 2020)
ECG changes during fibrinolysis and percutaneous coronary intervention (Figure 20.11)
Fibrinolysis
Percutaneous coronary intervention (PCI)
ECG in acute coronary syndrome with no ST elevation (NSTEMI) (Table 20.4)
ECG patterns (see Chapter 13, ST segment in ACS and Figures 20.16–20.26)
ST depression ≈ 70%
Extensive involvement: ST↓ in ≥7 leads with ST↑ in 1–2 leads (aVR > V1):
Lead aVR
Mid‐precordial leads
A.ST↑ > 1 mm
A. LM (Figure 20.18)
LM/3VD (Figure 20.19)
3VD (Figure 20.20)
B.ST↑ ≤ 1 mm
B. Usually 3VD/may be non‐severe LM Also ST↓ in I and aVL (Figure 13.30)
C.ST↑ < 1 mm
C. Figure 20.21. Severe LAD proximal subocclusion
D. ST↑ < 1 mm
D. LCX proximal subocclusion. Also, ST↓ in II and aVF
Regional involvement: ST↓ in <7 leads
Flat/negative T wave (≈15% of cases)
May be seen in different locations: V1–V3 proximal LAD (Figure 13.12); V4–V6 (distal LAD/LCX), etc.
Changes of U wave (<1%)
May be seen as a post‐ischemic pattern in case of LAD involvement (Figure 20.15), and also in case of acute ischemia (negative U with pseudonormalization of T wave) (Figure 20.26)
ECG normal or unmodified (≈15%)
The ECG may be normal even during pain. Usually, ACS with normal ECG during pain has good prognosis. Many cases with 3VD or LM trunk without pain present with normal ECG (Figure 20.17)
Differential diagnosis
The prognostic value of ECG changes at admission (Fiol‐Sala et al. 2020)
General considerations
High‐risk group
Low‐risk group
Changes from NSTEMI to STEMI ECG patterns and vice versa
Acute coronary syndrome with confounding factors: left ventricular hypertrophy and wide QRS
Bundle branch block and other situations with wide QRS
New or “presumably new” LBBB
Mistakes performed in the ECG interpretation of ACS (Birnbaum et al. 2012; Fiol‐Sala et al. 2020)
Arrhythmias in acute coronary syndromes
Ischemia and sudden death
Mechanical complications of an ACS evolving to myocardial infarction (Color Plate 5) (Fiol‐Sala et al. 2020)
Other ECG changes in acute coronary syndrome
P wave and PR segment
Changes in the QRS complex
QT interval
U wave changes (Table 20.4)
Changes in PVC morphology
Recurrence of acute coronary syndrome
ECG in chronic myocardial infarction with narrow QRS
Evolutionary concepts
Left bundle branch block
Wolff–Parkinson–White, Pacemaker} Often can present with an abnormal Q wave
Q wave myocardial infarction with narrow QRS (see Chapter 13)
The evolution of the ECG pattern
Diagnostic criteria of Q wave myocardial infarctions
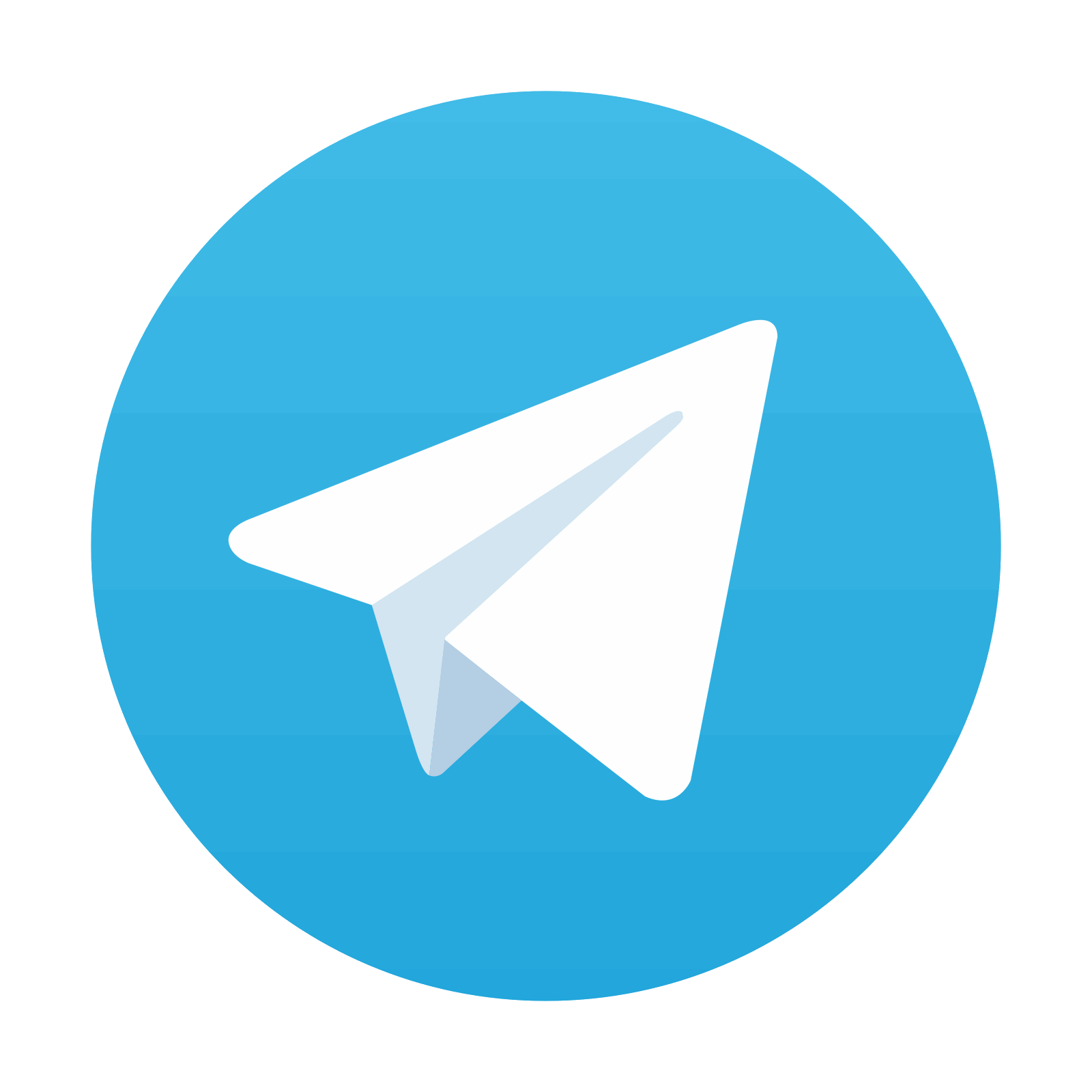
Stay updated, free articles. Join our Telegram channel

Full access? Get Clinical Tree
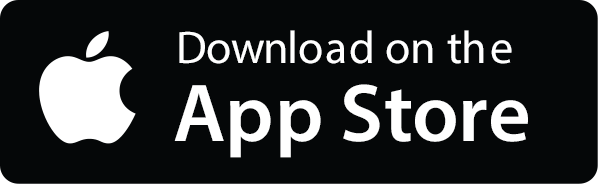
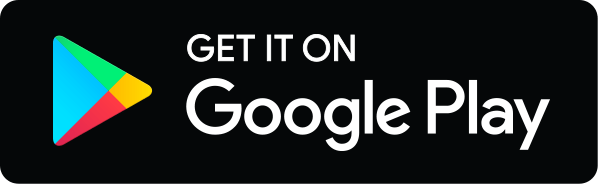