The Cytoskeleton
Come to me now, you muses who live on Olympus for you are goddesses, are everywhere and know all things, while we only hear of glory but do not truly know it. Who were the leaders of the Greeks, who the rulers? I could not tell the names or number of all the men, even if I had ten tongues, ten mouths, an unwearied voice, and a heart of bronze, unless you, Olympian muses, daughters of Zeus who bear the aegis, recall for me the men who came to Troy, so now I will recount the leaders of the ships, and all their numbers.
—Homer (Iliad. Book 2, 484–493. tr. P.B. Katz)
L ike Book 2 of Homer’s Iliad, this chapter includes a list of the many cytoskeletal proteins found in cardiac myocytes. As is true for any list, the role and significance of each entry can be difficult to appreciate. However, discoveries made over the past decade have made it clear that cytoskeletal proteins are important in the pathogenesis of human disease; they generate and transmit maladaptive signals that contribute to the progressive deterioration of failing hearts, and mutations in many of these proteins have been identified as causes of cardiomyopathies and sudden cardiac death. The following pages are intended to provide a brief introduction to many of these proteins, along with a framework by which these important molecules can be organized. Because the overall goals of this introductory text require that the descriptions of each protein be brief, the reader who seeks more detail is referred to the authoritative reviews cited in the bibliography.
Cardiac myocytes are not simply fluid-filled bags in which enzymes and cell organelles like myofilaments, the sarcoplasmic reticulum, nuclei, and mitochondria float freely in the cytosol; instead, a complex cellular architecture is maintained by a protein framework called the cytoskeleton. Like the girders in a modern building, these structural proteins hold cell constituents in place, organize functionally related proteins, and facilitate mechanical interactions with adjacent cells and the extracellular matrix. In addition to these structural functions, the cytoskeleton participates in cell signaling, and makes a major contribution to the signal transduction pathways that modify the size and shape of the heart. The cytoskeleton, therefore, is not simply a system of girders that maintains cell shape; because of its role in cell signaling, the cytoskeleton is more accurately viewed as a structural framework that also serves as a phone system!
In cardiac myocytes, the cytoskeleton organizes spatial relationships among intracellular structures, transmits tension developed by the contractile proteins from one sarcomere to another and to the cell surface, and links cells to other cells and to the extracellular matrix. The cytoskeleton also helps the heart adapt its architecture in response to cell deformation and changes in mechanical stress. By modifying the size, shape, and composition of the heart, cytoskeletal signaling serves a key role in adapting form to function. This is seen in the activation of specific signaling pathways by various types of cytoskeletal deformation, which contributes to the appearance of different
phenotypes of cardiac hypertrophy and helps explain why pressure overload causes concentric hypertrophy (seen in aortic stenosis where systolic stress is increased), and volume overload causes eccentric hypertrophy (as occurs in aortic insufficiency where diastolic stress is increased). These proliferative responses are especially important in failing hearts, where cytoskeletal signaling contributes to the different types of overload-induced hypertrophy. Although initially beneficial, these proliferative responses generally become maladaptive when the overload is sustained. Cytoskeletal signaling plays a role in determining prognosis in systolic heart failure, where deleterious transcriptional signaling contributes to the progressive ventricular dilatation, called remodeling, that shortens survival (see Chapter 18). For these reasons, cytoskeletal proteins can serve as targets for therapy directed to modifying maladaptive growth responses in diseased hearts. Drugs that can modify the behavior of these proteins are also likely to help patients with familial cardiomyopathies, many of which are caused by mutations in cytoskeletal proteins.
phenotypes of cardiac hypertrophy and helps explain why pressure overload causes concentric hypertrophy (seen in aortic stenosis where systolic stress is increased), and volume overload causes eccentric hypertrophy (as occurs in aortic insufficiency where diastolic stress is increased). These proliferative responses are especially important in failing hearts, where cytoskeletal signaling contributes to the different types of overload-induced hypertrophy. Although initially beneficial, these proliferative responses generally become maladaptive when the overload is sustained. Cytoskeletal signaling plays a role in determining prognosis in systolic heart failure, where deleterious transcriptional signaling contributes to the progressive ventricular dilatation, called remodeling, that shortens survival (see Chapter 18). For these reasons, cytoskeletal proteins can serve as targets for therapy directed to modifying maladaptive growth responses in diseased hearts. Drugs that can modify the behavior of these proteins are also likely to help patients with familial cardiomyopathies, many of which are caused by mutations in cytoskeletal proteins.
The mechanical and signaling functions of the cytoskeleton are made possible by interactions among dozens of proteins. Some of these proteins are largely structural, while other proteins associated with the cytoskeleton, including protein kinases, phosphatases, and transcription factors, function mainly in signal transduction. However, many cytoskeletal proteins serve both mechanical and signaling functions, so that the designations “structural” and “signaling” in the following discussion are arbitrary. In many ways, these proteins are like the front wheels of an automobile, which both hold the vehicle to the road and participate in steering.
Cytoskeletal Filaments
The cytoskeleton contains three types of intracellular fiber (Table 5-1). Actin microfilaments are found in two structures: the thin filaments of the sarcomeres (see Chapter 4) and microfilaments. The latter connect the contractile proteins, plasma membrane, and other cytoskeletal elements to one another, connect the cytoskeleton to the extracellular matrix, and anchor cells to each
other. Microtubules, which are made up mainly of tubulin, transport organelles and vesicular structures within cardiac myocytes and participate in the mitosis occasionally seen in these terminally differentiated cells (see Chapter 9). Specialized arrays of microtubules participate in the movements of cilia and flagella, but these are not found in adult cardiac myocytes. The heart contains two types of intermediate filament. Class III intermediate filaments contain desmin, and form strong rivet-like structures, called desmosomes, which help maintain cell architecture, link cells to one another, and attach cells to the extracellular matrix. Class V intermediate filaments, which contain lamin, support the nuclear membrane. Unlike microfilaments and microtubules, intermediate filaments do not participate in cell motion or intracellular transport. However, all of these cytoskeletal fibers participate in cell signaling.
other. Microtubules, which are made up mainly of tubulin, transport organelles and vesicular structures within cardiac myocytes and participate in the mitosis occasionally seen in these terminally differentiated cells (see Chapter 9). Specialized arrays of microtubules participate in the movements of cilia and flagella, but these are not found in adult cardiac myocytes. The heart contains two types of intermediate filament. Class III intermediate filaments contain desmin, and form strong rivet-like structures, called desmosomes, which help maintain cell architecture, link cells to one another, and attach cells to the extracellular matrix. Class V intermediate filaments, which contain lamin, support the nuclear membrane. Unlike microfilaments and microtubules, intermediate filaments do not participate in cell motion or intracellular transport. However, all of these cytoskeletal fibers participate in cell signaling.
Table 5-1 Cytoskeletal Filaments | |||||||||||||||||||||||||
---|---|---|---|---|---|---|---|---|---|---|---|---|---|---|---|---|---|---|---|---|---|---|---|---|---|
|
Cytoskeletal Proteins Associated with the Myofilaments
Our view of the sarcomere has changed dramatically since the 1950s, when the sliding filament hypothesis for muscular contraction was first proposed. At that time, sarcomeres were thought to be made up of two proteins, actin and myosin; in the 1970s, after the roles of tropomyosin and the troponin complex were recognized, this number increased to six (see Chapter 4). However, the classical studies of Huxley and Hanson (1960) showed that although the A-bands disappeared when myosin was extracted from muscle fibers, and that subsequent extraction of actin removed the I-bands, removal of these proteins did not cause the fibers to fall apart. Instead, the sarcomeres remained intact and Z-lines retained their normal alignment. For almost 30 years, these observations, while not forgotten, were generally overlooked. It was not until the 1990s that the role of the cytoskeletal proteins, which maintained sarcomere structure after the contractile proteins had been removed, came to be appreciated. Initially, the cytoskeleton was believed simply to provide mechanical support that helped maintain sarcomere structure and transmit the tension developed by the contractile proteins to adjacent sarcomeres, other myocytes, and the extracellular matrix. It is now clear, however, that cytoskeletal proteins also regulate the lengths of the thick and thin filaments and serve a number of vital signaling functions. Even more important clinically has been the recognition that disturbances of these signaling functions are important in human disease.
Cytoskeletal Proteins of the Thick Filaments
The thick filaments, once thought to be composed entirely of myosin, are now known to contain a number of cytoskeletal proteins. Some are incorporated into these filaments where they bind directly to myosin. An even larger number of cytoskeletal proteins form aggregates that link the thick filaments to nearby structures and participate in cell signaling. The resulting complexity makes it difficult to create visual images that describe the interactions among these proteins; for this reason, the following descriptions of these interactions use both diagrams and lists.
Titin, a huge protein with a molecular weight of ∼3,000,000 that extends from the Z-lines to the center of the thick filament (Fig. 5-1), supports sarcomeric structure and contributes to the high resting stiffness of the myocardium (see Chapter 6). The regions of titin that are incorporated into the thick filaments add rigidity to these structures, while a more compliant region in the I-bands contributes to the heart’s elasticity. Phosphorylation of titin by PKA reduces sarcomere stiffness, and so facilitates ventricular filling when β-adrenergic activity is increased, as during exercise.
Two titin isoforms, N2B and N2BA that differ in the flexible region of the molecule, are found in adult human ventricles. N2BA, which is normally the dominant isoform, is more compliant than N2B. Heart failure patients with dilated left ventricles (“systolic heart failure”) overexpress the more compliant N2BA isoform, whereas those whose left ventricles are stiff and have smaller cavities (“diastolic heart failure”) have an increased content of the stiffer N2B isoform. Mutations in titin are associated with both dilated and hypertrophic cardiomyopathies (see Chapter 18).
Titin forms transverse fibers with a number of other cytoskeletal proteins in the M-bands at the center of thick filaments (Fig. 5-1). These include myosin binding protein C (C protein), M-protein, and myomesin, which provide lateral mechanical stability to the sarcomere, and muscle creatine kinase, adenylate kinase, and phosphofructokinase, which may participate in length-dependent modifications of energy production. Obscurin, another titin-linked M-band protein, connects the sarcomeres to the sarcoplasmic reticulum through small ankyrin-1 (see below). Ubiquitin and the ubiquitin-like modifier SUMO3 regulate protein degradation. Additional M-band proteins participate in cell signaling; these include calmodulin, myofibrillogenesis regulator 1, four-and-a-half LIM protein (FHL), the transcription factors p53, muscle-specific ring finger protein (MURF-1), and the two zinc-finger proteins (nbr1 and p62) (see Chapter 9). The M-band proteins provide an example of a “hot spot” where interactions between structural and regulatory proteins generate proliferative responses.
The interactions among the cytoskeletal proteins shown in Figure 5-1 suggest several ways that M-band proteins could mediate specific responses to cell deformation. For example, MURF, FHL, and the two zinc-finger proteins (nbr1 and p62) regulate gene transcription and protein synthesis, which could allow these cytoskeletal proteins to modify the heart’s size, shape, and composition in response to abnormal mechanical stresses. Interactions of the M-band proteins with obscurin, small ankyrin-1, and the sarcoplasmic reticulum might contribute to length-dependent changes in calcium release by these internal membranes (see Chapter 6).
Different responses of cytoskeletal proteins oriented parallel and perpendicular to the long axis of the sarcomere probably explain why transverse and longitudinal stretch of cardiac myocytes evokes different focal adhesion kinase responses (Senyo et al., 2007), and activation of different mitogen-activated protein kinases when cardiac myocytes are stretched in systole and in diastole (Yamamoto et al., 2001). Activation of different cytoskeletal signal transduction pathways by increased systolic and diastolic stress can also explain how chronic pressure overload causes concentric hypertrophy, as occurs when aortic stenosis increases systolic stress, and how volume overload causes eccentric hypertrophy, as occurs when aortic insufficiency increases stress during diastole (Katz and Konstam, 2009).
Cytoskeletal Proteins of the Thin Filaments
Actin can be viewed both as part of the cytoskeleton and as a contractile protein. As the former, sometimes called cortical actin, actin is found in the microfilaments (see above), while as sarcomeric actin this protein makes up the backbone of the thin filaments (see Chapter 4). Two cytoskeletal proteins cap the ends of the thin filaments; tropomodulin, which is found at the ends that interdigitate with the A-bands, and cap Z (β-actinin), which anchors the other ends of the thin filaments in the Z-lines (Fig. 5-2). In addition to linking the thin filaments to other proteins, tropomodulin and cap-Z regulate thin filament length and participate in proliferative signaling. Nebulette, a cytoskeletal protein that runs alongside the thin filaments from the Z-line into the I-bands, interacts with a variety of signaling proteins, most of which are found at the Z-lines.
Cytoskeletal Proteins of the Z-Lines
The Z-lines (or Z-discs) (see Chapter 1) are rigid structures in which the thin filaments of adjacent sarcomeres are interwoven with a number of cytoskeletal proteins that transmit tension developed by the contractile proteins to the fascia adherens of the intercalated discs that link
adjacent cells (Fig. 5-3). The Z-lines also anchor a number of proteins that are linked to cytoskeletal filaments that connect to the plasma membrane, sarcoplasmic reticulum, and other cellular structures (see below). Rigid bands made up of desmin and α-actinin surround the Z-lines in structures called costameres that resemble the staves of a barrel. Desmin, the major protein of the costameres, is one of a family of intermediate filament proteins that includes vimentin, which supports connective tissue cells, keratins found in epithelial cells, neurofilaments that help organize axon structure, glial fibrillary acidic protein in astrocytes and other glial cells, and lamins that support the inner surface of nuclear membranes. Desmin links the Z-lines to intermediate filaments that connect to desmosomes in the intercalated disc (see Chapter 1), and so participates in transmitting tension between adjacent cardiac myocytes. As desmin lacks signaling domains, this protein probably does not directly modify cell signaling; however, by transmitting tension to other cytoskeletal proteins, desmin can play an indirect role in signal transduction.
adjacent cells (Fig. 5-3). The Z-lines also anchor a number of proteins that are linked to cytoskeletal filaments that connect to the plasma membrane, sarcoplasmic reticulum, and other cellular structures (see below). Rigid bands made up of desmin and α-actinin surround the Z-lines in structures called costameres that resemble the staves of a barrel. Desmin, the major protein of the costameres, is one of a family of intermediate filament proteins that includes vimentin, which supports connective tissue cells, keratins found in epithelial cells, neurofilaments that help organize axon structure, glial fibrillary acidic protein in astrocytes and other glial cells, and lamins that support the inner surface of nuclear membranes. Desmin links the Z-lines to intermediate filaments that connect to desmosomes in the intercalated disc (see Chapter 1), and so participates in transmitting tension between adjacent cardiac myocytes. As desmin lacks signaling domains, this protein probably does not directly modify cell signaling; however, by transmitting tension to other cytoskeletal proteins, desmin can play an indirect role in signal transduction.
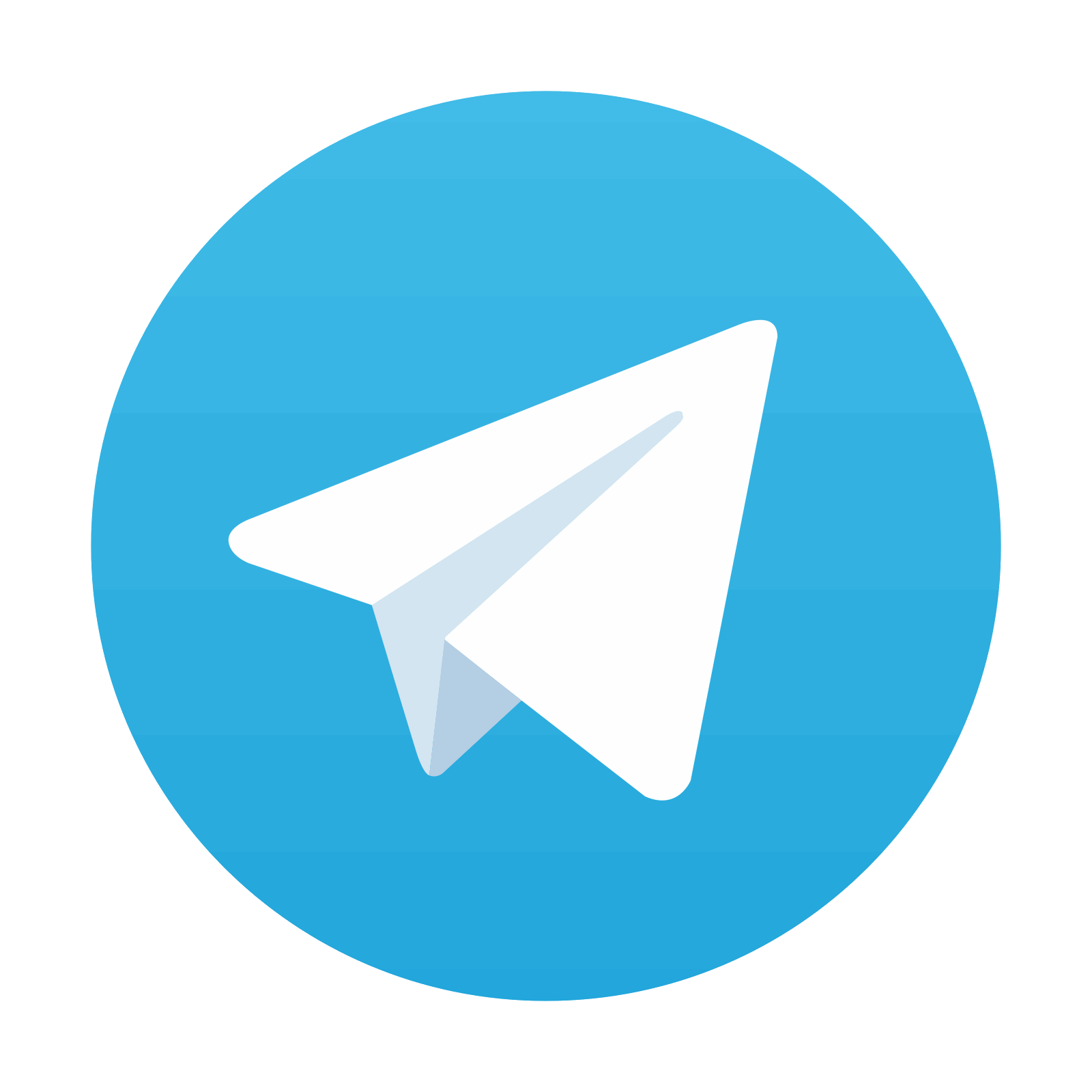
Stay updated, free articles. Join our Telegram channel

Full access? Get Clinical Tree
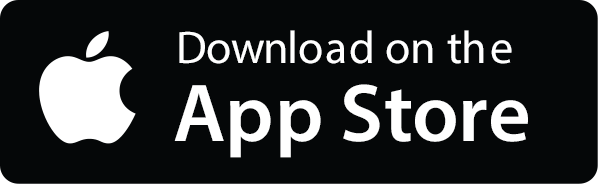
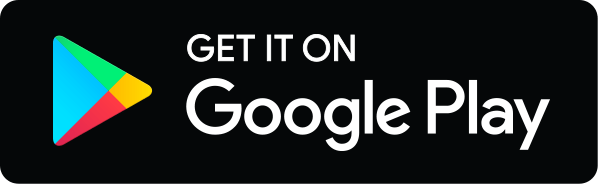