Structure of the Heart and Cardiac Muscle
It has been shown by reason and experiment that blood by the beat of the ventricles flows through the lungs and heart and is pumped to the whole body … the blood in the animal body moves around in a circle continuously, and … the action or function of the heart is to accomplish this by pumping. This is the only reason for the motion and beat of the heart.
—William Harvey (1628).
Exercitatio Anatomica de Moto Cordis et Sanguinis in Animalibus.
Harvey’s proof that the heart is a muscular pump, which overthrew the ancient view that the heart is the source of the body’s heat, along with increasing use of human autopsies, made it possible to understand the pathogenesis of heart disease in terms of abnormal organ structure (Katz, 2008). Virchow’s founding of pathology in the 19th century supplemented this anatomical knowledge with histological pathology. In the 20th century, development of electron microscopy and new insights into the biochemistry and biophysics of cardiac function further extended knowledge of the causes of heart disease. Today, rapid advances in molecular biology are providing additional insights into the ultrastructural and molecular basis of cardiovascular disease.
Organ Structure
Mammalian hearts can be viewed as two pumps that operate in series: the right atrium and the right ventricle, which pump blood from the systemic veins into the pulmonary circulation, and the left atrium and the left ventricle, which pump blood from the systemic veins into the pulmonary circulation (Fig. 1-1). Within the heart, atrioventricular (AV) valves prevent blood from flowing backward from the ventricles into the atria: on the right the tricuspid valve and on the left the mitral valve (Fig. 1-2). Semilunar valves, named for their crescent-shaped cusps, separate each ventricle from its great artery: the pulmonic valve between the right ventricle and the pulmonary artery, the aortic valve between the left ventricle and the aorta. All four of these valves lie in a plane within a connective tissue “skeleton” that separates the atria and ventricles in which the mitral, tricuspid, and aortic valves surround a fibrous triangle, called the central fibrous body (Fig. 1-3). This connective tissue skeleton can be viewed as an insulator that prevents electrical impulses from being conducted between the atria and the ventricles. The AV bundle (also called the common bundle or bundle of His), a strand of specialized cardiac muscle that penetrates this insulator, normally provides the only conducting pathway between the atria and the ventricles. Damage to this critical conducting structure is an important cause of AV block (Chapter 16).
![]() Fig. 1-1: Circulation of the blood. Light shading: deoxygenated blood; dark shading: oxygenated blood. RA, right atrium; LA, left atrium; RV, right ventricle; LV, left ventricle. (Adapted and modified from Starling, 1926). |
The free margins of the semilunar aortic and pulmonary valve cusps are supported by thick tendinous edges. Sinus of Valsalva lie behind each of the three aortic valve cusps; the anterior and left posterior sinuses contain the orifices of coronary arteries (see below), whereas the right posterior sinus does not give rise to a coronary artery and so is often called the “noncoronary” sinus (see Fig. 1-3). The larger cusps of the mitral and tricuspid valves are tethered at their free margins by fibrous chordae tendinae that attach to “fingers” of myocardium called papillary muscles that project into the right and left ventricular cavities (see Fig. 1-2). Much as the strands of a parachute arise from a skydiver’s harness, several chordae tendinae fan out from each papillary muscle to support the valve margins (Becker and deWit, 1979). Laxity of the connective tissue supporting the mitral valve can allow the leaflets to move backward (prolapse) into the left atrium when left ventricular pressure rises during systole. In some patients, abrupt opening of these abnormal valves causes an audible “click”; if blood subsequently leaks from the left ventricle into the left atrium (mitral regurgitation), a late systolic murmur can be heard. This syndrome, called mitral valve prolapse, is often of no hemodynamic significance (although leaky valves are susceptible to bacterial infection); when severe, however, laxity of the chordae tendinae can cause significant mitral regurgitation. Rupture of a papillary muscle, which can occur when coronary artery occlusion interrupts the blood supply to these vital muscular structures (see Chapter 17), generally causes severe mitral regurgitation and can be fatal.
![]() Fig. 1-2: Major structures in a human heart opened after transection slightly anterior to the midline. (Adapted and modified from Berne and Levy, 1967.) |
Architecture of the Walls of the Heart
When the heart is viewed from above, the rounded margin of the left ventricle forms an above obtuse angle, whereas the margin of the right ventricle is sharper, like an acute angle (see Fig. 1-3); this explains the terms “obtuse marginal” and “acute marginal” used in naming branches of the coronary arteries (see below). The thin-walled atria, which develop much lower pressures than do the ventricles, contain ridges of myocardium called pectinate muscles that may provide preferential conducting pathways, often referred to as internodal tracts or sinoatrial (SA) ring
bundles, that link the SA and AV nodes (Hayashi et al., 1982). The ventricles, which develop much higher pressures than do the atria, have thicker muscular walls. The left ventricle, which has approximately three times the mass and twice the thickness of the right ventricle, can be viewed as a “pressure pump” whose cavity resembles an elongated cone in which the mitral valve, through which blood flows into the ventricle, and the aortic valve, through which blood leaves the ventricle, lie side-to-side in the wider end (Fig. 1-4). Peak systolic pressure in the left ventricle is normally about three times higher than that in the right ventricle; the latter, which represents a “volume pump,” is shaped like a crescent with inflow through the tricuspid valve at one end and outflow through the pulmonic valve at the other (see Fig. 1-4). During systole, the interventricular septum normally moves toward the left ventricular free wall and so participates in left ventricular ejection. In chronic right ventricular overload, for example, in patients with pulmonary hypertension, the septum can move paradoxically away from the left ventricular cavity during systole to aid right ventricular ejection.
bundles, that link the SA and AV nodes (Hayashi et al., 1982). The ventricles, which develop much higher pressures than do the atria, have thicker muscular walls. The left ventricle, which has approximately three times the mass and twice the thickness of the right ventricle, can be viewed as a “pressure pump” whose cavity resembles an elongated cone in which the mitral valve, through which blood flows into the ventricle, and the aortic valve, through which blood leaves the ventricle, lie side-to-side in the wider end (Fig. 1-4). Peak systolic pressure in the left ventricle is normally about three times higher than that in the right ventricle; the latter, which represents a “volume pump,” is shaped like a crescent with inflow through the tricuspid valve at one end and outflow through the pulmonic valve at the other (see Fig. 1-4). During systole, the interventricular septum normally moves toward the left ventricular free wall and so participates in left ventricular ejection. In chronic right ventricular overload, for example, in patients with pulmonary hypertension, the septum can move paradoxically away from the left ventricular cavity during systole to aid right ventricular ejection.
The left ventricular cavity, which is conical in shape during diastole, assumes a more spherical shape as intraventricular pressure rises at the end of isovolumic contraction (Hawthorne, 1961, 1969) (Fig. 1-5). During left ventricular ejection, its cavity again assumes its conical shape. Because ejection propels blood superiorly (toward the head), according to Newton’s third Law—which states that for every action there is an equal and opposite reaction—the base of the heart moves inferiorly (toward the feet). This movement, called “decent of the base,” explains the prominent “x descent” seen in the normal venous pulse during ventricular ejection.
The heart, along with a small amount of fluid, is contained within a noncompliant fibrous sac called the pericardium whose inner surface, the parietal pericardium, is continuous with the epicardium (see below). The cavities of the atria and the ventricles, along with the valves, are lined with another connective tissue layer called the endocardium (Brutsaert, 1989). Because the heart is contained within the rigid pericardium (see below), the ventricles interact with one another. Ventricular interactions are especially important in diastole, when dilatation of one ventricle can impair filling of the other (Yacoub, 1995; Santamore and Dell’Italia, 1998; Williams and Frenneaux, 2006).
The muscular walls of the ventricles are made up of overlapping fiber bundles, sometimes called bulbospiral and sinuspiral muscles, that follow spiral paths as they sweep from the fibrous skeleton at the base of the heart to its apex (Grant, 1965; Lower, 1669; Streeter et al., 1969;
Fenton et al., 1978; Sengupta et al., 2006). The bundles at the epicardial surface of the left ventricle are oriented as a right-handed helix that tends to parallel the base-apex axis of the heart, whereas those at the endocardial surface form a left-handed helix and are oriented more circumferentially (Cheng et al., 2008) (Fig. 1-6).
Fenton et al., 1978; Sengupta et al., 2006). The bundles at the epicardial surface of the left ventricle are oriented as a right-handed helix that tends to parallel the base-apex axis of the heart, whereas those at the endocardial surface form a left-handed helix and are oriented more circumferentially (Cheng et al., 2008) (Fig. 1-6).
![]() Fig. 1-5: Schematic diagrams of the canine left ventricle at the ends of diastole, isovolumic contraction, and ejection. The broad lightly shaded arrow in the right-hand side of the diagram shows the “decent of the base” in an inferior direction as blood is ejected in a superior direction in to the aorta (darkly shaded arrow). Ao, aorta; LA, left atrium; LV, left ventricle. (Adapted and modified from Hawthorne, 1961.) |
![]() Fig. 1-6: Spiral musculature of the ventricular walls. A: Spiral bundles in the left ventricle. Left: anterior view, Right: inferior view. (Modified from Lower, 1669.). B: Schematic drawing of the spiral bundles that sweep from the fibrous skeleton at the base of the heart (above) to the apex (below). C: Schematic diagram showing the different helical orientations of the fiber bundles in the subendocardium (left) and the subepicardium (right). |
Electrical Activation
The heartbeat is initiated and controlled by electrical impulses that are generated and conducted by specialized myocardial cells in different regions of the heart. Activation normally begins in the SA node (Fig. 1-7), a band of spontaneously depolarizing cells derived from the embryonic right sinus venosus that lies between the superior vena cava and the right atrium (Oosthoek et al., 1993a; Anderson and Ho, 1998; Verheijck et al., 1998). Because the firing rate of the SA node is more rapid than that of the other regions of the heart, this structure normally serves as the cardiac pacemaker (see Chapter 15).
The wave of depolarization initiated by the SA node is propagated through atrial myocardial cells to the right atrium, and then to the left atrium. After encountering a delay in the slowly
conducting cells of the AV node, which is derived from the embryonic left sinus venosus, the wave of depolarization enters the AV bundle (Oosthoek et al., 1993b). The latter, a rapidly conducting structure made up of Purkinje cells (see below), bifurcates into right and left bundle branches at the top of the interventricular septum. The right bundle branch crosses the right ventricular cavity within the moderator band, a muscular bundle that extends from the interventricular septum to the base of the papillary muscle that supports the anterior leaflet of the tricuspid valve (Fig. 1-7). The left bundle branch is often pictured as bifurcating into anterior and posterior fascicles, but this branching is highly variable (see Chapter 15). The impulses conducted through the bundle branches reach the ventricular myocardium via the His-Purkinje system, a subendocardial network of rapidly conducting cells that synchronizes ventricular activation.
conducting cells of the AV node, which is derived from the embryonic left sinus venosus, the wave of depolarization enters the AV bundle (Oosthoek et al., 1993b). The latter, a rapidly conducting structure made up of Purkinje cells (see below), bifurcates into right and left bundle branches at the top of the interventricular septum. The right bundle branch crosses the right ventricular cavity within the moderator band, a muscular bundle that extends from the interventricular septum to the base of the papillary muscle that supports the anterior leaflet of the tricuspid valve (Fig. 1-7). The left bundle branch is often pictured as bifurcating into anterior and posterior fascicles, but this branching is highly variable (see Chapter 15). The impulses conducted through the bundle branches reach the ventricular myocardium via the His-Purkinje system, a subendocardial network of rapidly conducting cells that synchronizes ventricular activation.
![]() Fig. 1-7: Conducting system of the human heart (capitalized labels at right) and major anatomical features (lower case labels at left). AV, atrioventricular; SA, sinoatrial. (Modified from Benninghoff, 1944.) |
The Coronary Circulation
Major Epicardial Coronary Arteries
Large epicardial coronary arteries carry virtually all of the blood that supplies the heart. Although a few layers of endocardial myocytes are perfused from the ventricular cavities via arteriosinusoidal and arterioluminal vessels, this auxiliary blood supply is of no clinical importance when a large coronary artery becomes occluded (Chapter 17). The major coronary arteries are the left main coronary artery (LEFT MAIN), right coronary artery (RCA), left anterior descending (LAD), circumflex (CIRC), and posterior descending artery (PDA) (Fig. 1-8). All lie
in grooves between the heart’s chambers: the RCA and the CIRC between the atria and the ventricles, the LAD and the PDA between the left and right ventricles.
in grooves between the heart’s chambers: the RCA and the CIRC between the atria and the ventricles, the LAD and the PDA between the left and right ventricles.
![]() Fig. 1-8: Major coronary arteries and their branches (labels at right and left) and key elements of the cardiac conduction system (labels above and below). AV, atrioventricular; SA, sinoatrial. |
The anatomy of these vessels can be summarized by the statement three out of two makes four. Two coronary arteries arise from the aorta (RCA and LEFT MAIN) and, after the LEFT MAIN divides into the LAD and CIRC, continue as three vessels (RCA, LAD, and CIRC). The PDA is a continuation of either the RCA or the CIRC, so that the myocardium is supplied by four major arteries (RCA, LAD, CIRC, and PDA).
The LEFT MAIN, which originates in the left posterior sinus of Valsalva (see Fig. 1-3), continues as a single vessel of variable length before dividing into its two major branches: the LAD and the CIRC (see Fig. 1-8). The LAD, which courses down the anterior interventricular groove, gives rise to septal perforating arteries that supply the anterior two-thirds of the interventricular septum, diagonal branches that supply the anterior wall of the left ventricle, and right ventricular branches that provide blood to the anterior wall of the right ventricle. After crossing the apex of the heart, the LAD usually turns upward, toward the base, to run a short distance in the posterior interventricular groove (see Fig. 1-8; Fig. 1-9). The CIRC, which courses to the left in the anterior AV groove, gives rise to obtuse marginal branches that supply the lateral wall of the left ventricle. In most human hearts, the CIRC, after reaching the back of the left ventricle, runs only a short distance down the posterior interventricular groove to end near the crux of the heart, where the plane of the interventricular septum crosses the plane of the AV groove (Fig. 1-9B).
The PDA, which can arise from either the CIRC or the RCA, runs inferiorly in the posterior interventricular groove where it supplies septal perforating branches that perfuse the posterior third of the interventricular septum. In approximately 90% of human hearts the PDA is supplied by the RCA (called “right dominant”); in the remaining approximately 10% the CIRC turns downward at the crux to supply the PDA (“left dominant”) (Fig. 1-9A).
The RCA, which arises from the anterior sinus of Valsalva, courses toward the right in the anterior AV groove where it gives rise to right ventricular (acute marginal) branches that supply the free wall of the right ventricle. The RCA then crosses the acute margin of the heart, turns to the left in the posterior AV groove, and, after reaching the crux of the heart, usually continues in the posterior interventricular groove as the PDA (“right dominant” coronary circulation, Fig. 1-9B).
Coronary occlusive disease is often described as “one-vessel,” “two-vessel,” and “three-vessel” disease, terms that describe how many of the three major arteries (RCA, LAD, and CIRC) are narrowed significantly. Obviously, the more vessels that are narrowed, the worse is the clinical prognosis. LEFT MAIN disease is especially dangerous because this vessel supplies both of the arteries that supply blood to the left ventricle (LAD and CIRC).
Collateral Vessels
Occlusion of a large epicardial artery generally causes an infarct (defined as an area in which cells have died because of inadequate blood supply) whose borders are sharply demarcated from the adjacent normally perfused myocardium supplied by other, nonoccluded arteries. Intracoronary collateral vessels can connect the vascular beds supplied by different large epicardial arteries, such as the RCA, LAD, and CIRC. Although collateral vessels are usually poorly developed in younger individuals, in older patients, especially those with long-standing coronary atherosclerosis, intracoronary collaterals can enlarge and provide blood flow to regions of the heart downstream from
an occluded coronary artery. However, collaterals are not found at the level of the microcirculation, so that there is usually little or no “border zone,” defined as a region where a limited blood supply is preserved at the edge of a myocardial infarct (Factor et al., 1981).
an occluded coronary artery. However, collaterals are not found at the level of the microcirculation, so that there is usually little or no “border zone,” defined as a region where a limited blood supply is preserved at the edge of a myocardial infarct (Factor et al., 1981).
Blood Supply to the Ventricular Myocardium
Blood from the epicardial arteries reaches the myocardium via muscular branches that traverse the walls of the ventricles (Fig. 1-10); normal compression of these muscular branches during systole explains why virtually all nutrient coronary flow occurs during diastole, and why the subendocardial regions of the thick-walled left ventricle are especially vulnerable to coronary artery narrowing.
The left ventricular papillary muscles receive their blood supply from large penetrating vessels called perforators. The anterolateral papillary muscle, which supports the anterior leaflet of the mitral valve, has a dual blood supply derived from branches of the CIRC and the LAD. The posteromedial papillary muscle, which supports the posterior leaflet, receives its blood supply from the RCA or the CIRC via the PDA.
Blood Supply to the Conduction System
The SA node is perfused by the SA node artery (see Fig. 1-8), which in slightly more than half of human hearts is a branch of the RCA; in the remainder, this artery arises from the CIRC. The AV node is usually supplied by an AV node artery that is a branch of the PDA, so that the blood supply to the AV node is derived from the RCA in approximately 90% of human hearts and the CIRC in approximately 10%.
The AV bundle, along with proximal portions of both right and left bundle branches, is perfused by septal perforators that arise from both the LAD and the PDA. Because these critical conducting structures have a dual blood supply, the appearance of a conduction block in the AV bundle or bundle branches in a patient with an acute myocardial infarction implies that more than one major coronary artery is occluded. The anterior division of the left bundle branch and midportion of the right bundle branch are supplied by septal perforators arising from the LAD, whereas the posterior division of the left bundle branch is perfused by septal perforators supplied by the PDA.
Coronary Venous Drainage
The venous effluent of the heart is collected in large veins that parallel the epicardial coronary arteries. Most venous drainage of the left ventricle enters the coronary sinus, which parallels the CIRC in the left posterior AV groove before emptying into the floor of the right atrium. A portion of the venous drainage of the left ventricle, along with much of that derived from the right ventricle, enters the right atrium through anterior cardiac veins. A small fraction of the venous drainage of the ventricular myocardium flows directly into the cavities of the right and left ventricles by way of Thebesian veins.
Because the coronary sinus passes to the left behind the heart in the left AV groove, an electrode catheter inserted into this venous channel can be used to record the electrical activity of the left atrium and ventricle, stimulate these structures, and perform ablation therapy on the left side of the heart.
Fractal Anatomy of the Heart
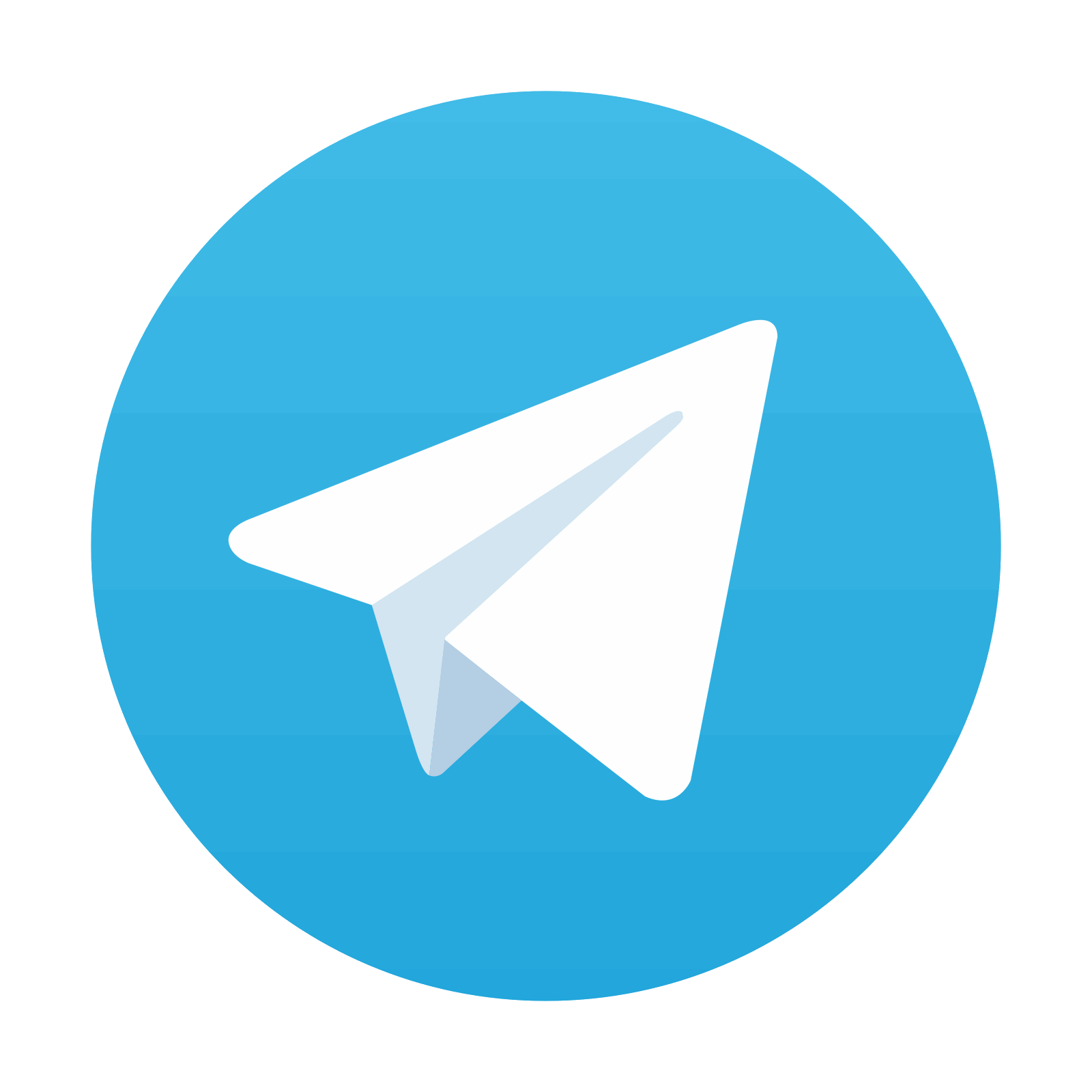
Stay updated, free articles. Join our Telegram channel

Full access? Get Clinical Tree
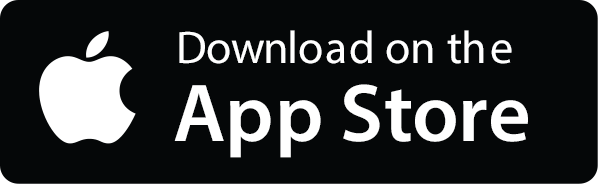
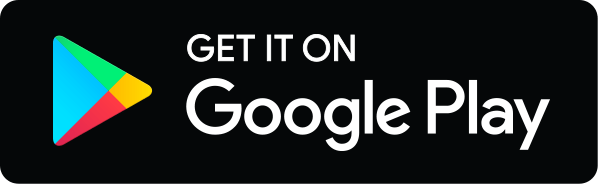