The Contractile Proteins
Cardiac contraction and relaxation depend on interactions among the six proteins listed in Table 4-1. This chapter describes each of these proteins, how they interact as actomyosins in vitro to cause the walls of the heart to shorten and develop tension, and how these interactions are regulated.
Myosin
Myosins, along with kinesins and dyneins, are “motor proteins” that participate in a variety of functions, including muscular contraction, cytokinesis, endocytosis, transport of cell organelles, signal transduction, and sensory functions such as hearing and vision. Like railway engines, the motor proteins move along tracks; in the case of myosin the interactions are with actin filaments, whereas kinesins and dyneins move along microtubules. All utilize chemical energy released during ATP hydrolysis to perform mechanical work.
Cardiac myosin is a tadpole-shaped molecule made up of two heavy chains and four light chains (Fig. 4-1). The “tail,” which is a “coiled coil” made up of two α-helical heavy chains wound around each other, provides rigidity to the thick filaments. The heavy chains contain paired “heads” which, along with the light chains, make up the cross-bridges that project from the thick filaments (see Chapter 1). Interactions between the cross-bridges, which contain the ATPase site of myosin, and actin filaments release the chemical energy that powers contraction.
Two fragments, called meromyosins, are released from myosin by the proteolytic enzymes trypsin and chymotrypsin. The smaller fragment, light meromyosin, is derived from the tail of the molecule, while the larger heavy meromyosin includes the head, a small portion of the tail, and the light chains (Fig. 4-2). Further digestion of heavy meromyosin with papain, another proteolytic enzyme, removes the rest of the tail, leaving a globular protein called heavy meromyosin subfragment 1 that includes the paired heads of the myosin heavy chains and the four light chains. Myosin ATPase activity and the ability to interact with actin are present in heavy meromyosin and heavy meromyosin subfragment 1.
Myosin forms filaments in vitro in which the cross-bridges project away from the center of these aggregates (Fig. 4-3). These structures, which resemble the thick filaments of striated muscle, allow polarized interactions between the cross-bridges and the thin filaments that enter the A-band from each of the two adjoining I bands. In resting hearts, where the thick and thin filaments are detached, the cross-bridges are nearly perpendicular to the long axis of the muscle (Fig. 4-4). Following activation, the cross-bridges attach and detach from actin in a series of steps that, like the oars of a racing shell, draw the thin filaments toward the center of the sarcomere. Because muscle volume remains virtually constant during contraction, the lateral distance between the thick and thin filaments is modified by changes in sarcomere length. The effects of
these changes in lattice spacing on tension development and shortening velocity are minimized by “hinges” in the myosin molecule (Fig. 4-1) which allow the cross-bridges to maintain contact with the thin filaments at short sarcomere lengths by varying the extent to which the myosin heads extend from the thick filaments (Fig. 4-5).
these changes in lattice spacing on tension development and shortening velocity are minimized by “hinges” in the myosin molecule (Fig. 4-1) which allow the cross-bridges to maintain contact with the thin filaments at short sarcomere lengths by varying the extent to which the myosin heads extend from the thick filaments (Fig. 4-5).
Table 4-1 Contractile Proteins of the Heart | |||||||||||||||||||||||||||||||||||
---|---|---|---|---|---|---|---|---|---|---|---|---|---|---|---|---|---|---|---|---|---|---|---|---|---|---|---|---|---|---|---|---|---|---|---|
|
![]() Fig. 4-1: Each myosin molecule contains two heavy chains and four light chains. The “tail” of the elongated molecule is a coiled coil (two α-helical chains wound around each other) made up of the two heavy chains; the latter continue into the paired “heads” that, along with the light chains, form the cross-bridge. Myosin has two points of flexibility, (“hinges”) at which proteolytic cleavage releases the meromyosins (Fig. 4-2). One lies below the heads, and the other divides the tail into two unequal lengths. |
Heavy Chains
Adult human atria and ventricles contain different myosin heavy chain isoforms (Table 4-2); additional isoforms are found in fetal and neonatal hearts. The human ventricle contains mainly a low ATPase β-myosin heavy chain along with a small amount (<10%) of a higher ATPase α-myosin
heavy chain. Human atria contain two atrial myosin heavy chain isoforms, both of which differ from those in the ventricles. Myocardial cells containing the α- and β-heavy chain isoforms are distributed in a “mosaic” pattern where different isoforms are found in adjacent cells (see Chapter 1). Chronic overload induces a hypertrophic response that is accompanied by an isoform shift in which the low ATPase heavy chain isoform replaces the high ATPase isoform (see Chapter 18).
heavy chain. Human atria contain two atrial myosin heavy chain isoforms, both of which differ from those in the ventricles. Myocardial cells containing the α- and β-heavy chain isoforms are distributed in a “mosaic” pattern where different isoforms are found in adjacent cells (see Chapter 1). Chronic overload induces a hypertrophic response that is accompanied by an isoform shift in which the low ATPase heavy chain isoform replaces the high ATPase isoform (see Chapter 18).
Table 4-2 Cardiac Myosin Heavy and Light Chains | |||||||||||||||||||||||||||||||||||||||
---|---|---|---|---|---|---|---|---|---|---|---|---|---|---|---|---|---|---|---|---|---|---|---|---|---|---|---|---|---|---|---|---|---|---|---|---|---|---|---|
|
Light Chains
Cardiac myosins contain two pairs of light chains, often referred to as essential and regulatory light chains (Table 4-2). These names refer to the fact that extraction of the essential light chains (also called LC1, ELC, MLC-1, or alkali-light chains) inactivates myosin, whereas the regulatory light chains (also called LC2, RLC, MLC-2, or DTNB– or EDTA-light chains) can be removed without abolishing myosin ATPase activity. All of these myosin light chains are members of the family of EF-hand calcium-binding proteins that includes troponin C and calmodulin (see below).
Five different light chain isoforms are found in human atrial and ventricular myosin. Two, LC1A and LC1V, are the essential light chains of atrial and ventricular myosin, respectively. The other three are regulatory light chains; two are found in the ventricles (LC2V and LC2V*) and one (LC2A) in the atria. In chronically overloaded ventricles, the atrial essential light chain LC1A replaces some of the LC1V. LC1A is also found in developing ventricles. The human cardiac myosin light chains do not bind calcium, but provide substrates for phosphorylations that regulate muscle shortening velocity.
Actin
Actin, a highly conserved protein found in all eukaryotic cells, received its name because of its ability to activate myosin ATPase activity. Actin can be stabilized in vitro as a monomer, called G-actin (G = globular), or as the filamentous F-actin polymer (F = fibrous). Both G- and F-actin contain nucleotide- and cation-binding sites. The bound nucleotide in G-actin is ATP while F-actin ordinarily contains bound ADP; in both, the bound cation can be either calcium or magnesium. Actin monomers, which are much smaller than myosin (Table 4-1), are ovoid globular proteins that are ∼55 á in diameter. The polymer, a macromolecular helix in which two chains of actin monomers are wound around one another like two strings of beads, makes up the backbone of the thin filaments (Fig. 4-6) and represents one of the three types of cytoskeletal filament described in Chapters 1 and 5.
![]() Fig. 4-7: Tropomyosin is located in the groove between the two strands of F-actin in the thin filament. |
Two actin isoforms are found in the human heart, α-cardiac actin and α-skeletal actin. Adult human ventricles contain mainly α-cardiac actin; α-skeletal actin, which is present in smaller amounts, is the fetal isoform.
Actomyosins reconstituted in vitro from purified actin and myosin can hydrolyze ATP and undergo physicochemical changes similar to those that occur during muscle contraction. However, these two-protein actomyosins are not regulated by calcium because they lack the regulatory proteins (see below).
Tropomyosin
Tropomyosin is a homodimer or heterodimer made up of two α-helical peptide chains, called α- and β-tropomyosin. Although this protein had no known function for the first 15 years after it was first purified, its structure was of interest to physical chemists because, like the tail of the myosin molecule, it is a rigid coiled-coil. Tropomyosin itself has no biological activity, but when incorporated into reconstituted actomyosins, this seemingly inert protein regulates the interactions between myosin and actin. These regulatory effects arise from shifts in the position of tropomyosin in the grooves between the two F-actin chains in the thin filament (Fig. 4-7). The activity of the cardiac contractile proteins is inhibited when p38-MAPK, a stress-activated mitogen-activated protein kinase (see Chapter 9), causes a regulatory serine in tropomyosin to be dephosphorylated.
The Troponin Complex
The troponin complex includes three proteins (Table 4-1) that, along with tropomyosin, are found in the thin filaments (Figs. 4-8 and 4-9). Troponin I received its name because its most important effect is to inhibit actin–myosin interactions, troponin T binds the troponin complex to tropomyosin, and troponin C contains the calcium-binding sites that participate in excitation-contraction coupling and relaxation.
![]() Fig. 4-9: Cross section of the thin filament in resting muscle at the level of a troponin complex, showing relationships between actin, tropomyosin, and the three components of the troponin complex. |
Troponin I
Changes in the strength of a labile bond linking troponin I and actin allow calcium to regulate cardiac contraction. In the relaxed heart, where troponin C is not bound to calcium (see below), troponin I binds tightly to actin in a conformation that causes tropomyosin to “block” the myosin-binding sites of actin. Calcium binding to troponin C induces an allosteric change in the thin filament that loosens the bond linking troponin I to actin, which allows active sites on actin to interact with the myosin cross-bridges. In this way, changes in the actin-binding affinity of troponin I provide a molecular switch that recognizes a rise in cytosolic calcium as a signal to initiate contraction (see below).
Different troponin I isoforms are found in cardiac, fast skeletal, and slow skeletal muscles; an additional troponin I isoform has been identified in developing muscle. Cardiac troponin I contains a regulatory serine that, when phosphorylated by protein kinase A [PKA, cyclic adenosine monophosphate (cAMP)-dependent protein kinase], reduces the calcium sensitivity of troponin C; this posttranslational response accelerates relaxation during sympathetic stimulation by favoring dissociation of activator calcium from the contractile proteins (see Chapter 10). Protein kinase C (PKC) also catalyzes the phosphorylation of cardiac troponin I, but the consequences of this posttranslational change are not well understood.
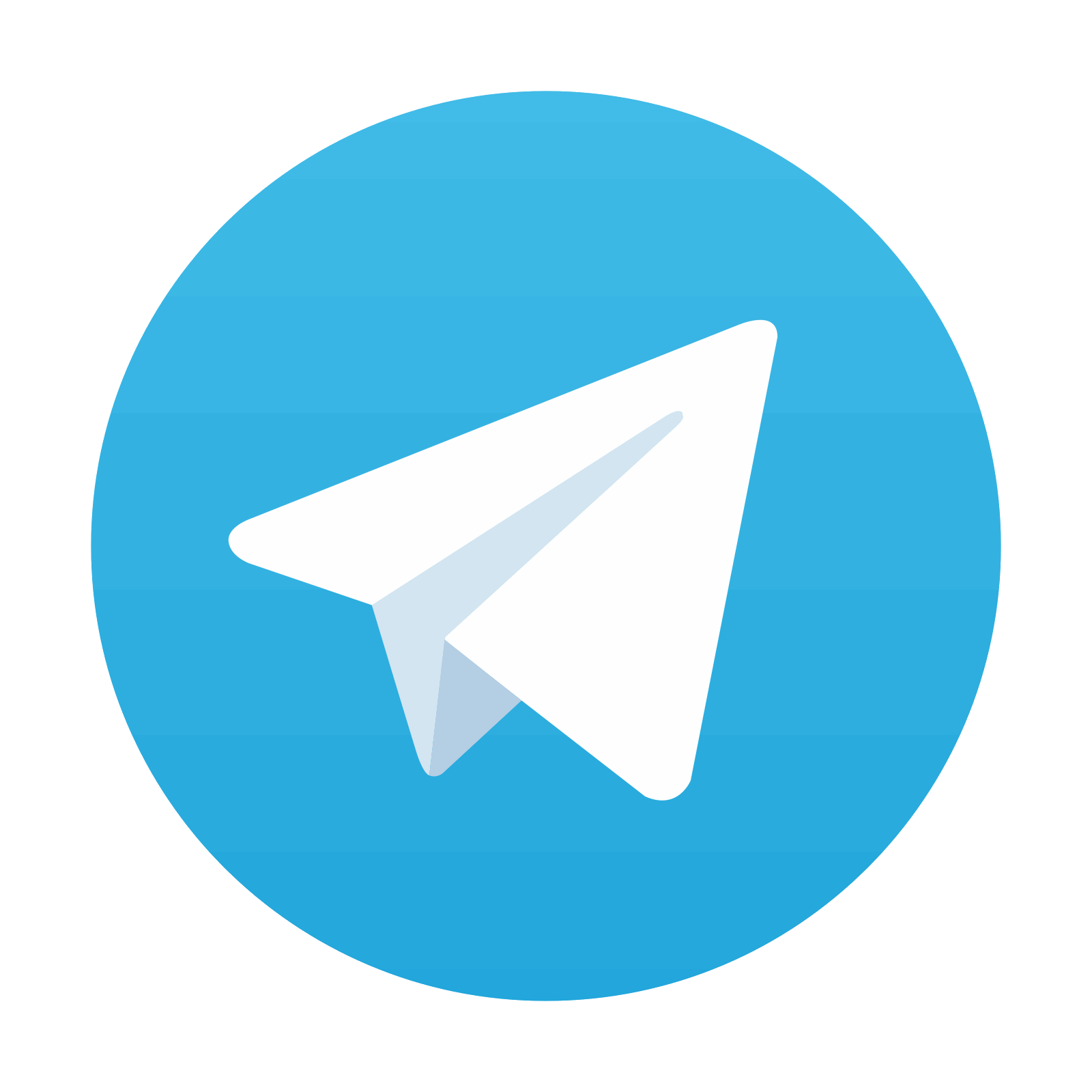
Stay updated, free articles. Join our Telegram channel

Full access? Get Clinical Tree
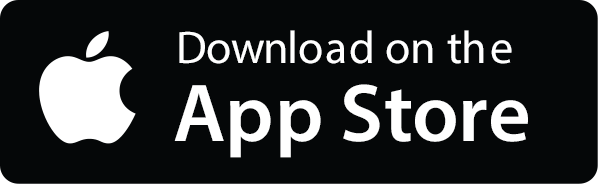
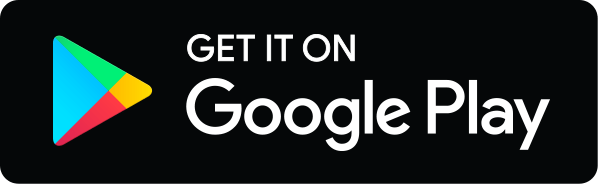