Fig. 12.1
Static elastic recoil decreases throughout life starting around the age of 20. TLC total lung capacity. Shaded area represents mean ± 1 SD(This material has not been reviewed by the European Respiratory Society prior to release; therefore, the European Respiratory Society may not be responsible for any errors, omissions, or inaccuracies or for any consequences arising therefrom, in the content. Reproduced with permission of the European Respiratory Society ©: Janssens et al. [7])
Age-Related Changes in Mechanics of Breathing
Chest Wall and Respiratory Muscles
The chest wall progressively stiffens with aging because of structural changes of the intercostal muscles, intercostal joints, and rib-vertebral articulations, leading to a decrease in static chest wall compliance [7, 9]. The increase in the rigidity of the rib cage is secondary to multiple factors, including changes in rib-vertebral articulations, changes in the shape of the chest (mainly because of osteoporosis that increases both dorsal kyphosis and anteroposterior chest diameter), costal cartilage calcification, and narrowing in the intervertebral disk spaces [7, 9].
The changes in the chest wall geometry with aging result in flattening of the diaphragm curvature (Fig. 12.2) [1], which has a negative effect on the maximal transdiaphragmatic pressure [1, 7]. A reduction in muscle mass contributes to a decrease in the force produced by respiratory muscles. In a healthy 70-year-old individual, maximal skeletal muscle electromyographic activity is reduced by approximately 50% [10]. In frail or malnourished elderly patients, respiratory muscle strength may be further affected [11, 12]. The main consequence of the reduction in the maximal transdiaphragmatic pressure is predisposition of the diaphragm to fatigue in the presence of increased ventilatory load; [13] this could manifest clinically by difficulty weaning an elderly patient from the ventilator .


Fig. 12.2
Aging-induced reduction of elastic recoil results in an enlargement (barrel shaped) of the thorax and flattening of the diaphragm . The flatter diaphragm is less efficient in generating muscle power which increases the work of breathing. The loss of elastic recoil results in narrowing of small airways. Left panel, juvenile lung; right panel, aged lung (Reprinted from Zaugg and Lucchinetti [1]. With permission from Elsevier)
Lung Parenchyma
Lung compliance increases with aging primarily because of the loss in parenchymal elasticity (Table 12.1) [7, 8]. As a result, elastic recoil pressure of the lungs decreases with age (Fig. 12.1) [7, 8, 14]. The presumed mechanism for this decrease in elasticity is changes in the spatial arrangement and/or cross-linking of the elastic fiber network [9]. Changes in lung parenchyma become pronounced after 50 years of age, resulting in a homogeneous enlargement of air spaces and a reduction of alveolar surface area from 75 m2 at age 30 to 60 m2 at age 70 [9]. Because these changes functionally resemble emphysema, they are sometimes referred to as “senile emphysema ” [6, 15].
Table 12.1
Changes in respiratory function associated with aging and pathophysiologic mechanisms that explain perioperative complications
Function alteration | Change | Pathophysiology | Potential complications |
---|---|---|---|
Upper airway patency | ↓ | Hypotonia of hypopharyngeal and genioglossal muscles, obesity (redundant tissues) | Upper airway obstruction and OSA |
Swallowing reflexes and cough | ↓ | ↓ Clearance of secretions | Aspiration risk, inefficient expectoration, pneumonia, atelectasis, hypoxemia |
Chest wall compliance | ↓ | Structural changes of the intercostal muscles and joints and rib-vertebral articulations | ↑ Work of breathing, delayed weaning from mechanical ventilation |
Airway resistance | ↑ | ↓ Diameter of small airways | Air trapping, propensity for developing intraoperative atelectasis; ↓ maximal expiratory flow (airflow limitation) during exercise |
Lung compliance | ↑ | ↓ Lung static elastic recoil pressure | Air trapping, potential for dynamic hyperinflation during mechanical ventilation |
Closing volume | ↑ | Closing of small airways, sometimes within normal tidal volume breathing | Intraoperative hypoxemia, especially with ↓ FRC airflow limitation |
Gas exchange | ↓ Oxygenation | ↑ Ventilation/perfusion heterogeneity and ↓ diffusing capacity | Hypoxemia |
Gas exchange | ↔ In CO2 | ↑ In dead space ventilation counteracted by ↓ in CO2 production because of ↓ in basal metabolic rate | |
Exercise capacity | ↓ From deconditioning | ↓ VO2 max because of ↓ in cardiac output | Associated with higher incidence of postoperative pulmonary complications |
Regulation of breathing | ↓ | Dysfunction of central chemoreceptors and peripheral mechanoreceptors | ↓ Ventilatory response to hypoxemia. Risk of hypercarbia and hypoxemia during use of opioids |
Spirometry : Static and Dynamic Tests and Underlying Physiology
All lung volumes increase from birth until somatic growth stops. Age has interesting effects on total lung capacity (TLC) , the net effect being only slight changes with increasing age. TLC is correlated with height. With advancing age, height diminishes because of vertebral changes (e.g., flattening of the intervertebral disks, compression fractures), and TLC reduces but, if normalized for height, remains unchanged (Fig. 12.3) [7, 16, 17]. The age-associated effects of the loss of inward elastic recoil and decline in the chest wall outward force are typically balanced so that TLC remains unchanged [9]. Because TLC remains relatively stable with age, changes in other measured lung volumes and capacities offset each other and are balanced. An understanding of these changes helps to explain the decline in pulmonary function .


Fig. 12.3
Evolution of lung volumes with aging. TLC total lung capacity, VC vital capacity, IRV inspiratory reserve volume, ERV expiratory reserve volume, FRC functional residual capacity, RV residual volume. Aging produces an increase in RV with consequent reduction in ERV and VC, without changing TLC (This material has not been reviewed by the European Respiratory Society prior to release; therefore, the European Respiratory Society may not be responsible for any errors, omissions, or inaccuracies or for any consequences arising therefrom, in the content. Reproduced with permission of the European Respiratory Society ©: Janssens et al. [7])
The reduction of the alveolar surface area results in a gradual increase in the residual volume (RV) with an increase of 5–10% per decade [18]. The RV/TLC ratio increases from 25% at 20 years to 40% in a 70-year-old subject. The increase in RV results in a compensatory decrease of vital capacity (VC) ; after age 20, VC decreases 20–30 mL per year [18]. Functional residual capacity (FRC) is determined by the balance between the inward recoil of the lungs and the outward recoil of the chest wall. FRC increases by 1–3% per decade (Fig. 12.3) because at relaxed end-expiration, the rate of decrease in lung recoil with aging exceeds that of the rate of increase in chest wall stiffness [18, 19].
Forced expiratory volume in 1 s (FEV1) and forced VC (FVC) increase up to 20 years of age in females and up to 27 years of age in males, followed by gradual decrease (up to 30 mL per year) (Fig. 12.4) [9, 20, 21]. After 65 years of age, this decline may accelerate (38 mL per year) [22]. Smoking dramatically accelerates these age-related changes in FEV1 and FVC [23]. In healthy, elderly subjects from 65 to 85 years of age, the normal FEV1/FVC ratio may be as low as 55%, compared with expected ≥70% in younger individuals [24]. Lung volume is a major determinant of airway resistance, but, when adjusted for age-related change in mean lung volume, aging has no significant effect on airway resistance [25]. A decrease in small airway diameter with aging, associated with reduced mean lung volume (Fig. 12.2, Table 12.1), contributes to a decrement in maximal expiratory flow with aging [26], present even in lifetime nonsmokers [26].


Fig. 12.4
Effect of aging on FEV1 (forced expiratory volume in 1 s) and FVC (forced vital capacity) in males and females. Both progressively decline after 20 years of age (Reprinted from Burrows et al. [20]. With permission from Elsevier)
Airway Closure Concept (Closing Volume)
The loss of elastic recoil [7, 16] also affects the caliber of intrathoracic airways (Fig. 12.2) [27]. These airways are kept open by the transpulmonary pressure gradient (Ptp), i.e., the pressure gradient from inside the airway (0 cmH2O) to the pleural space (-10 cmH2O) (Fig. 12.5A) [28]. When the patient exhales, active contraction of the expiratory muscles generates a pleural pressure that is above atmospheric (+10 cmH2O, Fig. 12.5B) [28]. The pressure inside the airway decreases downstream due to flow resistance, and at some point the intraluminal pressure equals the pleural pressure (“equal pressure point ,” * in Fig. 12.5B). Downstream compression of airways limits the effectiveness of the expiratory muscles and sets a maximal flow rate for each lung volume (“airflow limitation ”) [29–31].


Fig. 12.5
Effects of pleural pressure on airway diameter at rest (a) and during expiration (b). Units shown are cmH2O. Intrapleural pressure is -10 cmH2O at rest but increases to +10 cmH2O during exhalation. This change in intrapleural pressure compresses the alveoli which increases the airway pressure. During exhalation this increased intrapleural pressure compresses the airways narrowing their lumen and resulting in increased flow resistance. This results in decreasing airway pressure downstream from the alveoli. The point where pleural pressure equals intraluminal bronchiolar pressure is called the “equal pressure point ” and results in airway narrowing with airflow limitation. The lung volume at which this occurs to a significant extent is called the closing volume (Reprinted from Shields et al. [161]. With permission from Wolters Kluwer Health)
With aging-induced loss of lung elastic recoil pressure , flow limitation occurs at higher lung volumes compared with younger subjects. This expiratory airflow limitation in elderly subjects causes a significant alteration of the ventilatory response to exercise compared with younger adults (Fig. 12.6) [32, 33]. Older subjects have less reserve to accommodate the increased ventilatory demand of exercise because of increased airflow limitation [33]. During similar levels of maximal exercise (minute ventilation of 114 L/min), 45% of the tidal volume of the 70-year-old subject is flow limited because of airway compression, in comparison to less than 20% in the 30-year-old untrained adult (Fig. 12.6) [32]. Despite these limitations, arterial Pco2 and Po2 are well maintained, even during maximal exercise.


Fig. 12.6
Flow limitation with progressive maximal exercise in 30-year-old untrained adults and in 70-year-old adults. At a given minute ventilation, the incidence of flow limitation during tidal breathing is greater in the elderly than in the young (Adapted from Johnson et al. [32]. With permission from Elsevier)
Because of the existence of vertical gradient in transpulmonary pressure , airway closure occurs earlier in dependent lung regions. The volume of lung when small airways in the dependent parts of the lung begin to collapse during expiration is termed “closing volume.” Subsequent research has shown that this closing volume concept is an oversimplification of a more complex process. Nonetheless, this concept is a useful means of conceptualizing lung behavior at low volumes. Because lung static recoil decreases with age, closing volume increases with age. In younger subjects, closing volume is less than FRC , and the airways remain open during resting tidal volume breathing. The increases in FRC with aging are less than the increases in closing volume, such that in erect subjects without lung disease, the closing volume starts to exceed FRC around the age of 65 [26]. Because FRC decreases when a subject assumes the supine position, airway closure may be present during resting tidal volume breathing, and this typically occurs around the age of 45. Airway closure during tidal breathing can lead to gas-exchange abnormalities (discussed below); indeed, changes in closing volume with age are correlated with hypoxemia [1].
The Effects of Aging on Gas Exchange
The efficiency of alveolar gas exchange decreases with age. One explanation is an imbalance in the ventilation/perfusion ratio mainly caused by increases in physiologic dead space and shunting [34, 35]. This imbalance leads to a gradual decrease in arterial Po2 with aging (Fig. 12.7) [19, 36, 37]. At the same time, once arterial Pco2 reaches 40 mmHg in the newborn, it remains virtually constant for the remainder of life, and CO2 elimination remains unaffected despite an increase in dead space ventilation [38] and reduction in CO2 sensitivity with aging. The latter is attributable at least in part to a decline in CO2 production associated with a decrease in basal metabolic rate. Multiple factors contribute to the decline in arterial Po2 related to age. In young, seated subjects breathing air at rest, the alveolar-arterial pressure difference for oxygen (A-aDO2) is between 5 and 10 mmHg. An increase in the A-aDO2 occurs with age because of an increase in ventilation/perfusion heterogeneity, thought to be caused by a decrease in alveolar surface area and increase in closing volume [39]. Additionally, increased body mass index, as seen with obesity, that frequently accompanies aging, can contribute to the widening of A-aDO2 . After 75 years of age, arterial oxygen tension remains relatively stable at around 83 mmHg [40]. The diffusing capacity of the lungs decreases with aging [41] at a rate between 0.2 and 0.3 mL/min/mmHg/year [19], with this decline being more pronounced after the age of 40. This deterioration is attributed to an increase in ventilation/perfusion mismatching, decline in pulmonary capillary blood volume [41], and/or the loss of the alveolar surface area [42].


Fig. 12.7
Arterial oxygenation (Po2) as a function of age from birth to 80 years. Note the decline in arterial Po2 after the age of 20 (Reprinted from Murray [19]. With permission from Elsevier)
Aging and Exercise Capacity
Age is a significant factor determining maximal O2 uptake (VO2 max). VO2 reaches a peak between 20 and 30 years of age and then decreases at a rate of 9% per decade (Fig. 12.8) [19, 43]. The VO2 max decrease is more pronounced in sedentary elderly subjects than in the physically active [44]. In elderly individuals who maintain athletic exercise, the decline in VO2 max is slowed. Factors that limit the VO2 max in the elderly include a decrease in maximal minute ventilation, decrease in the maximum arterial-venous O2 content difference, decrease in O2 extraction by the tissues, and reduced peripheral muscle mass. The decrease in O2 transport capacity during senescence is also linked to an age-related decrease in cardiac output. The O2 cost of breathing (i.e., proportion of O2 consumption by respiratory muscles) is higher than in younger subjects. Also, compared with younger individuals, the elderly are more responsive to CO2 during exercise; for a given CO2 production, the ventilatory response increases with aging, unrelated to oxyhemoglobin desaturation or increase in metabolic acidosis [44].


Fig. 12.8
Maximal oxygen uptake (VO2 max) measured during maximal exercise as a function of age. Note the decline in VO2 max starting near 30 years of age (Reprinted from Murray [19]. With permission from Elsevier)
Regulation of Breathing
In humans, ventilation is adjusted by inputs from different chemoreceptors that respond to metabolic factors and by inputs from mechanoreceptors that provide feedback from the chest wall, lungs, and airways. Minute ventilation at rest is similar in young and elderly subjects, but tidal volumes are smaller and respiratory rates are higher in the elderly [45]. The mechanism is not fully understood, but it may represent an adaptation to decreases in chest wall compliance, as well as changes in the function of central chemoreceptors and peripheral mechanoreceptors in the chest wall and lung parenchyma [46]. Compared with younger subjects, elderly individuals have approximately 50% and 60% reduction in the ventilatory response to hypoxia and hypercapnia, respectively [47]. Moreover, studies have shown that the average increase in ventilation in response to an alveolar pressure of oxygen of 40 mmHg in older men is 10 L/min, in contrast to 40 L/min for younger individuals [48]. Responses to normocapnic hypoxemia during sleep can be even more depressed. For example, elderly individuals may not arouse from the REM phase of sleep until their oxyhemoglobin saturation decreases below 70%. Although in elderly subjects the ventilatory response to hypercapnia is blunted compared with younger subjects, the ventilatory response to exercise is actually increased: for a given CO2 production during exercise, the ventilatory response increases with aging compared with younger individuals [44]. This cannot be explained by either increased anaerobiosis or oxyhemoglobin desaturation, but it seems that increased ventilation in the elderly compensates for increased inefficiency of gas exchange, allowing for the maintenance of normocapnia during exercise [49].
Other respiratory control mechanisms may be altered in the elderly because of reduced efficiency in distinguishing respiratory stimuli and/or altered integration of perception of stimuli within the central nervous system [50–52]. The elderly also have a lesser ability to perceive methacholine-induced bronchoconstriction [41]. The loss of important protective and adaptive mechanisms, which may result in lesser awareness of disease and delayed diagnosis of pulmonary dysfunction in the elderly, is influenced by the blunted response to hypoxia and hypercapnia and a lower ability to perceive disease states such as bronchoconstriction.
Upper Airway Dysfunction
Hypotonia of the hypopharyngeal and genioglossal muscles predisposes elderly subjects to upper airway obstruction, and the prevalence of sleep-disordered breathing increases with age [53]. Studies have found that up to 75% of subjects over 65 years old have obstructive sleep apnea (OSA) [54, 55]. Some of the consequences of chronic hypoxemia associated with OSA may include cognitive impairment, personality changes, and hypertension [55]. OSA may be even more prevalent in elderly obese individuals, who may have increased postoperative risk of respiratory complications [56].
The protective mechanisms of cough and swallowing are altered in elderly individuals, which may lead to ineffective clearance of secretions and increased susceptibility to aspiration. Mucociliary transport is also impaired in the elderly. Coughing is also less efficient in terms of volume, force, and flow rate. The loss of protective upper airway reflexes is presumably attributable to an age-related alteration in peripheral signaling together with decreased central nervous system reflex activity [58]. In addition, elderly individuals have an increased prevalence of neurologic diseases that may be associated with dysphagia and an impaired cough reflex leading to the increased likelihood of pulmonary aspiration [57] and pneumonia [58], which may have a significant impact on perioperative morbidity and mortality .
Perioperative Pulmonary Complications in the Elderly
With increased longevity , more elderly patients are potential candidates for major surgical procedures. For example, in 1997 in the United States, the Agency for Healthcare Policy and Research reported 1,350,000 major procedures in the 65- to 84-year-old age group and 233,000 procedures in the 85 and older age group [59]. Postoperative pulmonary complications, including atelectasis, pneumonia, respiratory failure, and exacerbation of underlying chronic lung disease, have a significant role in the risk for anesthesia and surgery [60]. These complications have been reported in 5–10% of the general patient population [61] and usually prolong the hospital stay by an average of 1–2 weeks [62]. Pulmonary complications in nonthoracic surgery are as prevalent as cardiovascular complications and contribute in a similar manner to morbidity, mortality, and length of stay [63, 64].
Numerous factors may contribute to the development of postoperative pulmonary complications in the elderly (Table 12.2) [65]. Advanced age is a significant independent predictor of pulmonary complications even after adjustment for various comorbid conditions [60, 63, 66]. Age increases the risk of pulmonary complications with an odds ratio of 2.1 for patients 60–69 years old and 3.0 for those 70–79 years old compared with patients younger than 60 years [60, 63, 67]. Older age represents the second most common identified risk factor for pulmonary complications after the presence of chronic lung disease [60, 66, 68]. A multifactorial risk index for predicting postoperative respiratory failure in men after major noncardiac surgery [69] showed that age above 70 conferred a 2.6-fold increase in the risk of respiratory failure compared with subjects less than 60 years old.
Table 12.2
Risk factors for postoperative pulmonary complications
Patient characteristics | Preoperative testing | Surgery | Anesthetic management |
---|---|---|---|
Age | Low albumin | Open thoracic surgery | General anesthesia |
Male sex | Low Spo2 (≤95%) | Cardiac surgery | High respiratory driving pressure (≥13 cm H2O) |
ASA class ≥3 | Anemia (Hb < 10 g/dl) | Open upper abdominal surgery | High inspiratory oxygen fraction |
Previous respiratory infection | Major vascular surgery | High volume of crystalloid administration | |
Functional dependency | Neurosurgery | Erythrocyte transfusion | |
Congestive heart failure | Urology | Residual neuromuscular blockade | |
COPD | Duration of surgery >2 h | Nasogastric tube use | |
Smoking | Emergent surgery | ||
Renal failure | |||
Gastroesophageal reflux disease | |||
Weight loss |
Factors contributing to an increased risk of pulmonary complications in the elderly are (a) decreases in chest wall compliance and muscle strength (increasing the work of breathing and the risk for respiratory failure); (b) changes in lung mechanics (including increased tendency for small airway closure which may impair gas exchange and promote atelectasis); (c) increased aspiration risk secondary to swallowing dysfunction; and (d) alterations in the control of breathing, including impaired responses to hypercapnia and hypoxia and increased sensitivity to drugs used during anesthesia (especially opioids) [70].
Intraoperative alterations in chest wall function lead to atelectasis, which forms within minutes after the induction of anesthesia and is an important cause of intraoperative gas-exchange abnormalities. Chest wall dysfunction persists into the postoperative period because of pain (which limits the voluntary actions of the chest wall muscles), reflex inhibition of the respiratory muscles, and mechanical disruption of respiratory muscles (surgery in the thoracic and abdominal cavities). Consequently, after thoracic or upper abdominal surgery, FRC and VC decrease, and breathing becomes rapid and shallow, all of which may contribute to the development of pulmonary complications [71]. These effects apply to all ages but may be of special significance in the elderly patient with reduced respiratory reserve.
Older, nonanesthetized individuals have less efficient gas exchange compared with younger subjects [36]. Upon assuming the supine position, there is a decrease in FRC and hence an increase in airway resistance, which is more marked in the elderly (especially those who are obese) [36]. Alveolar gas exchange during anesthesia is less efficient in the elderly, and there is an inverse relationship between increased age and arterial Po2 in spontaneously breathing anesthetized patients [37, 39]. After the induction of anesthesia, atelectasis develops in dependent lung regions and may produce significant shunting. However, both the amount of atelectasis and pulmonary shunting do not increase significantly with age [72, 73]. A similar phenomenon occurs in patients with chronic obstructive pulmonary disease (COPD) ; after the induction of anesthesia, there is less formation of atelectasis and less shunting compared with normal patients, which is explained by changes in the chest wall secondary to hyperinflation that prevents alveolar collapse [74].
Decreased respiratory muscle strength, combined with diminished cough and swallowing reflexes (e.g., neurologic disorders, stroke), may diminish clearance of secretions and increase the risk of aspiration in the elderly [75, 76]. This risk is even higher in the presence of gastroesophageal reflux, which is also more prevalent in the elderly. Selective, rather than routine, nasogastric tube decompression after abdominal surgery has been proposed to improve the return of bowel function and reduce the risk of postoperative pulmonary complications, specifically a lower rate of atelectasis and pneumonia [77, 78]. Interestingly, the aspiration rate was not lower in patients with selective nasogastric decompression [78]. Finally, age-related changes in control of breathing, increased sensitivity to anesthetic agents, and diminished response to gas-exchange abnormalities predispose elderly patients to postoperative respiratory failure. Elderly patients also have a higher incidence of postoperative sleep apnea episodes [70, 79].
General Health Status
Multiple measures of functional status and general health predict the risk of postoperative pulmonary complications. An American Society of Anesthesiologists Physical Status Classification above II, poor exercise capacity, the presence of COPD , and congestive heart failure are all associated with increased risk of pulmonary complications in the elderly [63, 80, 81]. COPD is more prevalent in the elderly population and is the most important patient-related risk factor for the development of postoperative pulmonary complications, producing a three- to fourfold increase in relative risk [66, 81, 82]. Although obesity is prevalent in elderly patients and is associated with decreased perioperative arterial oxygenation, obesity is not a significant independent predictor of risk [63, 80, 83].
Decreased functional status, which may accompany aging, is an independent risk factor for pulmonary complications [63]. Objective measurement of exercise capacity in geriatric patients demonstrated that inability to perform 2-min supine bicycle exercise and an increase in the heart rate to above 99 beats/min were the best predictor of perioperative cardiopulmonary complications in patients older than 65 years undergoing elective abdominal or noncardiac thoracic surgery [84]. Patients with better exercise tolerance by self-report, better walking distance, or better cardiovascular classification had lower rates of postoperative pulmonary complications [85].
Strategies Used to Minimize Pulmonary Risk in Elderly Patients: Preoperative Considerations
Preoperative Testing
The value of routine preoperative pulmonary function testing is controversial. For lung resection surgery, the results of pulmonary function testing, including measurement of arterial blood gases, have proven useful in predicting pulmonary complications and postoperative function; however, spirometry does not predict postoperative pulmonary complications after abdominal surgery [85, 86]. The degree of airway obstruction assessed by spirometry does not represent an independent risk factor for postoperative respiratory failure, even in smokers with severe lung disease [87]. Spirometry , chest radiograms, and arterial blood gases should be obtained as indicated from the history and physical examination as a part of this evaluation, but should not be routinely ordered [78].
There is a high prevalence of unrecognized OSA in the surgical population [88]. In 2006 the American Society of Anesthesiologists published guidelines recommending a thorough preoperative evaluation for all surgical patients [89]. Overnight polysomnography is the gold standard to make the diagnosis of OSA but is impractical for widespread screening of all surgical patients [90]. Overnight pulse oximetry can be used as a screening tool but lacks diagnostic accuracy [91]. A practical option, being adopted by an increasing number of practices, is the use of a preoperative screen to assess OSA risk. The STOP BANG (snoring, tiredness, observed apneas, high blood pressure, BMI >35 kg/m2, age > 50 years, neck circumference > 40 cm, and male sex, with a score of 3 positives indicating moderate/high risk of OSA) OSA assessment tool has been widely used with positive and negative predictive values of 81.0 and 60.8% for OSA and 31.0 and 100% for severe OSA, respectively [92]. Anesthetic management of elderly patients with a history or positive screen of OSA can be tailored to decrease postoperative respiratory depression (see below).
Preoperative Therapies
To minimize postoperative pulmonary complications in elderly patients, it is important to optimize the respiratory status, beginning with a careful assessment of general physical status and particular attention to the cardiopulmonary system. Specific therapy should be instituted preoperatively if such treatment is likely to result in improved functional status, so long as the therapeutic benefit outweighs any risk from surgical delay (Fig. 12.9). For example, obstructive sleep apnea (OSA) is prevalent and often undiagnosed in elderly patients because aging may be blamed for many OSA symptoms (i.e., snoring, tiredness, unintended napping) [93]. Identification of these undiagnosed OSA patients and starting continuous positive airway pressure (CPAP) therapy preoperatively may improve respiratory function and outcomes [94]. Interestingly, the use of CPAP in nonsurgical ≥65-year-old patients with recently diagnosed severe OSA improves cognitive function (episodic and short-term memory, speed of mental processing, and mental flexibility) compared to conservative care [95]. Whether perioperative CPAP improves cognitive function in aging surgical patients with OSA has not been explored.


Fig. 12.9
Perioperative strategies used to minimize pulmonary complications
Preoperative spirometry should be used only to monitor the degree of therapeutic response to treatments such as bronchodilators used to treat reactive airway disease. Patients with a reversible component of airway obstruction must be treated with bronchodilators and/or corticosteroids. Antibiotics must be given if a pulmonary infection is suspected. Preoperative smoking cessation may decrease postoperative pulmonary complications, and all patients who smoke should be given help to quit [96, 97]. Past studies have been interpreted as demonstrating that quitting within a few weeks of surgery actually increases pulmonary complications by stimulating mucous production [98]. However, careful review of these studies and more recent data show that, although it may take several weeks of abstinence before pulmonary outcomes are improved, brief abstinence does not worsen outcomes [97, 98]. Thus, this consideration should not prevent practitioners from promoting preoperative abstinence from smoking, even for a brief period before surgery .
Strategies Used to Minimize Pulmonary Risk in Elderly Patients: Intraoperative Considerations
Surgical Considerations
The surgical site is the most important risk factor for the development of postoperative pulmonary complications and outweighs other patient-related risk factors [67, 69]. There is a higher likelihood of pulmonary complications with incisions closer to the diaphragm because of diaphragmatic dysfunction , splinting, and decreased ability to take deep breaths. For example, pulmonary complications caused by upper abdominal surgeries range from 13% to 33% as compared with lower abdominal surgeries that range from 0% to 16% [80]. Duration of surgery also has a significant role in the development of pulmonary complications, and surgeries that last more than 3 h have an increased risk of pulmonary complications [99]. When surgically feasible, laparoscopic techniques should be considered; however, significant respiratory dysfunction can occur even after laparoscopically performed operations [78], and whether laparoscopic procedures may reduce the risk of clinically important pulmonary complications is not clear. Nonetheless, other considerations such as reduced postoperative pain and length of stay often favor the use of laparoscopic techniques. Placement of an aortic stent instead of an open aortic aneurysm repair may be desirable in patients with significant pulmonary comorbidity.
Induction of Anesthesia
Preoxygenation is recommended before the induction of general anesthesia. In contrast to younger patients, performing only four deep breaths before the induction may not be sufficient in elderly patients, who may require a full 3 min of 100% oxygen breathing to avoid oxyhemoglobin desaturation during rapid sequence induction [100]. In patients with intact oropharyngeal reflexes but significant reactive airway disease, avoidance of endotracheal intubation by using a laryngeal mask airway may be desirable.
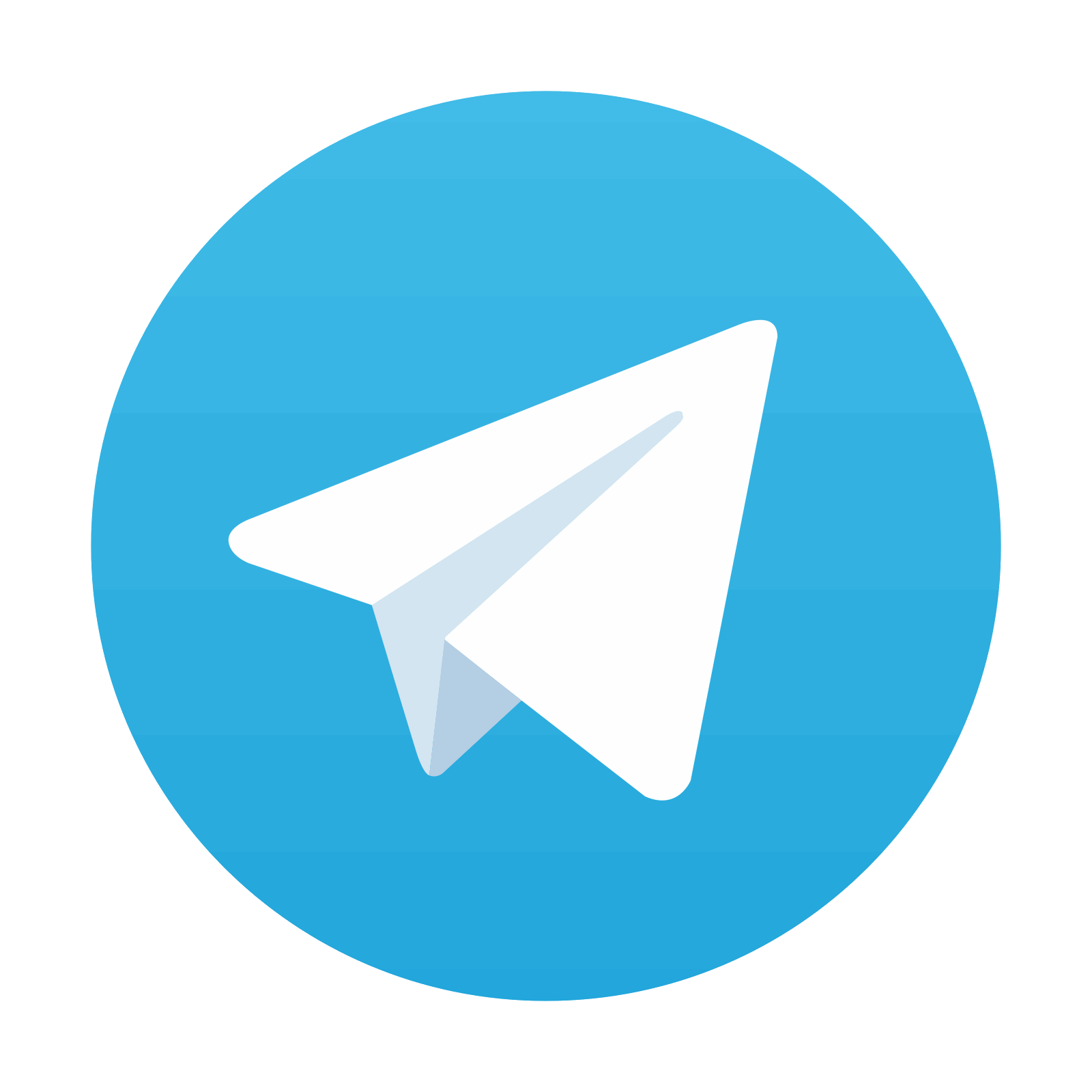
Stay updated, free articles. Join our Telegram channel

Full access? Get Clinical Tree
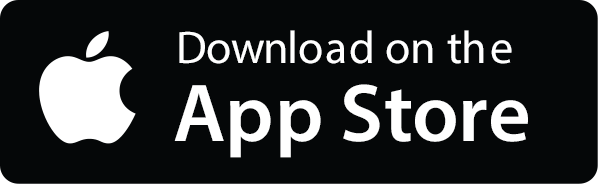
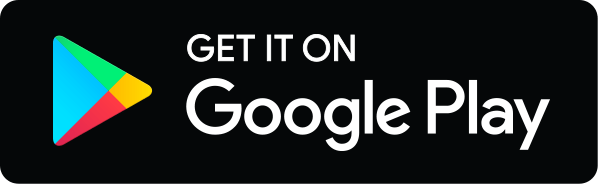