Clinical traits
Treatment
Expected benefits of treatment
Pulmonary
Fixed airflow obstruction
Variable airflow obstruction
Airway inflammation
Alveolar hypoplasia
Focal parenchymal defects
Reduced exercise performance
Pulmonary hypertension
Sleep-disordered breathing
Abnormal control of breathing
Bronchodilators
Stop inappropriate ICS
Exercise prescription or pulmonary rehabilitation
Ensure normoxia
CPAP if needed (rare)
Ensure normoxia
Better lung function
Reduce side effects
Well-being improved
Prevent progression
Improved symptoms
Prevent progression
Extrapulmonary (comorbidities)
Neurological
reflux and aspiration
Abnormal swallow
Retinopathy
Bone disease
Renal disease
Rehabilitation services
Reflux medication
Thicken liquids or PEG
Better QoL
Airway-protected
Prevent pneumonia
Lifestyle
Smoking
Pollution
Susceptibility to infection
Smoking cessation
Avoidance
Immunizations
Preservation of lung function (all traits)
Normal Airway Development
This topic is reviewed in detail in chapter “Structural and Functional Changes in the Preterm Lung” of this book and elsewhere [40, 41]. For normal lifelong lung health, airway caliber must be normal at birth; spirometry improves to a plateau at age 20–25 years, and thereafter declines with age. These changes are best described by Global Lung Initiative (GLI) equations [42]. The threshold for respiratory disability will be crossed prematurely if there is impairment at birth, suboptimal growth in childhood, or accelerated decline in adult life. The developmental track of airway disease of prematurity is not clear. There are no large prospective cohort studies of preterm survivors extending from birth into middle age. Although a series of overlapping large birth cohort studies (term babies) have shown that spirometry tracks from the preschool years into the sixth decade [43–45], with no evidence of catch-up growth, this may not be the case in preterm airways disease [46, 47]. Indeed, in one cohort, there was evidence of airflow obstruction at age 7–9 years, which was no longer present at age 20–22 years [36]. However, these were relatively large babies by modern standards, and these data are not totally reassuring.
Pulmonary
As with any airway disease, deconstructing the components allows logical treatment; this is to be preferred to asking meaningless questions like “do BPD survivors have asthma?” – which is only interpretable if all concerned have a common definition of asthma. Survivors of prematurity generally have fixed and variable airflow obstruction, the latter of which is responsive to short-acting β-2 agonists [31, 46, 48, 49]. The severity of airflow obstruction varies between studies, with some, but by no means all, reporting significant airflow obstruction in adult BPD survivors [50]. The differences between studies likely reflect that different types of populations were studied. Generally, a greater extent and duration of neonatal intensive care predicts worse lung function long term, although there is considerable overlap between groups, and the relationship is not close. Although acute bronchodilator response to short-acting β-agonists, more peak flow variability and bronchial responsiveness to methacholine have all been reported, the pathophysiology of the variable airflow is unclear; despite fixed and variable airflow obstruction, the evidence is that these patients do not have airway inflammation; the underlying cause may be airway wall thickening and increased airway smooth muscle [51]. Alternatively, genetic factors predisposing to airway hyperresponsiveness may also contribute with other insults to the development of BPD. What is clear is that there is little evidence for ongoing airway inflammation in adult preterm survivors. Hence neither exhaled breath temperature nor exhaled nitric oxide (both indirect surrogates for airway inflammation) are elevated [52, 53], implying in this largely inhaled steroid naïve population that there is no eosinophilic, Type 2 inflammation. The logical corollary is that, unless there is a superposed component of atopic airways disease, inhaled corticosteroids should not be prescribed; and indeed, there was no benefit in one admittedly small trial [54]. One study using exhaled breath condensate showed increased oxidative stress(elevated exhaled breath condensate 8-isoprostane) in adolescent survivors, which, although the clinical significance is unclear, given there was no correlation with symptoms or spirometry [55]. A metabolomics approach also showed differences between BPD survivors and normals, also suggesting there may be ongoing airway abnormalities [56]. There are no studies on the airway microbiome or the cough reflex, and mucus hypersecretion is not generally a component of this airway disease. Finally, proximal airway disease should be considered, including tracheobronchomalacia, subglottic stenosis, and left vocal cord palsy secondary to surgery for patent arterial duct; this last is considered in more detail below.
Among the determinants of adult life lung function is birth weight [57]. In the wider population, low birth weight and low weight gain in the first 3 years of life were correlated with reductions in adulthood FEV1, lung volumes and transfer factor (DLCO), but not the presence of airflow obstruction [58]. Other studies have suggested an inverse relationship between weight gain in the first year of life and accrual of lung function [59–61]. Excessive weight gain after age 5 years may by contrast have later adverse effects [61]. However, there may be an increased risk of developing asthma if early weight gain is excessive [62]. In one cohort, birth weight in the SGA, but not AGA babies was an important determinant of spirometry age 20–22 years [36].This is important, because frequently this information is not reported. The study also did not demonstrate a greater prevalence of bronchial hyper-responsiveness to methacholine in adult survivors, which was reassuring; however this was not confirmed in a more recent study of a more severely affected population [63]. Another group [64] reported airflow obstruction in VLBW survivors (here defined as birth weight <1.5 kg) irrespective of any neonatal complications, worse those with BPD. There was an adverse an effect of maternal smoking in pregnancy, most marked in BPD survivors, but no effect of growth patterns at any developmental stage. In the longest follow up population based cohort [65] the effects of birth weight gradually declined with age, likely because environmental factors became increasingly important determinants.
It has been suggested that the survivors of premature birth will represent a new cohort of COPD patients. There are only very limited data on the very long-term outcome of BPD. Such data as exist do not suggest an accelerated rate of decline [63], at least in the early 20s, although factors such as the presence of bronchial hyper-responsiveness suggest this is a high-risk population for accelerated decline. A cohort of more than 6000 adults born between 1925 and 1949, reported in 2013, showed that women but not men born before 32 weeks gestation had a hazard ratio for any obstructive airway disease of 2.77 (95 % CI 1.39–1.54), with both low birth weight and prematurity being significant risk factors [66]. In a healthy population, lower birth weight was associated with a more rapid decline in FEV1/FVC ratio, but not in FEV1 or FVC; no gender effect was reported [67]. Whether bronchial hyper-responsiveness has the same adverse association with the attainment of poorer spirometry and greater decline in lung function in BPD survivors as in the general population is unclear [68–70]. Indeed, the basis of the hyper-responsiveness may well be different in the two groups (above). Low birth weight was associated with an increased risk of death from COPD [71]. The data would support the hypothesis that survivors are at risk from premature airflow obstruction, both by virtue of failing to reach the expected lung function plateau and also from having an accelerated rate of decline. In a population-based study, 26 % of those with an abnormal FEV1 at age 40 years went on to develop premature airflow obstruction [72]; whether this also applies to preterm survivors is not known, but the data do suggest a high risk in those born preterm if they fail to attain a normal lung function plateau. However, as with airways disease in childhood (above), it is important to deconstruct airways disease in the elderly; it should not be assumed that these patients have the same airway problems as lifelong smokers, nor should it be assumed that management is the same [73]. This is a fruitful area for future research, but until adult physicians are in the habit of taking a history of early life events [6, 7], research will likely be stalled.
Extrapulmonary
The range and treatment of comorbidities is beyond the scope of this chapter. There may be direct impact on the lung, for example, neurodevelopmental handicap leading to incoordinated swallow and aspiration, and impaired cough; and other organ manifestations, including visual impairment from retinopathy of prematurity, and renal and bone disease. Neurodevelopmental handicap may impact on the ability to use inhalers, and special attention needs to be paid to technique if these are prescribed. In one study, twice as many BPD patients reported difficulty with mobility and self-care compared with controls [31], although this did not reach statistical significance. Another group [32] reported that attention-deficit hyperactivity disorder (ADHD) was commoner in preterm survivors; however, another group suggested this was only the case for SGA survivors [74]. Cerebral palsy was commoner in adult BPD survivors, and they were less likely to access higher education or be in full-time employment; whether this relates to BPD itself, or associated low socioeconomic status, could not be determined. BPD survivors were more likely to use antipsychotic, sedative, and anxiolytic medication, although this is not a uniform finding [75]. Although it is difficult to unpick the pathways leading to these comorbidities, nevertheless, these data underscore that this is a needy group of young adults. Some of these young adults need access to holistic care; unfortunately, in most parts of even the developed world, this is not forthcoming, and transition from pediatric care, where there is a strong network of school and community-based services, is a nonevent because of total lack of services to which to transition.
Behavioral and Lifestyle Issues
Maternal smoking is an important risk factor for prematurity, and anecdotally, in our study [36], survivors of prematurity are more likely to smoke, although whether prematurity conveys an extra risk of taking up smoking, as against the risks if the parents smoke, was unclear. Whether these young adults are more likely to abuse other substances is not known. By contrast, in another study [75], survivors were no more likely to abuse alcohol than their peers, and the only criminal behavior that was more common in preterm survivors was fare-dodging. If possible, other forms of environmental pollution, in addition to tobacco smoke, should be avoided. Immunization especially against influenza is also important.
Parenchymal Disease
Alveolar Hypoplasia
“New BPD” in particular appears to be characterized by arrest of alveolar development. In animal models, hyperoxic gas mixtures, positive pressure ventilation, and systemic corticosteroids, all lead to alveolar simplification and failure of secondary septation. Previously, it was thought that the phase of maximal alveolar growth was within the first 2 years of life, the time of maximal exposure to iatrogenic toxicity. However, this may in fact not be the case (below). Hyperpolarized Helium (He3) data [76, 77], confirming animal work [78], suggest that in fact alveolar numbers continue to increase throughout the period of somatic growth. This is rather a double-edged finding; on the one hand, this gives more opportunity for catch-up growth, and on the other hand, the period of vulnerability is much greater.
Diffusing capacity of the lungs for carbon monoxide (DLCO) has been used as a surrogate for pulmonary capillary blood volume and can be measured reproducibly in preterm survivors [79]. Both the membrane and blood volume components of DLCO are reduced in preschool children born prematurely, suggestive of early alveolar hypoplasia [80]. Two studies suggest that there may be alveolar catch-up growth. He3 work in preterm survivors aged 10–14 years showed normal alveolar size [77]. An exercise study in 20–22-year-old survivors that used DLCO measured using CO18 rebreathing and respiratory mass spectrometry as a surrogate for alveolar capillary blood volume showed normal results at maximal exercise, implying the alveolar–capillary bed size was normal and normally distensible [81]. This study also highlights the difficulties in interpreting DLCO in the absence of a simultaneous measurement of pulmonary blood flow, since higher blood flows (as in exercise) lead to recruitment and distension of the pulmonary capillary bed and elevation of DLCO. Effective pulmonary blood flow (Qp.eff, which in this context is virtually equivalent to pulmonary blood flow) was also measured using C2H2. The results showed that there were differences in SGA and AGA survivors; the SGA survivors had reduced DLCO and Qp.eff at rest, normalizing on maximal exercise, which implies that the low resting DLCO was not due to lung or heart disease, but because cardiac output at rest had been set to a lower level, presumably as a result of intrauterine adverse circumstances. The AGA survivors were normal both at rest and on maximal exercise. These studies are reassuring, but in neither study were measurements made in early childhood, and neither studied the very small, extremely preterm babies; so, it would be wrong to be too reassured by these data. Others have reported lower DLCO at rest in preterm survivors [79, 82, 83], but without measurements of cardiac output, the significance of these findings is difficult to assess.
More recently, He3 data [84], backed up by animal work [85], suggests that maternal nicotine exposure reduces secondary septation, thus increasing alveolar size and reducing numbers. Taken together, it is likely that there is early onset of an emphysema-like disease as a result of prematurity and not merely premature airflow obstruction.
Focal Parenchymal Abnormalities
Imaging abnormalities usually reflect the consequences of prematurity and its treatment, but just occasionally, an interstitial lung disease may present in a preterm baby, and will not be suspected unless appropriate imaging is carried out. The chest radiograph may be obviously abnormal (Fig. 1). High-resolution computerized tomography (HRCT) may reveal extensive abnormalities that were not immediately obvious on the chest radiograph. However, there is no current indication for routine HRCT in preterm survivors [86]. The classical HRCT appearances of “old BPD” are well-defined linear densities, hypoattenuated areas, and subpleural triangular densities, suggestive of patchy atelectasis and hyperinflation [87] (Fig. 2). The largest imaging series [88] showed confirmed these findings, but perhaps surprisingly, BPD was not associated with worst change over and above prematurity itself, underscoring that there is only a loose association between neonatal events and long-term outcomes. There was a relationship however with duration of oxygen therapy [89]. Subsequent studies have frequently shown often quite extensive areas of focal lung destruction in BPD survivors, even if lung function is not particularly abnormal [89–91]. Studies in “new BPD” are more limited [92, 93]. Perhaps surprisingly, the changes are quite similar to “old BPD,” at least in the relatively younger populations studied. Thus, although the relatively mild lung function abnormalities are reassuring to some degree, there may still be quite marked structural changes. It is likely that advances in MRI will allow this modality to be used more, elucidating both structure and function [94].



Fig. 1
Chest radiograph of an ex 24-week gestation baby with bronchopulmonary dysplasia, now aged 10 years. There are low flat diaphragms and very featureless lung fields, reminiscent of adult emphysema

Fig. 2
(a) Chest radiograph of a 3-month old, ex-preterm baby with “old” BPD, still oxygen-dependent. There appears to be only relatively mild areas of hyperinflation with some opacification. (b) The CT scan of the same child shows very severe changes, classical of “old BPD” with coarse fibrotic strands and atelectasis, alternating with severe hyperinflation. The child subsequently dies
Impaired Exercise Performance
In general, exercise performance in daily life in preterm survivors is good in adolescence and adult life, and the results of exercise testing, although not normal, often only reveal subtle abnormalities [81, 82, 95, 96], with no difference in longitudinal changes when these have been reported. Generally, exercise hypoxemia is not reported in these survivors [83]. Overall, neonatal events do not predict exercise performance [97]. Adults born preterm may exercise less than term born controls [98], and leisure time exercise rather than airflow obstruction or degree of prematurity correlates with formal exercise performance, and perhaps this is an area in which some form of pulmonary rehabilitation may be helpful. There is a differential hemodynamic response to exercise in SGA and AGA children, as discussed above, underscoring the need to try to separate out these two groups in future studies.
Notwithstanding, exercise testing does unmask physiological differences between preterm survivors and controls. Narang et al. [81] found that a minor elevation in FRC at end-exercise was the only difference between survivors of prematurity and controls, suggestive that there may have been some dynamic hyperinflation, despite no differences in resting spirometry. In a much more premature group of survivors than that studied by Narang et al., Lovering et al. [99] reported that, compared with normal controls, inspiratory reserve volume reduced at lower workloads in preterm survivors, who also developed severe dyspnea and leg pain on exercise, this despite having only relatively minor airflow obstruction at rest. The BPD survivors had more expiratory flow limitation at an early stage of exercise than the other groups. They suggested that there was a mechanical constraint to raising Vt during exercise (by contrast with Narang et al. [81]). There were no measurements of muscle blood flow or muscle oxygen extraction, which might have shed light on the mechanism of leg pain. It is surely conceivable that if auto-PEEP increases on exercise as pleural pressures rise, then venous return and hence cardiac output might drop, although again this was not found by Narang et al. [81]. The dyspnea was attributed to loss of inspiratory reserve capacity [100].
Exercise performance may also be reduced by upper airway obstruction. The left recurrent laryngeal nerve closely abuts the arterial duct on its course into and out of the thorax to innervate the left vocal cord. In one study, adults who were born preterm (defined as birth weight ≤1000 gm or gestational age ≤28/40) were studied using transnasal flexible laryngoscopy (at rest and on exercise) [101]. Thirteen had undergone cardiac surgery for a patent arterial duct, a common complication of prematurity, of whom seven had a left vocal cord palsy. Exercise-induced noisy breathing had previously been put down to prematurity or asthma, but unsurprisingly there had been no response to treatment. Increasing exercise symptoms correlated with increasing aryepiglottic collapse at endoscopy.
In summary, levels of activity are reduced in preterm survivors, and this is associated with what are usually mild impairments of exercise performance. The data suggest these subjects may benefit from fitness training, although this needs to be tested in future studies.
Abnormal Control of Breathing
There are extensive studies on the multiple mechanisms whereby control of breathing is disordered in infancy after premature birth [102]. There is very little information relating to adolescents and adulthood. Sleep-disordered breathing is more common in mid-childhood after prematurity [103], but whether BPD confers an extra risk is unclear [104, 105]. A very small study documented abnormal responses to hypoxia and hyperoxia in adult preterm survivors [106]. This is an area for future research, and certainly there should be a low threshold for performing polysomnography in adult preterm survivors. Whether there is any deficit in control of breathing during exercise is unclear [102].
Pulmonary Circulatory Disease
Pulmonary hypertension, which is multifactorial, and increased pulmonary vasoreactivity are well described in preterm infants. It is said that the elevation in pulmonary vascular resistance disappears by childhood [107]. However, pulmonary vascular reactivity to hypoxemia and inhaled nitric oxide may persist into adolescence [108]. There are only scanty data on pulmonary circulation in adult survivors. The most intriguing data are from a comparison of 10 survivors of persistence of the fetal circulation (PFC) compared with 10 matched controls at age 21 years [109]. The investigators performed echocardiographic estimates of pulmonary arterial pressure at sea level and altitude. There was no difference in change in mean arterial oxygen saturation, but the PFC survivors had a much greater pulmonary hypertensive response (mean 62.3 vs.49.7 mm Hg). Another group showed a greater pulmonary hypertensive response to hypoxemia in preterm survivors [110]; this has implications for air travel, given that flying in a commercial jet is equivalent to breathing 15 % oxygen [111]. The systemic cardiovascular consequences of prematurity are out of the scope of this chapter, but have been reviewed elsewhere [112].
In summary, the possibility that an adult survivor of prematurity may have reduced pulmonary vascular reserve, and have or develop frank pulmonary arterial hypertension during aging, should be borne in mind. It is clearly important to ensure that such patients do not develop significant hypoxemia due, for example, to sleep-disordered breathing (above).
Developmental Origins of Health and Disease Hypothesis (DOHAD)
This hypothesis states that in utero events at critical times can reprogram an individual to respond better to current adverse circumstances, but at a cost of later maladaptive responses. Speculatively, this may account for the pulmonary hypertensive responses in preterm survivors described earlier. Unsurprisingly, the best data come from murine models; tobacco smoke exposure of pregnant dams leads to enhanced allergic responses postnatally in the offspring [113], and neonatal hyperoxia alters pulmonary immune responses and oxidative stress [114, 115]. It is possible, but speculative, that the increased oxidative stress in human preterm survivors (above) may be a DOHAD manifestation. Clearly, more research is needed, but the clinician should be alert to the possibility that these survivors may react differently to adverse later life insults.
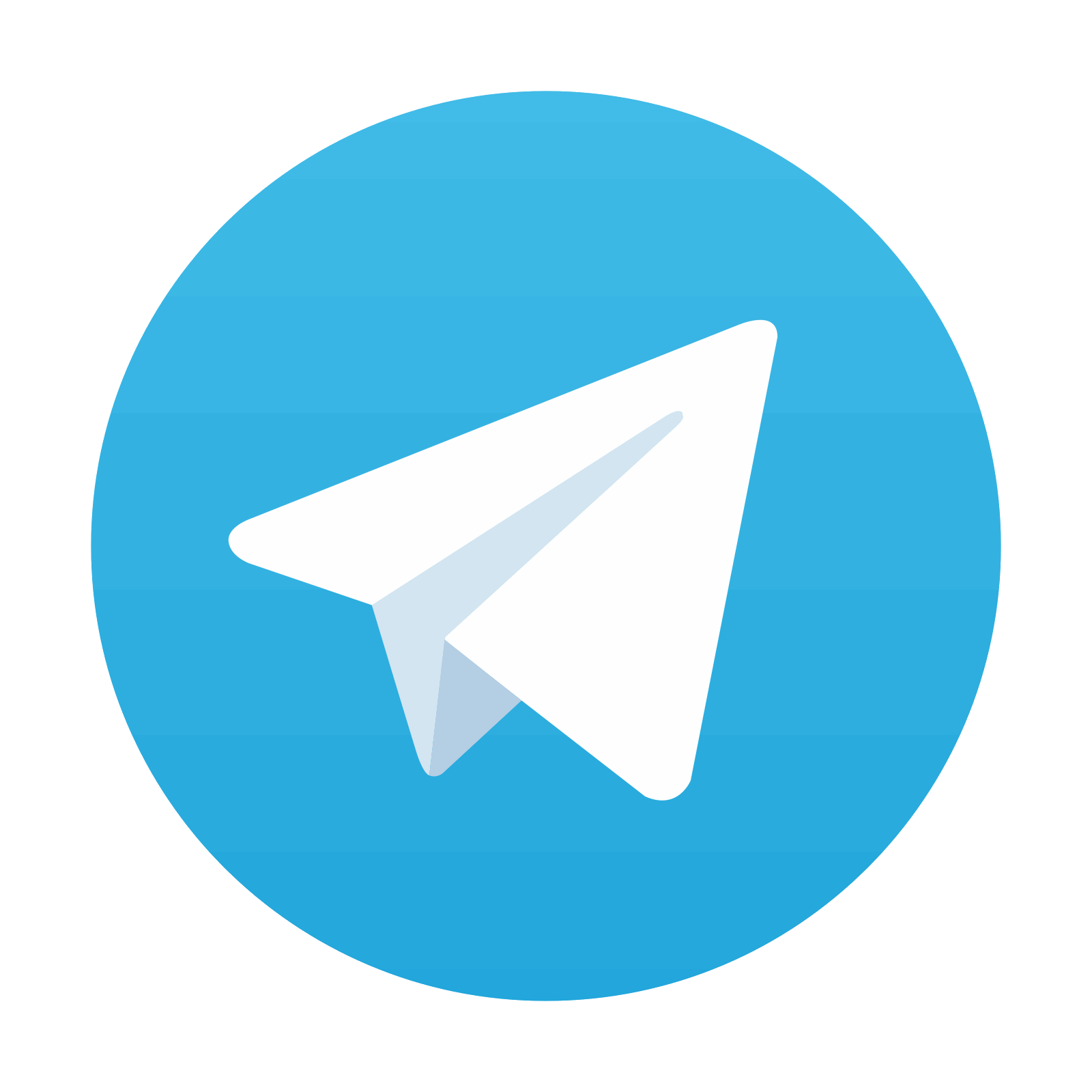
Stay updated, free articles. Join our Telegram channel

Full access? Get Clinical Tree
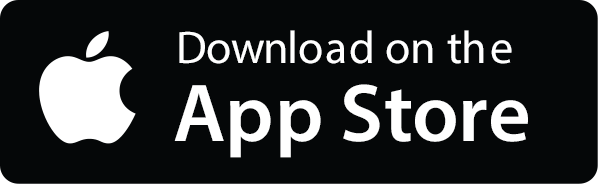
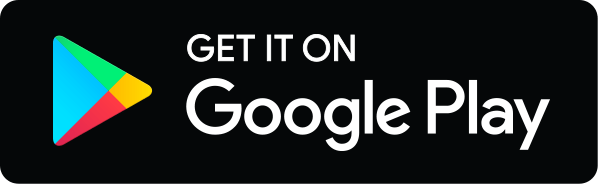