and Susan Redline2
(1)
Case Western Reserve University School of Medicine, Rainbow Babies and Children’s Hospital, Cleveland, OH, USA
(2)
Harvard Medical School, Brigham and Women’s Hospital and Beth Israel Deaconess Medical Center, Boston, MA, USA
Keywords
PrematurityBronchopulmonary dysplasiaSleep-disordered breathingObstructive sleep apneaCircadian rhythmInfancyChildhoodAdolescenceAdulthoodAbbreviations
AHI
Apnea hypopnea index
AI
Apnea index
AS
Active sleep
BMI
Body mass index
BPD
Bronchopulmonary dysplasia
NREM
Nonrapid eye movement
OSAS
Obstructive sleep apnea syndrome
PSG
Polysomnogram
QS
Quiet sleep
RDI
Respiratory disturbance index
REM
Rapid eye movement
SDB
Sleep-disordered breathing
SIDS
Sudden infant death syndrome
UARS
Upper airway resistance syndrome
VLBW
Very low birth weight
Emergence and consolidation of sleep states and maturation of control of breathing during sleep and wake are critical developmental milestones in the first year of life. Additional maturation occurs throughout childhood, and early insults may impact sleep and breathing into childhood, adolescence, and adulthood. The developmental trajectories of sleep state maturation and control of breathing are impacted by premature birth across the spectrum from marked prematurity with infants born with very low birth weight (VLBW, <1500 g) to those born at 34–37 weeks gestation (late preterm and early term). In this chapter, we will review the association of premature birth with sleep outcomes once infants reach full term, through childhood, and into early adulthood. Our focus will be on the putative impact of premature birth on disordered breathing during sleep and sleep behaviors and circadian rhythm development, organized by age group. We will also highlight opportunities for further research to address important knowledge gaps.
Overview of Respiratory Control and Sleep State Maturation in Infancy
While a detailed review of normal maturation of breathing and sleep and the impact of prematurity on these processes during the period immediately after birth is outside the scope of this chapter and has been reviewed elsewhere [1–5], we will briefly review important concepts. The maturation of respiratory control, sleep state, and arousal is governed by complex interactions between the central and peripheral nervous systems during prenatal and immediate postnatal development, with voluntary and involuntary components. Maturation of neural control of breathing, including peripheral chemoreceptors, central chemoreceptors, and centers in the midbrain and forebrain, is a nonlinear developmental process that spans from prenatal to postnatal periods. In addition to central nervous system development, chest wall compliance, mechanoreceptors or stretch receptors, airway compliance, laryngeal reflexes, and responsiveness of chemoreceptors to hypoxia, hypercapnia, and changes in pH all undergo maturational changes in the postnatal period in healthy infants. In the immediate postnatal period, prematurity-related interruptions or delays in these developmental processes can lead to apnea of prematurity, periodic breathing, and difficulties coordinating sucking, swallowing, and breathing.
The maturation of control of breathing coincides with the emergence of mature sleep states. While ventilation generally decreases during sleep, there are significant variations in breathing patterns and respiratory chemosensitivity across sleep states, and these are evident throughout the life span. Sleep in children and adults can be divided into two primary states: nonrapid eye movement sleep (NREM) and rapid eye movement (REM) sleep. By about 32 weeks gestation, fetuses and neonates exhibit immature versions of these states, namely quiet sleep (QS) and active sleep (AS) [6]. QS and AS mature into NREM and REM sleep, respectively, during the first several months of life in term infants. The proportion of time humans spend in each sleep state changes dramatically during prenatal and postnatal development, with AS dominating in the prenatal period, with a transition to NREM dominance after birth. This maturational change is thought to be important for brain maturation and plasticity. Term infants spend approximately 50 % of sleep time in AS [7], and as AS matures into REM sleep, the proportion decreases to approximately 25 % as infants approach 1 year of age [8]. Maturation of sleep architecture during the first year of life does not appear to be significantly delayed in children who were born preterm [9, 10]. NREM sleep is characterized by a lack of behavioral controls, with more regular breathing and reduced tidal volume and respiratory rate compared with wakefulness. During REM sleep, respiratory rate and tidal volume become more irregular. REM sleep is also characterized by a reduction in muscle tone; short central respiratory pauses are common during REM sleep in healthy children. The loss of muscle tone and marked respiratory variability interact with the immature features of the infant respiratory and nervous systems to result in increased risk for apneas and periodic breathing in premature infants in the immediate postnatal period. Specifically, reduced neuromuscular input to respiratory muscles along with the increased chest wall compliance seen in infants leads to a reduction in ventilation during sleep. Increased laryngeal reflexes and increased sensitivity of central and peripheral reflexes may then precipitate apneas.
In summary, differences between adult and newborn respiratory systems that make infants more vulnerable to disordered breathing during sleep include central nervous system immaturity, exaggerated laryngeal reflexes, increased chest wall compliance, immaturity of chemoreceptors, and increased time in active sleep/REM sleep (summarized in Table 1). Premature birth, with interruption and delay of neurological and pulmonary maturation, along with increased susceptibility to pulmonary disease and exposure to adverse effects of treatment, exaggerates these influences.
Table 1
Characteristics of the premature respiratory system that contribute to disordered breathing in infancy
Interrupted development of alveolar units, surfactant system, and pulmonary vasculature |
Increased inflammation and oxidative stress (infection, treatment-related) |
Immature chemoreceptors (enhanced peripheral sensitivity and reduced central sensitivity) |
Increased laryngeal chemoreflexes with apnea, bradycardia, and vasoconstriction |
Increased time in active sleep with loss of expiratory braking and stabilizing intercostal muscle tone |
Increased chest wall compliance, lower specific lung compliance |
Mechanical disadvantage due to barrel-shaped chest and more horizontal rib position |
Disordered Breathing During Sleep Across the Life Span (Fig. 1)
Preterm Infants at Term Postconceptual Age
While control of breathing and sleep state maturation becomes more normal as preterm infants approach term postconceptual ages, there is some evidence for residual effects of prematurity. Two early cross-sectional studies in late preterm infants demonstrated minor increases in the number of short (2–15 s) central pauses in former late preterm infants [11], and shorter but similar numbers of pauses in preterm compared to term infants [12]. Neither study reported an increase in the number of prolonged apneas or apneas that resulted in significant cardiorespiratory consequences. Both of these studies assessed breathing during sleep only at a single time point.


Fig. 1
Summary of associations between premature birth and sleep-disordered breathing during sleep throughout the life span
Albani et al. prospectively studied a small group (n = 12, 50 % male) of late preterm (mean gestational age 35 weeks, range 32.2–36.6) infants and term (n = 21, 48 % male) infants with sequential polysomnograms (PSGs) at 40, 52, and 64 postconceptual weeks to characterize the number, duration, and character (obstructive, central, mixed) of apneas [13]. All infants in both groups were apparently healthy, and did not have evidence of apnea of prematurity requiring pharmacological treatment, bronchopulmomary dysplasia or other pulmonary disease, or intracranial hemorrhage. Apnea was defined as a pause of 3 s or greater, and classified as central if no respiratory effort was recorded, obstructive if respiratory effort was recorded, and mixed if both patterns were present in either order. Apnea density (number per unit time), duration, character, and the presence of periodic breathing were measured. Compared to term infants, preterm infants had significantly increased density of apneas at the 40-week recording (126 vs. 49 per 100 min recording, p < 0.05). Most of the observed differences were attributable to a higher density of obstructive/mixed apneas in NREM/quiet sleep among the preterm infants. At the 52-week postconceptual age recording, term infants had higher apnea density overall (50 vs. 20 per 100 min, p < 0.05), driven largely by findings in REM sleep, and there were no differences at 64 weeks postconceptual age in apnea density or type. Apneas in both groups were very rare during the final recording. The majority of preterm infants demonstrated periodic breathing at 40 weeks (75 % vs. 42 % term, p < 0.05), primarily during NREM sleep. Periodic breathing was rare in both groups during subsequent recordings. Somewhat surprisingly, two of the preterm infants died during the study; both deaths were attributed to sudden infant death syndrome (SIDS), one at 44 weeks after one PSG and one at 56 weeks after two PSGs. The infant who died at 44 weeks was described as having more frequent and longer apneas (obstructive and mixed) and more periodic breathing than the other preterm and term infants. The second infant was similar to preterm peers. This study, although small, suggests that variations in breathing during sleep in preterm infants in the immediate postnatal period largely resolve shortly after postconceptual term ages are reached in healthy late preterm infants. These findings cannot be extrapolated to children born extremely prematurely or with significant lung disease, and are limited by the very small sample size. Research studies also have shown that the presence and severity of bronchopulmonary dysplasia (BPD) may be associated with the severity of alterations in control of ventilation [14, 15]; therefore, BPD may influence propensity for persistent sleep-related breathing disturbances in preterm infants.
First Year of Life and Sudden Infant Death Syndrome
SIDS is defined as the unexplained death of a child during sleep before 1 year of age. While the precise pathophysiology and etiology of SIDS remain elusive, and is likely multifactorial, it is thought to be a disorder of respiratory control and arousal. Infants born prematurely are at increased risk for SIDS. Early descriptions of the relationship between preterm birth and SIDS suggested that rates rise substantially with increasing prematurity (1.06 deaths/1000 live births at term vs. 3.52 deaths/1000 live births at 24–28 weeks gestation) and that the peak age at death was 4–6 weeks earlier in preterm children [16]. Despite a decrease in overall rates of SIDS with the “Back to Sleep” campaign, preterm birth remains a risk factor for SIDS. In one population-based case-control study in five regions in England, preterm birth (<37 weeks gestation) was associated with a 3.44 (2.47–4.79) increased odds of SIDS, although this relationship was attenuated and no longer significant after multivariate adjustment [17]. Other studies, however, have found higher rates of SIDS in children born preterm in multivariate analyses that include adjustment for maternal smoking, markers of socioeconomic status, and race, including a study of linked US birth and death records from 1996 to 1998 [18]. In this study, adjusted odds ratios for SIDS in children born preterm compared with those born at 40–41 weeks gestation ranged from 1.52 (1.42–1.63) for children born at 36–37 weeks gestation to 2.88 (2.57–3.23) for children born at 28–32 weeks gestation. Halloran and colleagues also found that the postnatal age at death was slightly later in preterm infants than term infants (16 vs. 12 weeks), with a second peak at approximately 28 weeks in those born extremely prematurely (22–27 weeks gestation), although postconceptional age at death was earlier in preterm infants [18]. Similar to the findings by Malloy and Hoffman [16], when analyzed by postconceptional age, very preterm infants died, on average, more than 6 weeks earlier than term infants (46 vs. 54 weeks postconceptual age). The increased prevalence of SIDS in children who were born prematurely suggests that there are sustained abnormalities in respiratory control in some cases, although non respiratory (cardiac) differences may also play a role. Instability of central chemoreception, responses to carbon dioxide and hypoxia, and the impact of early chronic intermittent hypoxemia on the autonomic nervous system have all been implicated (reviewed in [4, 19]). Shared risk factors for prematurity and SIDS (maternal inflammation, nicotine exposure) have also been suggested to explain the association [19].
Sleep-Disordered Breathing: Preschool to Adolescence
SDB is a term that broadly describes abnormal breathing and/or gas exchange during sleep, and encompasses a spectrum of severity from primary snoring to upper airway resistance syndrome (UARS) to obstructive sleep apnea syndrome (OSAS). Untreated SDB is associated with behavioral and neurocognitive effects across its spectrum [20], and metabolic and cardiovascular effects in its more severe form [21]. The prevalence of snoring, as reported by caregivers, is highly variable depending on the definition used, but a meta-analysis of studies that included over 95,000 children (0–19 years of age) worldwide found the prevalence to be 7.45 % (95 % CI 5.75–9.61 %) [22]. Most studies suggest an OSAS prevalence of 4–11 % using questionnaires and 1–4 % using PSG in otherwise healthy children [22], with a peak prevalence occurring between ages 2 and 6 years. A second increase in prevalence is likely in adolescence, associated with obesity. Many studies have demonstrated prematurity to be a risk factor for obstructive sleep-disordered breathing during childhood [23–25]. However, these associations vary by age group. A longitudinal birth cohort showed that the association between SDB and prematurity was present in early childhood, but not during adolescence, when obesity emerged as a strong risk factor [26].
The pathophysiology of OSAS is complex, and reviewed in detail elsewhere [27]. While the defining feature is the partial or complete collapse of the upper airway during sleep, there are multiple factors that likely contribute to upper airway obstruction, including soft-tissue hypertrophy (adenoids, tonsils, airway fat deposition), skeletal craniofacial features, pharyngeal and laryngeal tone, lower respiratory tract neuromuscular tone, arousal threshold, and control of breathing [27, 28]. Gas exchange abnormalities due to lower respiratory tract disease, upper airway inflammation due to gastroesophageal reflux, or swallowing disorders may influence the development or presentation of OSAS. Premature birth may influence several of these factors, most notably ventilatory control, airway tone, craniofacial growth, lower airway disease, and increased risk for obesity in some premature populations. As these influences seem to change with age, we will discuss the relationship between prematurity and SDB by age category. When possible, differences in children with and without BPD will be discussed.
Sleep-Disordered Breathing in Preschool Children
While prematurity has been fairly consistently identified as a risk factor for SDB in older children (discussed later in the chapter), there are not enough data to reach a reliable conclusion about the role of prematurity (with or without BPD) in children prior to school age. Rates of symptoms of sleep-disordered breathing reported in cohorts of former premature infants in this age category range from approximately 8 % [29] to 20 % [30], and are not significantly different from rates in population-based studies of healthy toddlers [22]. In a study of preterm children at 9 months corrected age (mean gestational age of 31.6 weeks), birth weight and gestational age were not identified as risk factors for the presence of SDB symptoms [29]. In a study of slightly older toddlers (18–22 months) who had been born extremely prematurely (24–28 weeks gestation), no markers of severity of neonatal illness in the neonatal period were associated with SDB risk [30]. Surprisingly, several markers of neonatal illness severity were associated with a lower risk of reported SDB symptoms, including duration of caffeine treatment, duration of ventilator treatment, and hospital length of stay. The presence of BPD, necrotizing enterocolitis, and exposure to both hypoxia and hyperoxia were not associated with SDB symptoms in either direction. Female sex was the only risk factor identified, with females 2.7 times (95 % CI 1.13–6.5) more likely to have SDB symptoms [30]. These studies, however, are limited by the lack of accuracy of symptoms to identify SDB and potential parental reporting biases.
There have been two large population-based studies that have examined the relationship between prematurity and symptoms of SDB, with disparate findings. The first is a longitudinal study that enrolled over 14,000 children in Southwest England (Avon Longitudinal Study of Parents and Children) [31]. SDB was assessed using questionnaires administered at three time points, 1.5, 4.75, and 6 years of age. In this study, prematurity was not a risk factor for SDB symptoms (snoring, mouth-breathing, witnessed apneas) at ages 1.5 or 4.75 years. There was a modest relationship between gestational age and witnessed apneas only at age 6 (OR 1.23, 95 % CI 1.02, 1.47).
In a cross-sectional study using data from the medical records of nearly 400,000 Australian children ages 2.5–6, sleep apnea, as defined by the use of billing codes, was present in 4145 or 1 % of children [32]. Children born prematurely (gestational age <32 weeks) were more likely to have a diagnosis of sleep apnea (hazard ratio 2.74 (95 % CI 2.16, 3.49) in multivariate models. Children born small for gestational age did not have increased risk for sleep apnea.
The above studies, although quite large, used very different methodologies to classify children as having SDB, both of which may result in misclassification. The Avon Longitudinal Study of Parents and Children study [31] identified children with SDB symptoms via questionnaire, and based on other studies, less than half of these children would likely have PSG-documented OSAS. Conversely, the Australian study [32] identified sleep apnea using diagnostic codes, which requires a health care provider to elicit symptoms and often conduct follow-up testing, and then enter the code, likely underestimating the prevalence. This supposition is supported by the difference in the prevalence reported (1 %) and that reported in a meta-analysis of the prevalence of SDB symptoms such as snoring of around 8 % [22].
The studies discussed above used questionnaires or administrative data to assess for SDB symptoms. In-lab polysomnography (PSG) is expensive and may be burdensome to families, likely explaining the lack of published reports of cohorts of premature infants studied prospectively with PSG. We identified two retrospective studies that examined PSG findings in early childhood in former preterm infants. In a retrospective study of 62 children with BPD < 3 years of age (mean 10 months, range 2.5–31.4 months, mean gestational age of 25.8 ± 1.9 weeks) undergoing PSG for clinical indications including oxygen titration, McGrath-Morrow [33] reported that the mean respiratory disturbance index (RDI, sum of obstructive apneas, mixed apneas, central apneas, and hypopneas/hour of total sleep time) was 8.2 ± 10.1. Obstructive apneas were rare (average index of 0.7 ± 1.6). Similar to patterns seen in healthy children, central apneas made up the largest proportion of reported events (mean 3.6 + 4.6), followed by hypopneas (2.8 ± 6.1). Children who were 18 months or older when studied had a lower RDI (3.4 ± 2.9) than the entire cohort. A subset underwent a second PSG at a mean age of 13.4 + 5.2 months; RDI improved from 14.5 ± 13.3 to 5.2 ± 6.3. The authors did not report the number of children followed at the center who did not undergo PSG; so, prevalence estimates are not possible. There was no control population reported, but values are above published normal values, at least in the younger age groups. These findings suggest that former preterm infants with BPD may be more likely to have abnormal PSG findings, but the study design does not allow prevalence estimates nor does it distinguish between findings related strictly to lower respiratory tract disease versus upper airway obstruction.
Sharma and colleagues used a retrospective study design to study children with BPD followed at a single pulmonary center who had been diagnosed with OSAS [34]. Of 387 children with BPD, 12 (3 %) had PSG findings suggesting OSAS. Children with OSAS were characterized by a mean gestational age of 27 weeks (range 24–33); 10 out of 12 had been intubated in the neonatal period, and 9 out of 12 met criteria for BPD. The mean age at the time of the study was 19 months (range 9–28), and most presented with snoring. The mean apnea hypopnea index (AHI) was 29 (range 1–120). The degree of prematurity did not correlate with OSAS severity. All were treated with adenotonsillectomy. Three required additional treatment (positive pressure ventilation or supplemental oxygen), although most did not have follow-up PSGs. The PSGs were ordered based on clinical symptoms, and the authors do not report the number of children who were studied with PSG overall or the number with negative studies; so, it is not possible to estimate the prevalence of SDB in this sample of children.
Prematurity has been identified as a risk factor for PSG-defined OSAS in two retrospective studies of young children. In a single center retrospective study of 139 children ages 0–17 months with PSG-confirmed OSAS, prematurity was identified in 29 % of children [35]. More than half of the premature infants in this study had severe OSAS (AHI >10). Gestational age, the presence of BPD, or other comorbidities were not reported. In another single site retrospective study of children under 2 years of age who underwent adenotonsillectomy at a single institution over a 7-year period, the authors identified prematurity as a risk factor for PSG-defined OSAS as well as SDB as defined by symptoms prompting surgical treatment [36]. Prematurity (gestational age < 37 weeks) was present in 27 % of children with PSG-defined OSAS compared with 9 % of the statewide population of children under 2 years of age (p < 0.0001). In this study, prematurity was not associated with severe OSAS (AHI >10) in multivariate analyses, but this may have been limited by little statistical power.
In summary, in very young children, a number of studies have reported that prematurity is associated with SDB symptoms and/or PSG-identified OSAS. However, existing data are not fully consistent, with gaps in understanding whether SDB severity is associated with prematurity. There is also uncertainty over whether this relationship is explained by BPD.
Middle Childhood and Obstructive Sleep Apnea Syndrome
The published data suggesting prematurity is associated with an increased risk for sleep disordered breathing is more consistent in school-aged children than it is in younger children. The Cleveland Children’s Sleep and Health Study (CCSHS) is a population-based study of 907 children aged 8–11 years of age that used stratified sampling to achieve over-representation of former preterm children (gestational age < 36 weeks) [24]. Children were studied in their homes with multichannel recordings of airflow, respiratory effort, electrocardiography, and oximetry. The primary definition used to define OSAS was an obstructive AHI ≥5. Snoring was more common in children born preterm (21 % vs. 14 %, p = .0049), as was OSAS (4.3 vs. 0.9 %, p = .0013). OSAS was five times (95 % CI 1.6–20.1) more likely in children born prematurely than those born at term in analyses adjusted for race, obesity, and sex. OSAS was also independently associated with African American race.
In a follow-up analysis that focused on the 383 children born preterm in the Cleveland Children’s Sleep and Health Study cohort, researchers aimed to identify demographic and perinatal risk factors for SDB (defined as obstructive apnea index (AI) ≥1 or obstructive AHI ≥5) at school age [23] within this subgroup. Univariate analysis identified race, having a single mother, mild preeclampsia, cardiopulmonary resuscitation or intubation in the delivery room, and use of methylxanthines as risk factors. After adjusting for race, having a single mother was associated with a 2.45 (95 % CI 1.01–6.39) increase in odds of SDB, and maternal mild preeclampsia was associated with a 7.56 (1.66–34.48) increase. Severe preeclampsia was not associated in either unadjusted or adjusted analyses. The investigators speculated that differences in the putative pathophysiology of mild and severe preeclampsia could explain these findings, with mild preeclampsia reflecting influences of placental hypoxemia occurring independently of vascular and other changes characteristic of severe preeclampsia. Maternal smoking may have been a particularly important confounder, as maternal smoking may reduce the risk of severe preeclampsia, and smoking rates were much higher in those with mild preeclampsia. While the point estimates of the association between maternal smoking and SDB were elevated (2.1), this was a nonsignificant finding. There was no univariate or multivariate association between multiple markers of neonatal illness severity in this study, including gestational age, birth weight, BPD, duration of ventilatory support, and intraventricular hemorrhage.
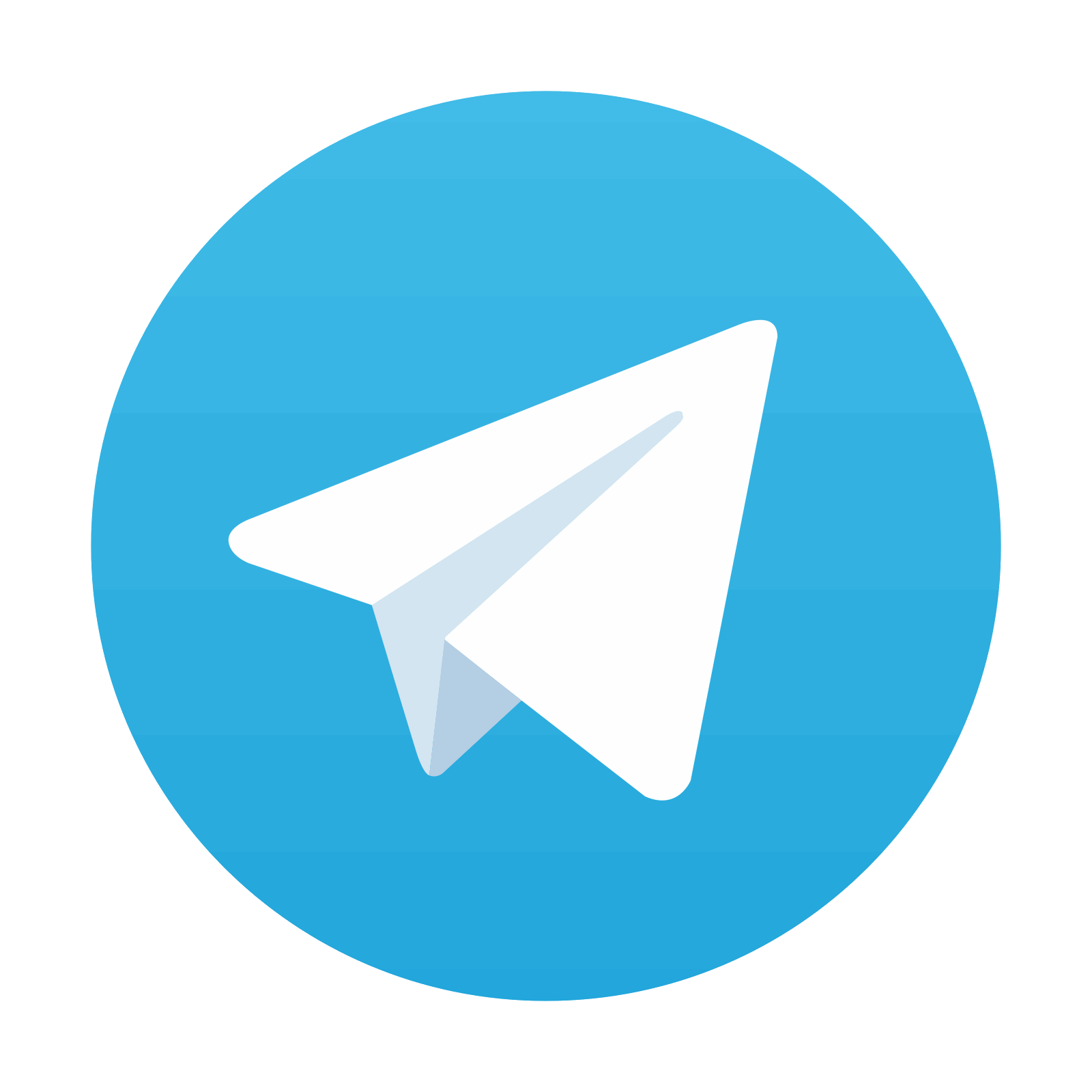
Stay updated, free articles. Join our Telegram channel

Full access? Get Clinical Tree
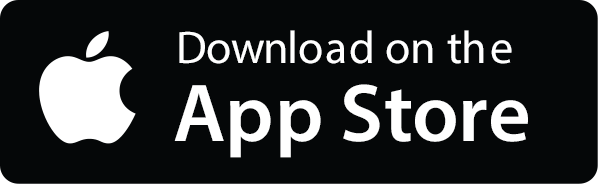
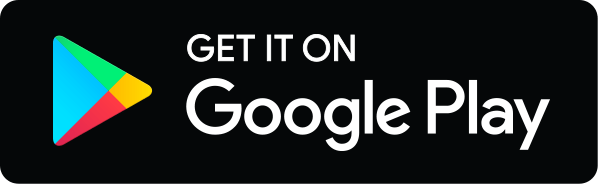
