Chapter 23 Surgical Critical Care
Central Nervous System
Neurologic Dysfunction
The central nervous system (CNS) is the most complex organ system in the body and is vulnerable to disturbances by a host of conditions and factors. The causes of an altered consciousness are so broad that a clouded sensorium is the norm in the ICU rather than the exception. This list of conscious-altering causes includes endogenous and exogenous factors. Some endogenous causes include sepsis, CNS infections, hypoxic-ischemic encephalopathy, tumors, trauma, electrolyte derangements, and metabolic conditions, whereas exogenous causes can include medications, environmental settings, and toxins. Any unexplained changes in a surgical patient’s level of consciousness or sensorium should be worked up thoroughly. Ascribing such changes to ICU psychosis should be considered strictly a diagnosis of exclusion.1 The term altered mental status is nonspecific and more descriptive definitions were offered by Plum and Posner almost 30 years ago in their text on stupor and coma and still apply today. The term confusion encompasses bewilderment, difficulty following commands, disturbed memory, and drowsiness or nighttime agitation, whereas delirium is a floridly abnormal mental state characterized by disorientation, fear, irritability, misperception of sensory stimuli and, often, visual hallucinations.
The presence of delirium can have far-reaching consequences and is associated with increased hospital length of stay and mortality.2 Obtundation is defined as mental blunting associated with slowed psychological responses to stimulation. Stupor is described as a condition of deep sleep or behaviorally similar unresponsiveness in which the patient can be aroused only by vigorous and repeated stimuli. Coma is a state of unarousable psychological unresponsiveness in which the subject lies with eyes closed and shows no psychologically understandable response to external stimuli or inner need. A vegetative state is a state of wakefulness but with apparent complete lack of cognitive function. Death in the presence of cardiopulmonary function (brain death) refers to the absence of function of the brain and brainstem; there are specific criteria for this, but the absence of cerebral function is paramount. Cortical nonfunction must be accompanied by the loss of reflexes to pupillary light and corneal stimulation, loss of the vestibulo-ocular and oropharyngeal reflexes, and apnea in the presence of adequate stimulation (PaCO2 > 60 mm Hg for 30 seconds). Generally, two clinical examinations must be documented, separated by a defined time interval (e.g., 6 hours) and confirmed by independent physicians; however, there is no nationally accepted definition of brain death. It is important that there be a reason or physiologic insult sufficient to cause death and there are no complicating or reversible conditions (e.g., recent administration of sedative or anesthetic agents, hypothermia, hypo- or hyperglycemia, or severe hypo- or hypernatremia, or other significant metabolic derangements) that could interfere with brain death determination. If such complicating conditions preclude the completion of a clinical examination, additional tests are required, including provocative tests (e.g., apnea test). Electroencephalography, radioisotope brain scanning, transcranial Doppler ultrasonography, and cerebral arteriography with documentation of absent cortical flow are all helpful in defining brain death but this diagnosis must conform to the criteria set forth by each state government and the hospitals contained within.
Although it is important for staff physicians and surgical house personnel to become comfortable and proficient with the neurologic examination of critically ill and ventilated patients, it is tremendously beneficial when nurses provide frequent objective evaluations and can relay any significant changes to the surgical team, especially regarding states of delirium or confusion. ICU nurses generally care for limited numbers of patients simultaneously and thus are able to perform these evaluations on a frequent basis. The confusion assessment method for identifying ICU delirium (CAM-ICU) is a rapid, objective measuring tool to determine the presence of delirium and can be performed serially with relative ease.2 CAM-ICU is straightforward and addresses whether there is an acute or fluctuating onset of a patient’s mental status change or delirium, general inattention, disorganized thought, or altered level of consciousness. The mean time required for each CAM-ICU assessment is less than 1 minute and can be performed by any trained health care provider (e.g., physician, nurse, respiratory therapist); its reliability and accuracy has been validated in prospective cohort studies weighed against the standard of evaluations of delirium experts using criteria from the Diagnostic and Statistical Manual of Mental Disorders. The importance of a tool such as CAM-ICU lies in its ability to identify delirium quickly, accurately, and easily so that appropriate drug therapy may be instituted.
Analgesia, Sedation, and Neuromuscular Blockade
Pain Assessment and Management
Perception of pain is influenced by prior experiences, negative expectations, and the cognitive capacity of the patient. The patient and family should be advised of the potential for pain and strategies to communicate pain. Patient self-reporting is the gold standard for the assessment of pain and the adequacy of analgesia. Pain assessment tools such as the visual analogue scale or numeric rating scale are most useful. In noncommunicative patients, assessment of behavioral (e.g., movements, facial expressions, posturing) and physiologic (e.g., heart rate, blood pressure, respiratory rate) indicators are necessary. It is paramount that the surgical staff and house officers communicate effectively with ICU nurses because their frequent assessments of pain consistently seem to lead to earlier and timelier administration of pain medications. Nurse-driven protocols for pain assessment and management have been shown to reduce ventilator days and ICU length of stay while at the same time providing patients with good pain relief.3 The key concepts of nurse-driven protocols in the ICU are that they not only allow for but encourage nursing staff to use and hone their clinical assessment skills, especially useful because they have almost continuous interaction with their patients.
Epidural anesthesia is achieved by delivering drugs via a catheter placed in the extradural or epidural space. Many benefits of epidural anesthesia have been reported, including better suppression of surgical stress and pain, reductions in circulating levels of proinflammatory mediators, minimized hemodynamic effects, improved peripheral circulation, and reduced blood loss. A recent meta-analysis of 58 trials of thoracic and abdominal surgery in almost 6000 patients has also demonstrated that epidural opioid analgesia is associated with a decreased risk of postoperative pneumonia when compared with systemic opioid regimens; the report also cited a decreased need for prolonged mechanical ventilation and reintubation, along with improved oxygenation.4 Patient-controlled epidural anesthesia is also possible and combines many of the benefits of PCA and epidural anesthesia.
Sedation
Inability to communicate, constant noise and light, disrupted sleep-wake cycles, various other stimuli common to the ICU setting, and immobility contribute to increased anxiety among ICU patients, which may be compounded further by mechanical ventilation. Sedation is necessary to alleviate anxiety and provide comfort, and prevent the patient from removing lines, catheters, and other necessary equipment. A predetermined sedation goal should be established and the level of sedation should be documented objectively based on a sedation scale such as the Richmond Agitation and Sedation Scale (RASS; Table 23-1). RASS is a rapid, simple construct that has been shown to detect changes in sedation in ICU patients over several days and facilitates the proper administration of analgesic and sedative medications. The nurse-driven protocols described earlier for managing pain and analgesic administration also apply to managing sedation. Much of the recent literature regarding pain and sedation protocols overlaps and also supports nurses implementing such protocols to assess and manage their patients’ sedation needs actively when they are properly trained and educated about their use.5
Table 23-1 Richmond Agitation Sedation Scale
SCORE | TERM | DESCRIPTION |
---|---|---|
+4 | Combative | Overly combative or violent; immediate danger to staff |
+3 | Very agitated | Pulls or removes tubes or catheters; has aggressive behavior toward staff |
+2 | Agitated | Frequent nonpurposeful movement; patient-ventilator dyssynchrony |
+1 | Restless | Anxious or apprehensive but movements not aggressive or vigorous |
0 | Alert and calm | |
−1 | Drowsy | Not fully alert, but has sustained (>10 sec) awakening with eye contact to voice |
−2 | Light sedation | Briefly (<10 sec) awakens with eye contact to voice |
−3 | Moderate sedation | Any movement (but no eye contact) to voice |
−4 | Deep sedation | No response to voice, but any movement to physical stimulation |
−5 | Unarousable | No response to voice or physical stimulation |
Adapted from Ely EW, Truman B, Shintani A, et al: Monitoring sedation status over time in ICU patients: Reliability and validity of the Richmond Agitation Sedation Scale (RASS). JAMA 289:2983–2991, 2003.
Figure 23-1 is an algorithm for the provision of analgesia and sedation in the ICU. It is important to be aware of the somewhat poorly understood propofol infusion syndrome, which manifests as rhabdomyolysis, lactic acidosis, and circulatory collapse. It is postulated that the likelihood of developing propofol infusion syndrome is increased if the patient is receiving concurrent vasopressors or steroids. The mechanism of this syndrome probably centers on decreased fatty acid metabolism coupled with damage to mitochondria, resulting in cardiac and peripheral myocyte dysfunction.
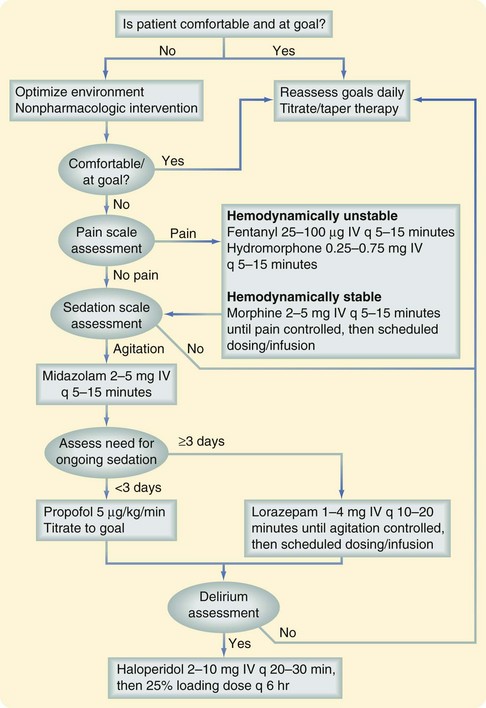
FIGURE 23-1 Algorithm for analgesia and sedation in the ICU.
(Adapted from Jacobi J, Fraser GL, Coursin DB, et al: Clinical practice guidelines for the sustained use of sedatives and analgesics in the critically ill adult. Crit Care Med 30:119–141, 2002.)
Dexmetomidine, a highly specific α2-adrenergic receptor agonist, has been increasingly used, especially in intubated patients, because it has been shown to decrease the amount of narcotic analgesics and sedatives that patients require while also providing a beneficial reduction in myocardial oxygen demands. Its main advantage over other agents is that it provides adequate sedation without significantly limiting a patient’s respiratory drive so it can be used more easily and result in extubation.6 Propofol and benzodiazepines are difficult to titrate to desired effect. Typically, when they are stopped in anticipation of extubation, patients become agitated to the point of requiring remedication, which may delay extubation and ventilator weaning. Recently, randomized data have shown decreased times of mechanical ventilation and ICU length of stay for patients placed on dexmedetomidine infusions for weaning from mechanical ventilation. The most significant disadvantages of using dexmedetomidine are its potential for creating hypotension and its cost, because it may be almost ten times more expensive than midazolam or propofol.
Neuromuscular Blockade
The nondepolarizing NMBs bind Ach receptors but do not activate them, thus blocking the receptor and inhibiting its function. There are two types of nondepolarizing NMBs, steroidal and nonsteroidal. The aminosteroidal compounds include agents such as rocuronium, vecuronium, and pancuronium. Rocuronium has a rapid onset of action and intermediate duration of action, making it useful for short procedures and prolonged relaxation. Vecuronium is an intermediate- acting agent, achieving NMB within 1 to 2 minutes and lasting about 30 minutes, but it can also be infused continuously. Patients with renal or hepatic dysfunction may have a prolonged response because vecuronium is cleared by the kidneys and liver. Pancuronium is long-acting (up to 90 minutes) and is relatively contraindicated in patients with coronary artery disease because its associated vagolytic effect causes pronounced tachycardia. Like vecuronium, pancuronium is eliminated via both the kidneys and liver and requires dose adjustments in the setting of renal or hepatic dysfunction. The nonsteroidal, nondepolarizing NMBs, or benzylisoquinolonium compounds, include atracurium, cisatracurium, tubocurarine, and mivacurium. Of these, atracurium and cisatracurium are the two agents most commonly used in the ICU. Atracurium is intermediate-acting with minimal cardiovascular effects but does have the tendency to promote histamine release. It is metabolized by plasma ester hydrolysis and spontaneous degradation; thus, it is most useful for patients with hepatic and renal dysfunction. A metabolite of atracurium may precipitate seizure activity if used at extremely high doses. Cisatracurium is an isomer of atracurium, with fewer tendencies to produce histamine release. Like atracurium, elimination is by ester hydrolysis and Hofmann elimination. An algorithm for the provision of NMB in the ICU is outlined in Figure 23-2.
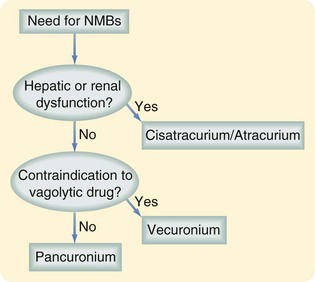
FIGURE 23-2 Algorithm for NMB in the ICU.
(Adapted from Murray MJ, Cowen J, DeBlock H, et al: Clinical practice guidelines for sustained neuromuscular blockade in the adult critically ill patient. Crit Care Med 30:141–156, 2002.)
Cardiovascular System
Hemodynamic Monitoring
Arterial Catheters
Arterial catheter placement is indicated if vasoactive drugs are being administered, continuous monitoring of systemic arterial pressure is required, or frequent arterial blood gas monitoring is needed. The primary complications associated with arterial catheters are infections, arterial thrombosis, and ischemic injuries. A widespread misconception is that the infectious risk associated with arterial catheters is much lower than that of a central venous catheter, but recent reviews of a number of ICUs has found no significant difference in the colonization and infection rates of arterial lines and central venous catheters.7 Arterial catheters must be placed under sterile conditions. Thrombosis with distal ischemia can be minimized by placing catheters in arteries with good collateral circulation. Thus, the radial or dorsalis pedis arteries are preferred to the brachial or femoral arteries. Allen’s test should be performed prior to placement of a radial artery catheter to document adequate collateral flow from the ulnar artery. Ulnar arterial lines are never acceptable because most hands are dominantly perfused by this artery. It appears that placement of difficult arterial lines may be facilitated by the use of ultrasound, likely resulting in fewer attempts. Best medical practice dictates that the need for indwelling medical devices, including arterial lines, in an ICU patient be evaluated daily and that all devices be removed from the patient as soon as possible to minimize nosocomial infections.8
Central Venous Catheters
Central venous catheter placement may be indicated for long-term venous access to provide parenteral nutrition or chemotherapeutic agents or to measure central venous pressure (CVP). The most common complications associated with central venous catheter insertion include dysrhythmias, pneumothorax (up to 5% following subclavian vein placement), arterial puncture with resultant intimal flap, pseudoaneurysm formation, hemorrhage or arteriovenous fistulization, air or catheter embolism, and even death. These complications represent technical errors, which emphasizes the importance of knowledge of anatomy and proper insertion techniques. Additionally, catheter-related bloodstream infections are a major cause of preventable morbidity and mortality in ICU patients that can be diminished by adherence to full barrier precautions, a chlorhexidine-alcohol skin preparation at the time of insertion, and removal of catheters as soon as possible.8
Pulmonary Arterial Catheters
There are also newer noninvasive approaches to hemodynamic monitoring, including pulse contour cardiac output analysis, lithium dilution, and peripheral catheter transpulmonary thermodilution, although no studies to date have demonstrated superior outcomes with these new technologies compared with the PAC. Despite early studies that showed promise in using the transpulmonary thermodilution technique to estimate intrathoracic blood volume, cardiac filling pressures, and preload, a more recent larger, prospective, multicenter multinational study did not show any clinical benefit compared with a PAC.9 Even though there is a paucity of data supporting the use of these newer technologies in the critically ill, it is still worth pursuing randomized prospective data because it is hoped that these technologies will afford the ICU clinician additional noninvasive options for hemodynamic monitoring and patient care.
Esophageal Doppler, a technique introduced over 30 years ago, can measure blood flow velocity in the descending aorta continuously to determine cardiac output and stroke volume. Although the pulmonary artery catheter relies on pressure measurements that can be affected by a number of neural and hormonal influences and thermodilution principles, the esophageal Doppler system directly measures flow velocity, so it is a potentially more direct indicator of cardiac performance.10 Esophageal Doppler measurement of left ventricular outflow stroke volume is similar to the technique of transesophageal echocardiography; studies have shown that placement of the catheter and probe is relatively easy and can rapidly produce data that can instantaneously and continuously yield cardiac output. When measured against the standard of thermodilution, measurements were found to be in good correlation with those of the PAC and that the variations in hemodynamics of individual patients yielded similar changes in cardiac output between the two monitoring methods.10 Significant disadvantages and drawbacks of esophageal Doppler monitoring include its invasiveness, its almost universal requirement for deep sedation and mechanical ventilation, and frequent need for repositioning. Also, the angle between the probe and flow of blood remain fairly constant and relies on many assumptions—for example, that the esophagus is exactly parallel to the descending aorta, blood flow remains fairly constant in distribution between the brachiocephalic, descending aortic, and coronary circulations, and the flow velocity is fairly uniform between blood flowing in the center of the descending aorta and the peripheral aspects of the vessel.
Cardiovascular Dysfunction
Shock
Shock is simply defined as perfusion that is inadequate to meet the body’s metabolic needs. Management of the patient in shock is focused on the following: (1) identifying the presence of shock; (2) searching for and treating immediately life-threatening conditions; and (3) treating shock based on the underlying pathophysiology (see Chapter 5).
After addressing immediate threats to life, it is important to identify and treat the underlying cause of shock. Shock may be broadly classified into five categories: hypovolemic, cardiac compressive, neurogenic, septic, and cardiogenic. Hypovolemic shock may be caused by third spacing of fluid, gastrointestinal or insensible losses, or hemorrhage. Hypovolemic shock secondary to acute blood loss is more accurately termed hemorrhagic shock. A crystalloid (isotonic saline) bolus (20 mL/kg) should be administered immediately, and repeated if necessary. In addition to its therapeutic benefit, the response to fluid may help confirm the assessment of hypovolemia. Glucose-containing fluids should be avoided because they may stimulate an osmotic diuresis. If hemorrhage is suspected and the hemodynamic response to crystalloid is not satisfactory, a blood transfusion should be initiated without delay and a search for the source of hemorrhage aggressively undertaken. The rapidity of resuscitation is predicated on the patient’s condition; restoration of normal blood pressure, heart rate (HR), skin color, mentation, and urine output signify a reversal of hypoperfusion. The need for continued resuscitation may be estimated by additional measurements (see later, “End Points of Resuscitation”). In the setting of hemorrhagic shock or ongoing bleeding, it is prudent to restore hemoglobin (Hb) to near-normal levels in the acute phase.
Septic shock represents cardiovascular collapse associated with an infectious process and represents the final stage on the continuum from systemic inflammatory response syndrome (SIRS) to sepsis and septic shock. Management of septic shock involves treating the underlying infectious process (source control), administering appropriate antibiotics (see later, “Sepsis”), and volume resuscitation. Cardiogenic shock refers to primary pump failure and is associated with high cardiac filling pressure and diminished cardiac output. Although fluid administration is a key component in treating shock, no matter what the cause, each type of shock requires additional interventions; thus, the prompt identification of shock’s cause is critical to a good outcome.
Support of Circulation
The CaO2 consists of the O2 that is carried by Hb and that which is dissolved into the blood itself:
Dysrhythmias
Dysrhythmias are common in the ICU and correct interpretation of the rhythm is the key to proper treatment. In a patient with cardiopulmonary arrest, it is helpful to diagnose the rhythm with quick-look paddles. The most recent algorithm from the American Heart Association stresses the need for almost continuous cardiopulmonary resuscitation (CPR) and presents guidelines for CPR, including advanced cardiac life support; it is updated approximately every 5 years (Box 23-1). For ventricular fibrillation or pulseless ventricular tachycardia, defibrillation with 360 J (monophasic) or 120 to 200 J (biphasic) should be delivered but should not be repeated or stacked without resuming another cycle of CPR because this interrupts chest compressions and decreases coronary perfusion pressure . If the phase type of the defibrillator is unknown, 200 J should be selected; automatic external defibrillators will administer their preprogrammed electrical dose. If electrical defibrillation is not successful, CPR should continue and the following steps should be taken:
Box 23-1 Adapted from ECC Committee, Subcommittees and Task Forces of the American Heart Association: American Heart Association Guidelines for Cardiopulmonary Resuscitation and Emergency Care, Part 7.2: Management of Cardiac Arrest. Circulation 112(Suppl):IV1–IV59, 2005.
Guidelines for Management of Cardiopulmonary Arrest
Ventricular Fibrillation and Pulseless Ventricular Tachycardia
Give one shock (monophasic, 360 J; biphasic, 100-200 J).
CPR; additional countershocks if shockable rhythm obtained
Consider amiodarone (300 mg IV), lidocaine (1-1.5 mg/kg), magnesium (1-2 g IV).
If no shockable rhythm, revert to asystole or pulseless electrical activity (PEA) algorithm.
Asystole should be verified by rotating leads and high-quality CPR should be initiated. Epinephrine (1 mg IV) or a one-time dose of vasopressin (40 U IV) should be given. Atropine (1 mg IV) should also be given and repeated every 5 minutes, for a total of three doses. Countershock should only be given if fine ventricular fibrillation is suspected. There is no role for antiarrhythmics or defibrillation in asystolic cardiac arrest (see Box 23-1).
The most common sustained dysrhythmia is atrial fibrillation, with a prevalence of 5% in patients older than 65 years. Numerous stresses in the perioperative period may trigger new-onset atrial fibrillation or loss of rate control in the patient with chronic atrial fibrillation. Cardioversion should be performed for hemodynamic instability; otherwise, rate control is attempted while the underlying cause (e.g., myocardial ischemia, fluid overload, electrolyte imbalances, hypoxemia, acidosis, pulmonary embolism [PE]) is identified and treated. IV amiodarone, calcium channel blockers, or beta blockers are usually effective in rapid conversion; digoxin takes several hours for maximal effect. There are also a number of new medications that can be used for conversion of atrial fibrillation to sinus rhythm, notably the class III antiarrhythmic agent dofetilide. However, potential drug-related complications such as torsades de pointes and the need for renal dosing limit their usefulness and make rate control and use of beta blockers and calcium channel blockers the preferred medical management for atrial fibrillation.11 In patients who have had atrial fibrillation for less than 48 hours, or who are already on warfarin, no anticoagulation is necessary. If the precise time of onset is not known, however, it is probably safest to anticoagulate prior to cardioversion or perform cardioversion under guidance by transesophageal echocardiography. A number of randomized trials have addressed rate control and rhythm conversion in patients with atrial fibrillation. Conversion may be detrimental in some patient populations because of toxicities of antiarrythmic medications but may provide some benefit in terms of improved quality of life and left ventricular function in patients with heart failure.
Pump Dysfunction
Hypotensive patients may require medication to augment cardiac contractility, increase systemic arterial vasoconstriction, or both. There are several agents that may be used, each having a unique profile of activity on adrenergic receptors (Table 23-2). α1-Adrenergic receptors have a primary effect on systemic arterial vasoconstriction, and lesser effects on systemic veins and pulmonary arteries. β1-Adrenergic receptors act primarily on the heart, increasing HR, contractility, and atrioventricular conduction. β2-Adrenergic receptors increase HR and contractility, but are also vasodilatory to the systemic and pulmonary vasculature. Dopaminergic receptors modulate arterial vasodilatation and, to a lesser degree, cardiac contractility, but the effects of dopamine are unpredictable and the side effects may be substantial; therefore, enthusiasm for its use in the ICU has been waning.
Vasopressin is commonly used by critical care physicians; it is not an adrenergic drug but instead functions through an independent G protein–coupled receptor and thus may be useful for patients who are refractory to catecholamines. Its main function in the body appears to be regulation of water balance but, in shock, it is a potent vasopressor, irrespective of the level of circulating endogenous vasopressin. In septic shock, it is typically administered in doses up to 0.04 U/min, which mimic physiologic levels and avoids some of the adverse effects associated with higher doses, such as myocardial or splanchnic ischemia. One of the primary advantages of vasopressin in septic shock is that it continues to have an effect in the setting of profound metabolic acidosis, whereas adrenergic agents typically have decreased efficacy in acidemic states. Despite this, vasopressin was found to be no more beneficial than norepinephrine in terms of 28-day mortality in a fairly large multicenter randomized trial of patients in septic or severe septic shock.12 Also, vasopressin is a potent peripheral vasoconstrictor; in shock states, these effects can worsen splanchnic and renal ischemia more than adrenergic agents such as norepinephrine that provide some inotropic and chronotropic effects.
In patients who have an adequate MAP but need augmented myocardial contractility, inotropic drugs are indicated. Generally, these drugs have vasodilatory effects, so it is important to ensure adequate preload prior to infusion. Dobutamine (5 to 15 µg/kg/min) can be effective, but it does increase myocardial oxygen demand and may be arrhythmogenic. Isoproterenol is a powerful synthetic β-adrenergic agonist that is no longer used in clinical practice because of its associated arrhythmogenicity. The phosphodiesterase inhibitors amrinone and milrinone are thought to act by inhibiting the breakdown of cyclic adenosine monophosphate (cAMP). They increase CO and reduce preload and afterload; amrinone may cause profound vasodilation and its long-term administration is associated with thrombocytopenia and gastrointestinal side effects. Milrinone is a more potent inotrope with fewer side effects but it is also associated with vasodilation and arrhythmias. It causes pulmonary vascular vasodilation and may be helpful in treating myocardial dysfunction in the setting of pulmonary hypertension. The phosphodiesterase inhibitors appear to be able to increase myocardial contractility without affecting myocardial oxygen demand by reducing wall stress, which counteracts the increased oxygen requirement to support enhanced contractility (Table 23-2).
Resuscitation
Fluids
Fluid resuscitation is the initial maneuver whenever a shock state is recognized. Crystalloid is typically administered to expand the intravascular volume, but only about one third of the fluid will remain in the intravascular space. Additionally, in many shock states, there may be cellular dysfunction, resulting in loss of capillary integrity and massive fluid extravasation and causing widespread tissue edema. For many decades, a debate about the advantages and disadvantages of crystalloid versus colloid fluid resuscitation has persisted. Although colloids provide more effective volume expansion than crystalloid solutions because of their ability to remain in the vascular space, prospective, randomized, clinical trials (PRCTs) have demonstrated that survival is no better and possibly worse when albumin is given instead of crystalloid.13 This argument has been countered by meta-analyses showing that the administration of albumin reduces morbidity in acutely ill hospitalized patients14 and may have beneficial effects in a wide range of clinical settings. Whatever the outcome of this debate, it is apparent that many more studies will be required before a definitive conclusion is reached. However, in the case of severe hemorrhagic shock, it is certain that the usual transfusion triggers and colloid-crystalloid debate do not apply, and Hb should be restored to near-normal levels.
There has also been some recent enthusiasm for the use of synthetic colloid analogues—namely, hydroxyethyl starch solutions—for septic, hypovolemic, or hemorrhagic shock states; it is thought that a much larger percentage of the synthetic solutions would remain in the intravascular space compared with that of crystalloid. No clinical studies have shown any benefit in using these solutions in surgical or critically ill patients; investigations of fluid resuscitation end points have found that the volume of these starch solutions required to reach these end points far exceeds the expected amount in relation to the amount of crystalloids given.17 Actually, almost equal volumes of the synthetic starch solutions and crystalloid were given to reach the resuscitation end points. There are also serious potential deleterious effects of synthetic starch solutions, including coagulopathy, with decreased levels of circulating factor VIII and von Willebrand factor, platelet dysfunction, nephrotoxicity, and pruritus. Other studies have also shown an increased need for renal replacement therapy (RRT) in patients with sepsis who have been given synthetic starch solutions. Newer hydroxyethyl starch (HES) solutions of different compositions (e.g., HES 130/0.4) are still unproven in terms of their potential to cause less hemostatic compromise or renal failure. Recent reports of these synthetic solutions have questioned their overall safety in critically ill surgical patients; thus, it is probably prudent to avoid using these agents at this time.15
End Points of Resuscitation
Although fluid resuscitation may normalize many clinical parameters such as HR, blood pressure, skin color, mentation, and urine output, it does not ensure that the O2 debt has been repaid. Thus, there should be an objective measure of the success of resuscitation in meeting tissue metabolic needs. In the early 1990s, Bishop and colleagues16 have identified values for CI (4.5 liter/min/m2), DO2 (600 mL O2/min/m2) and O2 consumption (; 170 mL O2/min/m2) above which survival could be predicted in critically ill patients. Subsequent PRCTs testing these resuscitation goals offered mixed results. Kern and Shoemaker17 have reviewed published data and suggested that if hemodynamic optimization is applied to subgroups with an expected mortality of 20% or higher, prior to the development of organ failure, and the goal of increased DO2 is achieved, then survival will be improved. It is difficult to prove definitively that early aggressive resuscitation benefits critically ill patients, but it is important to augment DO2 whenever possible, because this is the basis of goal-directed resuscitation.18 It must be recognized, however, that not all patients respond in the same way. For example, Moore and associates19 have reported that 38% of severely injured patients are unable to attain a
of 150 mL O2/min/m2, despite supranormal DO2. This group appeared to have defective aerobic metabolism, leading to a higher incidence of multiple organ failure (MOF). Thus, routine resuscitation to supranormal targets may be unnecessary and inappropriate when shock is readily reversed, fruitless when the patient does not respond, and even detrimental when it results in the abdominal compartment syndrome (ACS).
Recently, there have been inquiries into the potential detriments and benefits of supranormal fluid resuscitation or conservative fluid administration strategies. These inquiries are mainly based on the theorized mechanism of overzealous fluid resuscitation causing increased cardiac filling pressures and resultant pulmonary edema and impaired gas exchange. Post hoc analysis of the Fluid and Catheter Treatment Trial (FACTT) from 2006 has shown that surgical patients managed with a conservative fluid strategy that aimed for lower CVPs, PCWPs, and urine output than those managed with a liberal strategy have significantly fewer days on a ventilator, without any increase in mortality or renal failure.20 Further study is warranted to continue to assess the potential benefits of such conservative fluid strategies in a wider range of surgical patients.
The major drawbacks to the widespread use of gastric tonometry are technologic limitations, cost, and inconvenience. A number of investigators have measured transcutaneous O2 and CO2 levels, as well as skeletal muscle oxyhemoglobin. Early results have been encouraging in a military setting with tissue oxygen monitors placed in the thenar muscle bed guiding trauma resuscitations and have correlated well with clinical findings of hemorrhagic shock in small series of patients. This technology promises a useful device for guiding and monitoring massive resuscitations; however, its usefulness for other forms of shock resuscitation remains unknown.2 Arterial lactate levels and base deficit are measures of global tissue perfusion and can be particularly helpful in predicting which patient will experience an adverse outcome, because the time to normalization strongly correlates with mortality and morbidity.21 In addition to their prognostic significance, these parameters allow the degree of physiologic derangement to be quantified, and they serve as targets for ongoing resuscitation, but it appears that lactate levels may offer an improved predictive ability compared with base deficit.
The Surviving Sepsis Campaign, an international multiorganization effort to reduce the mortality associated with sepsis, is noteworthy for assembling a concise set of resuscitation principles that stresses early targeted physiologic goals.22 This evidence-based campaign began in 2002 and was updated in 2004 and 2008, with the goal of improving the management of severely septic and septic shock patients. Elements of the guidelines were “bundled” into two sets of targets: (1) the resuscitation bundle, which sets goals of care, data to be gathered, and tasks to be accomplished within 6 hours of a septic patient’s presentation; and (2) a second management bundle, less geared toward data gathering and more dedicated to actual management of the physiologic derangements of sepsis. The resuscitation bundle guides early fluid resuscitation and vasopressor support based on the presence of hypotension and/or elevated lactate levels, and targets a MAP higher than 65 mm Hg. If this goal is unmet, CVP is targeted to 8 mm Hg or higher or a central venous oxygen saturation of 70% or higher. In the next phase, the administration of corticosteroids for septic shock, consideration of activated protein C, glucose control, and maintenance of lower ventilatory plateau pressure are addressed.22 For all septic patients, it must be emphasized that identification of the cause of sepsis is crucial so that eradication or source control can be carried out; without it, the elements of this bundle stand little chance of reducing septic mortality.
With the establishment of these management principles, the campaign recruited over 250 hospital sites and has recently reported a steady decrease in in-hospital mortality of over 15,000 septic patients. This is the largest prospective evaluation of patients with severe sepsis that has ever been conducted.22 Interestingly, the longer a hospital used these guidelines, the better the survival benefit, and bundle compliance correlated with reductions in septic deaths. Although the campaign has been successful in reducing sepsis-related mortality, this success may be dependent more on an organized approach to caring for septic patients rather than on the actual components of the bundles themselves because the bundles sometimes contained elements that were or remain controversial or were subsequently shown to be ineffective in treating sepsis. With few exceptions, every prospective, goal-directed clinical trial that has shown a survival advantage has espoused the principles of the supranormal DO2 strategy—volume loading with or without transfusion plus inotropic support as needed to meet a predetermined goal. The optimal algorithm for fluids and inotropes remains to be determined, but it is clear that a defined end point is desirable. Rather than selecting a goal that simply confirms the act of resuscitation, it is more appropriate to select an end point that confirms a response to resuscitation.
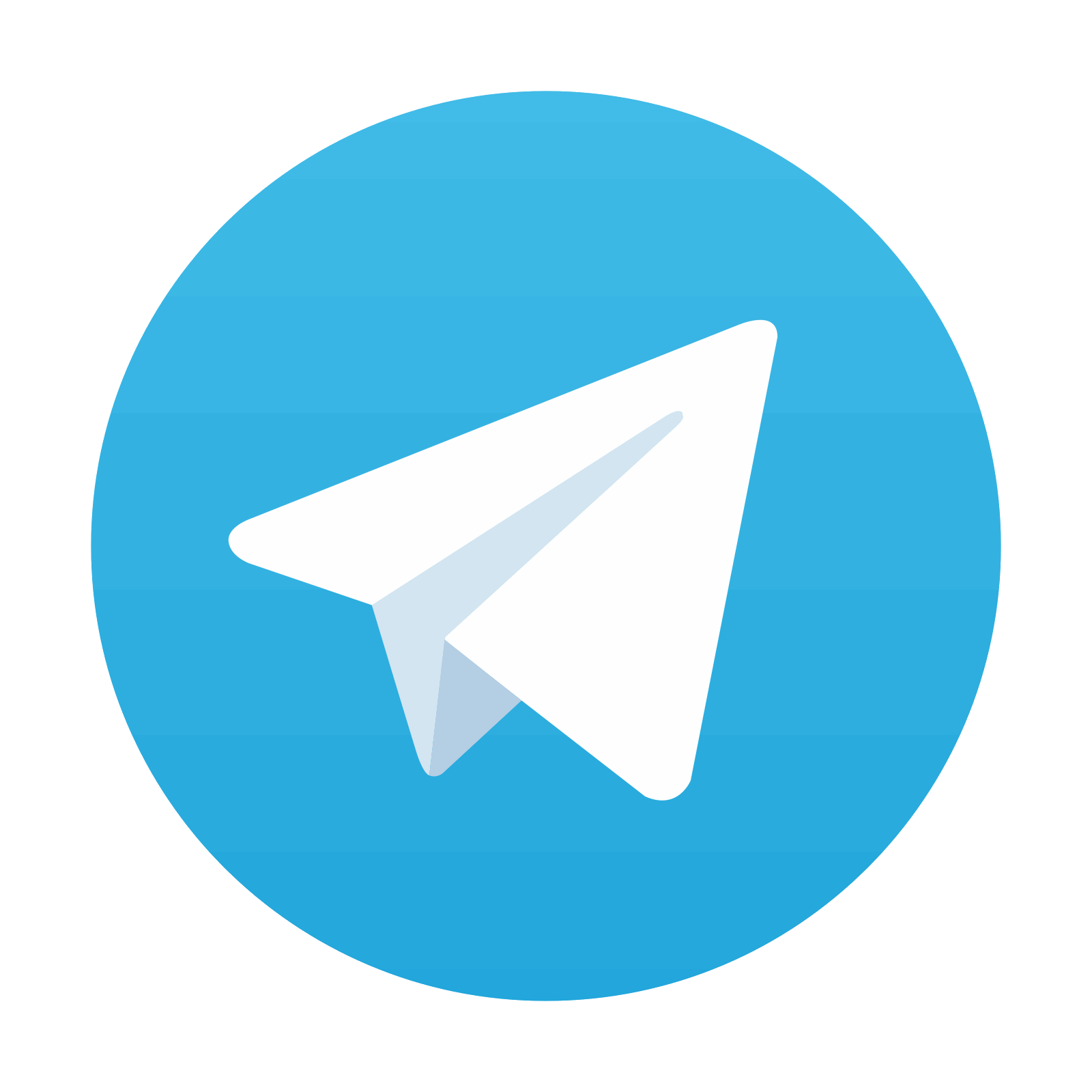
Stay updated, free articles. Join our Telegram channel

Full access? Get Clinical Tree
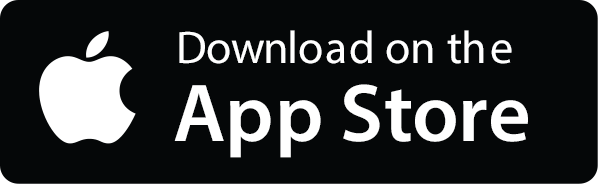
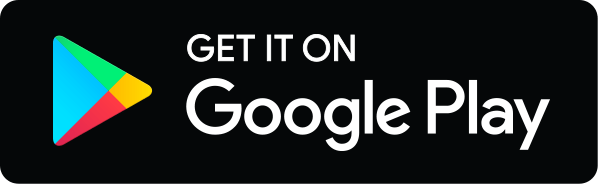