Supraventricular Tachycardias
The development and refinement of the techniques of intracardiac recording and programmed stimulation, and our ability to compare these findings with experimental models, have resulted in a greater understanding of the physiologic mechanisms responsible for paroxysmal supraventricular tachycardias (SVTs). This has led to a more meaningful classification of these tachycardias. This chapter discusses analysis of SVT using these techniques.
Mechanisms of Supraventricular Tachycardia
Our concepts of arrhythmogenic mechanisms have evolved over the past century. The two basic mechanisms responsible for SVT (and for that matter, all tachycardias) are enhanced impulse formation and abnormalities of conduction leading to reentry.1,2 Enhanced impulse formation may be due to enhanced phase 4 automaticity in normal or abnormal cells (abnormal automaticity) or to triggered activity. Tachycardias that are due to enhanced automaticity, either normal or abnormal, can in general be neither predictively initiated nor terminated by electrical stimulation. Although experimental models suggest that annihilation of an automatic focus (not a tachycardia) is possible,3 this is not predictable. The most common response of automatic rhythms to overdrive pacing is either overdrive suppression or no effect whatsoever.1,2,4 Triggered rhythms that are due to delayed afterdepolarizations have been demonstrated in atrial and coronary sinus tissue under a variety of experimental conditions.5,6,7 Observations in animal models and in vitro preparations have shown that electrical stimulation can induce tachycardias that are due to triggered activity secondary to delayed afterdepolarizations; however, the initiation of such arrhythmias is most consistently produced by overdrive pacing and is not associated with conduction delay and/or block. It is facilitated by catecholamines. Specific responses such as (a) a direct relationship between the coupling interval or paced cycle length initiating the rhythm and the interval to the onset of the rhythm and early cycle length of the rhythm and (b) overdrive acceleration are typical of such arrhythmias and distinguish them from reentrant arrhythmias (see following discussion).4,5,6,7 The incidence of triggered activity as the mechanism of spontaneous paroxysmal SVTs is uncertain and, with the possible exception of digitalis-induced atrial tachycardias, is certainly uncommon.8
The second mechanism is reentry, which is an expression of abnormalities of impulse propagation.1,2 These may be based on anatomic, functional, or a combination of anatomic and functional abnormalities, which provide the electrophysiologic inhomogeneity required for sustained reentry. The following three conditions are required for the initiation and maintenance of a reentrant rhythm: (a) at least two functionally (or anatomically) distinct potential pathways that join proximally and distally to form a closed circuit of conduction; (b) unidirectional block in one of these potential pathways; and (c) slow conduction down the unblocked pathway, allowing the previously blocked pathway time to recover excitability; that is, the conduction time along the alternative pathway must exceed the refractory period of the initially blocked pathway. If, and only if, conduction delay and refractoriness in both pathways are appropriate, a continuously circulating wavefront of electrical activity will ensue, resulting in a tachycardia. The sine qua non of a reentrant arrhythmia is the ability to reproducibly initiate the tachycardia by timed extrastimuli (particularly in association with evidence of conduction delay and block). Similarly, programmed stimulation can also reproducibly terminate the tachycardia. The mode of initiation and response to stimulation during a reentrant tachycardia differs from that of triggered activity. Timed extrastimuli are more effective than rapid pacing for initiation; there is no direct relationship of pacing cycle length or coupling of extrastimuli to the interval to the onset of the tachycardia or cycle length of the tachycardia; and resetting or entrainment of the tachycardia in the presence of fusion can be demonstr-ated.1,2,4,7 The latter two phenomena are diagnostic of reentry.9,10 In view of these responses to stimulation, the vast majority of paroxysmal SVTs appear to be reentrant.
Methods of Evaluation
Incremental pacing and programmed extrastimuli from both the atrium and ventricle may be used to initiate SVTs (see Chapter 2). After a tachycardia has been initiated, the stimulator is synchronized in, and triggered by, a suitable intracardiac
electrogram. One or more extrastimuli and overdrive pacing are then introduced to modify (reset, entrain, or terminate) the arrhythmia. The use of these techniques has demonstrated that most SVTs are due to reentry and that the general diagnostic category, SVT, is in reality composed of several different arrhythmias. Mechanisms and site of origin of SVT can be defined and classified by analysis of specific phenomena (Table 8-1) including the following:
electrogram. One or more extrastimuli and overdrive pacing are then introduced to modify (reset, entrain, or terminate) the arrhythmia. The use of these techniques has demonstrated that most SVTs are due to reentry and that the general diagnostic category, SVT, is in reality composed of several different arrhythmias. Mechanisms and site of origin of SVT can be defined and classified by analysis of specific phenomena (Table 8-1) including the following:
TABLE 8-1 Electrophysiologic Evaluation of Supraventricular Tachycardia | |
---|---|
|
The mode of initiation of the tachycardia, with particular attention to the site of conduction delay, which appears requisite for the development of the arrhythmia: to help distinguish reentry from triggered activity,4,7 one should also analyze the relationship of the basic drive cycle length and the coupling interval of the extrastimulus that initiates the tachycardia to the onset of the tachycardia and the initial cycle length of the tachycardia.
The atrial activation sequence and relationship of the P wave to the QRS complex at the onset of and during the tachycardia.
The effect of bundle branch block, spontaneous or induced, on the cycle length and ventriculoatrial (V-A) conduction time during the tachycardia.
The requirement of atrial, His bundle, and/or ventricular participation in the initiation and maintenance of the tachycardia, that is, the effect of A-V dissociation or variable A-V or V-A conduction on the tachycardia.
The effect of atrial and/or ventricular stimulation during the tachycardia. Response to such stimulation allows one to assess the role of atrial, His bundle, and ventricular participation in the tachycardia and can be used to distinguish atrial tachycardia, A-V nodal tachycardia, and tachycardias using concealed accessory pathways from one another. Responses to extrastimuli can establish and quantitate the presence of an excitable gap within the reentrant circuit.10,11,12,13,14
The effects of drugs and/or physiologic maneuvers on the tachycardia. This allows one to characterize the properties of the tachycardia circuit as well as to establish which components of the heart are required to maintain the tachycardia.
TABLE 8-2 Mechanisms of SVT (2,789 Patients) | ||||||||
---|---|---|---|---|---|---|---|---|
|
The mechanisms and frequency of SVTs based on the results of such investigations and observations are listed in Table 8-2.
Supraventricular Tachycardia Resulting from Atrioventricular Nodal Reentry
Supraventricular tachycardia due to A-V nodal reentry (AVNRT) is the most common form of SVT, accounting for approximately 50% of the cases.15,16,17,18 This arrhythmia is more common in women and usually occurs before age 40, with a median age of onset of 28. However, this arrhythmia may be seen in children or the elderly. We have recently studied three nonagenarians with severely symptomatic AVNRT. The rates of AVNRT tachycardia vary widely. In our patient population, we have seen SVT rates ranging from 100 to 280 beats per minute (bpm) with a mean of approximately 170 bpm. Rates are slower in patients over 50 than in those under 50. Holter monitoring in such patients demonstrates atrial premature depolarizations (APDs) and isolated atrial echo beats between episodes.
The concept of AVNRT as a mechanism of SVT was first proposed by Mines19 in 1913. Moe et al.20 were the first to postulate that SVT could be produced by longitudinal dissociation of the A-V node (AVN) into two pathways in the AVN. They were also the first to demonstrate that atrial extrastimuli could actually initiate and terminate SVT in the rabbit.21 Based on their experiments, these investigators postulated the presence of a dual A-V nodal transmission system with a slowly conducting alpha pathway with a short refractory period and a fast-conducting beta pathway with a longer refractory period. During sinus rhythm the impulse traverses the faster-conducting beta pathway to produce a single QRS complex (Fig. 8-1A). The impulse simultaneously conducts down the alpha pathway (slow), reaching
the lower part of the AVN or His bundle shortly after it has been depolarized and rendered refractory by the impulse that has conducted down the beta pathway. When an APD is delivered (Fig. 8-1B), the impulse blocks in the beta pathway, because of its longer refractory period, and proceeds slowly down the alpha pathway. This leads to a prolonged P-R interval. If conduction down the alpha pathway is slow enough to allow the previous refractory beta pathway time to recover, an atrial echo results. If, however, the alpha pathway does not itself recover excitability in time to permit subsequent antegrade conduction, only a single atrial echo results. An earlier APD (Fig. 8-1C) also blocks in the beta pathway, but conducts more slowly down the alpha pathway and arrives later to retrogradely excite the beta pathway, producing an echo. Because of the longer antegrade conduction time, the alpha pathway has now had more time to recover excitability, and a sustained tachycardia results. This schema depicts initiation of the most common form of A-V nodal reentrant tachycardia, typical AVNRT. Rarely, the beta pathway may have a shorter refractory period than the alpha pathway, and the tachycardia can be reversed, conducting antegradely down the fast pathway and retrogradely up the slow pathway to produce the uncommon variety of AVNRT (Fig. 8-2).18,22,23,24,25,26,27,28 Women appear to have greater differences in the refractory periods of the fast and slow pathways, which may be why A-V nodal tachycardia is more common in women.
the lower part of the AVN or His bundle shortly after it has been depolarized and rendered refractory by the impulse that has conducted down the beta pathway. When an APD is delivered (Fig. 8-1B), the impulse blocks in the beta pathway, because of its longer refractory period, and proceeds slowly down the alpha pathway. This leads to a prolonged P-R interval. If conduction down the alpha pathway is slow enough to allow the previous refractory beta pathway time to recover, an atrial echo results. If, however, the alpha pathway does not itself recover excitability in time to permit subsequent antegrade conduction, only a single atrial echo results. An earlier APD (Fig. 8-1C) also blocks in the beta pathway, but conducts more slowly down the alpha pathway and arrives later to retrogradely excite the beta pathway, producing an echo. Because of the longer antegrade conduction time, the alpha pathway has now had more time to recover excitability, and a sustained tachycardia results. This schema depicts initiation of the most common form of A-V nodal reentrant tachycardia, typical AVNRT. Rarely, the beta pathway may have a shorter refractory period than the alpha pathway, and the tachycardia can be reversed, conducting antegradely down the fast pathway and retrogradely up the slow pathway to produce the uncommon variety of AVNRT (Fig. 8-2).18,22,23,24,25,26,27,28 Women appear to have greater differences in the refractory periods of the fast and slow pathways, which may be why A-V nodal tachycardia is more common in women.
Recently, the concept of dual A-V nodal pathways causing longitudinal dissociation of the AVN permitting AVNRT has
been called into question. Jalife29 has elegantly shown that the jump in conduction times and isolated reentry can occur in a nonhomogenous linear structure if conduction occurs electrotonically across an area of block. Using a sucrose gap model, he demonstrated classic dual-pathway responses in isolated Purkinje fibers. Antzelevitch and Moe30 demonstrated similar responses in an ischemic gap preparation. Thus, electrotonic propagation clearly can produce dual-pathway responses in the absence of longitudinal dissociation and even can produce reflection of the impulse, a form of microreentry, at least for isolated beats. This does not mean that the AVN works by electrotonic conduction across areas of block. Nodal fibers are not inexcitable, and they conduct by slow responses31,32 and not solely by electrotonic interaction.
been called into question. Jalife29 has elegantly shown that the jump in conduction times and isolated reentry can occur in a nonhomogenous linear structure if conduction occurs electrotonically across an area of block. Using a sucrose gap model, he demonstrated classic dual-pathway responses in isolated Purkinje fibers. Antzelevitch and Moe30 demonstrated similar responses in an ischemic gap preparation. Thus, electrotonic propagation clearly can produce dual-pathway responses in the absence of longitudinal dissociation and even can produce reflection of the impulse, a form of microreentry, at least for isolated beats. This does not mean that the AVN works by electrotonic conduction across areas of block. Nodal fibers are not inexcitable, and they conduct by slow responses31,32 and not solely by electrotonic interaction.
![]() FIGURE 8-2 Schema of the atypical form of (A-V) nodal reentry. Here, the A-V node (AVN) is schematically drawn, as in Figure 8-1, but here the beta pathway has fast conduction but a relatively shorter refractory period than the slowly conducting alpha pathway. A: In sinus rhythm, the impulse conducts over the fast pathway, giving rise to a normal P-R interval, as in Figure 8-1. B: However, in response to an APD, block now occurs in the slow pathway, but it is concealed because conduction proceeds over the fast pathway with minimal prolongation of the P-R interval. The impulse conducting over the fast pathway tries to return up the alpha pathway, but it has not recovered excitability, so no echo occurs. C: An even earlier APD blocks again in the slow pathway, which is not manifested on the surface ECG as the impulse proceeds over the fast pathway with slightly longer P-R prolongation. However, as the impulse reenters the slow-conducting alpha pathway, conduction through that pathway proceeds so slowly that the site of block has time to recover excitability and repetitive reentrance occurs, with the development of SVT. Note in this case that because retrograde activation occurs slowly over the alpha pathway, the P wave follows the QRS by a longer R-P interval. See text for further discussion. |
The AVN is a complex three-dimensional structure with posterior and left atrial extensions that are engaged by transitional tissue. Cell-to-cell coupling in the AVN is extremely poor due to heterogenous connexins, both type and distribution, which is the main reason the node is so slowly conducting. In canines the posterior extension and transitional tissue in the posterior triangle of Koch are longitudinally arranged parallel to the tricuspid annulus with scant side-to-side connections. The anatomic characteristics create nonuniform anisotropic properties that could lead to saltatory conduction analogous to that shown by Spach et al.33,34 in atrial tissue and recently by Dillon et al.35 and Cardinal et al.36 in ischemic ventricular muscle. Recently Spach and Josephson37 showed that marked nonuniform anisotropic conduction is present in the posterior triangle of Koch in canines. Efimov’s group most recently described the three-dimensional structure and electrophysiology of the AVN in explanted human using voltage-sensitive dyes. They also described the gap junctional arrangement in the AVN (mostly connexin 45). While these hearts came from people without a history of AVNRT, they were able to see various patterns of reentry which involve the compact node and its extensions and surrounding transitional tissue. The left atrium was uninvolved. All models in which discontinuous propagation occurs can give rise to input–output responses analogous to dual pathways. In nonuniform anisotropic tissue, responses to premature stimuli can create functional longitudinal dissociation and sustained reentry.33,34,35,36 Dual pathways therefore could actually exist wholly secondary to nonuniform anisotropic conduction. Data in humans supporting the dual-pathway concept include the findings of two P-R or A-H intervals during sinus rhythm or at similar paced cycle lengths (Fig. 8-3);38,39 the observation of double responses (1:2 conduction) either antegradely in response to atrial extrastimuli or retrogradely in response to ventricular extrastimuli; and the ability to preempt atrial echoes by ventricular extrastimuli delivered during slow-pathway conduction during SVT.24,27,40,41,42 This latter phenomenon truly demonstrates two parallel pathways capable of conduction, because, if only a single pathway were present, the retrograde impulse from the ventricular extrastimulus would collide with the antegrade impulse and fail to reach the atrium. Thus, the concept of longitudinal dissociation of the AVN with dual A-V nodal pathways and the critical interrelationship of conduction and refractoriness in these two pathways best explain the mechanism of AVNRT.
While much of the literature implies that the fast and slow pathways are anatomically distinct structures, there is no anatomic evidence to support this concept. In fact analysis of the anatomy of the A-V nodal region of patients with and without AVNRT shows no differences and no evidence of specialized pathways.43,44,45 Even the “slow pathway” potentials described by Jackman et al.46 and Hassaguerre et al.47 are nonspecific electrograms that can be recorded in all people in the posterior triangle of Koch.48 The variations in retrograde activation in typical and uncommon forms of AVNRT support lack of anatomic pathways (see discussion of Atrial Activation During A-V Nodal Reentry later in this chapter). Therefore, I believe that it is most likely that electrophysiologic demonstration of dual A-V nodal pathways is a result of the influence of nonuniform anisotropy on propagation in the A-V junctional region.
Mechanisms of Initiation of Atrioventricular Nodal Reentry
The spontaneous initiation of typical AVNRT is almost always by an APD that is associated with a long P-R and A-H interval leading to a tachycardia in which the atria and ventricles are activated nearly simultaneously (Fig. 8-4). Thus, in typical AVNRT, initiation is produced when an APD blocks in the fast pathway and conduction proceeds antegradely over the slow pathway and retrogradely over the fast pathway, as depicted in Figure 8-1. It is logical to assume that if spontaneous APDs were the most frequent method of initiation of AVNRT, then programmed atrial extrastimuli could simulate the spontaneous situation and induce the tachycardia. In nearly 100% of patients with a history of AVNRT their arrhythmia can be induced by programmed stimulation, most commonly by timed APDs introduced over a reproducible range of coupling intervals (Fig. 8-5).15,16,17,18,27,43,49 In some instances, catecholamines and/or atropine are needed
to facilitate induction. We have actually required stimulation during a 30-degree tilt to induce AVNRT in three patients. The zone of coupling intervals of atrial extrastimuli that induces A-V nodal echoes or sustained SVT (i.e., “echo or tachycardia zone”) usually begins at coupling intervals associated with a marked prolongation of the P-R and A-H intervals. A-V nodal conduction delay (i.e., the increase in A-H interval), and not the coupling interval of the APD, is of prime importance in the genesis of A-V nodal reentrant SVT.50 This can be demonstrated by initiation of AVNRT by using the alternative method of atrial pacing to produce A-H delays at longer cycle lengths than at the coupling intervals of single extrastimuli required to produce comparable A-H delays (Fig. 8-6). If one compares the initiation of SVT by atrial extrastimuli with that by atrial pacing, one can demonstrate that only after sufficient A-V nodal conduction delay is achieved, as reflected by the A-H interval, SVT is induced (Fig. 8-7).
to facilitate induction. We have actually required stimulation during a 30-degree tilt to induce AVNRT in three patients. The zone of coupling intervals of atrial extrastimuli that induces A-V nodal echoes or sustained SVT (i.e., “echo or tachycardia zone”) usually begins at coupling intervals associated with a marked prolongation of the P-R and A-H intervals. A-V nodal conduction delay (i.e., the increase in A-H interval), and not the coupling interval of the APD, is of prime importance in the genesis of A-V nodal reentrant SVT.50 This can be demonstrated by initiation of AVNRT by using the alternative method of atrial pacing to produce A-H delays at longer cycle lengths than at the coupling intervals of single extrastimuli required to produce comparable A-H delays (Fig. 8-6). If one compares the initiation of SVT by atrial extrastimuli with that by atrial pacing, one can demonstrate that only after sufficient A-V nodal conduction delay is achieved, as reflected by the A-H interval, SVT is induced (Fig. 8-7).
Dual pathways are identified by a discontinuous response of A-V nodal conduction time to progressively premature APDs. Dual pathways can be identified by either plotting the A1-A2 coupling intervals against the resultant A2-H2 intervals or the A1-A2 intervals against the H1-H2 intervals. A typical dual-pathway curve is depicted in Figure 8-8. At longer coupling intervals, conduction proceeds over the fast (beta) pathway. At a coupling interval of 370 msec, a marked jump in the A-H interval is observed as the impulse blocks in the refractory beta pathway and proceeds slowly along the alpha pathway. Single A-V nodal echoes and SVT appear only during antegrade conduction down the slow pathway and retrograde conduction up the fast pathway. In approximately 25% of the cases, one does not see either an A-V nodal echo or SVT immediately after block in the fast pathway. In a smaller number of cases conduction proceeds down the fast pathway and the slow pathway producing a one-to-two response. An echo or SVT is observed only after a critical A-H interval is reached during slow-pathway conduction that allows the fast pathway to recover excitability and conduct retrogradely (Fig. 8-9). As seen in Figure 8-10, despite block in the fast pathway and very slow conduction down the slow pathway, AVNRT does not occur during high-right atrial stimulation. SVT is induced from the coronary sinus but only after a critical A-H in the slow pathway
is reached. Thus, relating these events to the proposed mechanism shown in Figure 8-1, one must conclude that typical nodal reentry is initiated by atrial stimulation producing block in the fast pathway with a jump to the slowly conducting pathway. Only when conduction is slow enough during slow-pathway conduction to allow recovery of the fast pathway does reentry occur.
is reached. Thus, relating these events to the proposed mechanism shown in Figure 8-1, one must conclude that typical nodal reentry is initiated by atrial stimulation producing block in the fast pathway with a jump to the slowly conducting pathway. Only when conduction is slow enough during slow-pathway conduction to allow recovery of the fast pathway does reentry occur.
By definition, in order to diagnose dual pathways in response to atrial extrastimuli, one must observe an increase of at least 50 msec in the A-H interval with a small (i.e., 10 msec) decrease in the coupling interval of the APD.15,16,38,49 Although the jump is usually in the order of 70 to 100 msec, marked increases in the A-H of several hundred milliseconds can be observed (Fig. 8-11). As mentioned, dual pathways may also be manifested by different P-R or A-H intervals during sinus rhythm or at identical paced rates (Fig. 8-3). A sudden jump in the A-H during atrial pacing may also be a manifestation of dual A-V pathways. We use a similar beat-to-beat change in the A-H interval of 50 msec during atrial pacing as a marker for dual pathways. Baker et al.51 suggested that another manifestation of dual A-V nodal pathways is the observation of atrial pacing-induced P-R interval exceeding the paced cycle length. This observation has been made only in patients with either overt dual pathways or AVNRT.
An uncommon manifestation of dual pathways is a double response to an atrial extrastimulus. In such an instance, an atrial extrastimulus can result in conduction down the fast pathway, and if conduction down the slow pathway is sufficiently prolonged, a second response to the ventricle can occur, which may be associated with an atrial echo or sustained AVNRT (Fig. 8-12). Occasionally, patients can exhibit spontaneous 1:2 conduction in sinus rhythm producing a nonreentrant tachycardia during sinus rhythm (Fig. 8-13). This situation requires slow enough conduction down the slow pathway that the HPS has time to recover to produce a second response. If conduction down the slow pathway is not long enough, block in the HPS will occur and the one-to-two response will be concealed (Fig. 8-14). Only a few such patients ever manifest spontaneous AVNRT, an example of which is shown in Figure 8-15. This reflects poor retrograde fast-pathway conduction and/or prolonged retrograde fast-pathway refractoriness, or both. In 11/12 cases I have studied AVNRT could be induced following isoproterenol and/or atropine (see Pharmacologic and Physiologic Maneuvers below).
The site of stimulation can affect the ability to initiate dual pathways and induce AVNRT. Although Ross et al.52 demonstrated that the site of stimulation may influence the A-H conduction times over the fast and slow pathways, they showed no significant alteration of refractoriness of these pathways or differences in the ease of induction of dual pathways or AVNRT. However, they noted variability of initiation from either site. Our experience differs slightly. We also have found that the conduction times over the fast and slow pathway may be different during high-right atrial and coronary sinus stimulation. The critical A-V nodal delay (i.e., A-H interval) required to initiate the tachycardia is different depending on the site of stimulation and is almost always shorter from the coronary sinus (Figs. 8-16 and 8-17).53 Whether or not these differences are due to true shortening of A-V nodal conduction time because of differential input into the AVN—as suggested by Batsford et al.54 and Aranda et al.55—or merely are due to apparent shortening of the A-H interval owing to the alteration of the relationship of the atrial electrogram in the His bundle recording and the true onset of A-V nodal depolarization is unclear. A shorter tachycardia cycle length associated with a shorter A-H at initiation would favor anisotropically based differences in conduction and refractoriness. Both of these mechanisms may play a role in individual patients.
In contrast to others, we have observed that dual A-V nodal pathways and reentry are easier to induce from the high-right atrium than from the coronary sinus, although in rare cases, induction of SVT can be accomplished only from the coronary sinus. The use of multiple drive cycle lengths, multiple extrastimuli, and rapid pacing make induction only by coronary sinus stimulation rare. Despite using these methods, we have observed induction of AVNRT by coronary sinus stimulation when high-right atrial stimulation failed in ∼3% of cases in which we performed both high-right atrial and coronary sinus stimulation (Fig. 8-18). We agree with Ross et al.52 that in most cases SVT can be induced with stimulation from both sites,
although different coupling intervals or paced cycle lengths may be required (Figs. 8-16 and 8-17). We have also observed that the refractory periods of the fast and slow pathways can differ depending on the site of stimulation. In approximately one-third of our patients, the refractory period of the fast pathway is shorter during coronary sinus stimulation than during high-right atrial stimulation (Figs. 8-10, 8-16, and 8-17). Moreover, as noted above, the critical A-H necessary to initiate AVNRT is greater during high-right atrial stimulation than for stimulation from the coronary sinus.53 This observation is compatible with the concept that the left atrial extension of the AVN is closer to the fast pathway, thus, stimulation from this site allows earlier recovery. Alternatively, it may just be an expression of a different, functionally determined fast pathway based on the site of stimulation. These site-dependent differences in the ability to initiate AVNRT, as well as differences in the refractory periods of the fast and slow pathways, are likely due to different inputs into the AVN with its nonuniform anisotropic nature. Although in some circumstances this may result from differences in the functional refractory periods (FRPs) of the atrium at the site of stimulation, the nonuniform anisotropic structure of the AVN is a more likely explanation. In any case, because of the variability of induction of AVNRT, it is important to stimulate from both the coronary sinus and the high-right atrium.
although different coupling intervals or paced cycle lengths may be required (Figs. 8-16 and 8-17). We have also observed that the refractory periods of the fast and slow pathways can differ depending on the site of stimulation. In approximately one-third of our patients, the refractory period of the fast pathway is shorter during coronary sinus stimulation than during high-right atrial stimulation (Figs. 8-10, 8-16, and 8-17). Moreover, as noted above, the critical A-H necessary to initiate AVNRT is greater during high-right atrial stimulation than for stimulation from the coronary sinus.53 This observation is compatible with the concept that the left atrial extension of the AVN is closer to the fast pathway, thus, stimulation from this site allows earlier recovery. Alternatively, it may just be an expression of a different, functionally determined fast pathway based on the site of stimulation. These site-dependent differences in the ability to initiate AVNRT, as well as differences in the refractory periods of the fast and slow pathways, are likely due to different inputs into the AVN with its nonuniform anisotropic nature. Although in some circumstances this may result from differences in the functional refractory periods (FRPs) of the atrium at the site of stimulation, the nonuniform anisotropic structure of the AVN is a more likely explanation. In any case, because of the variability of induction of AVNRT, it is important to stimulate from both the coronary sinus and the high-right atrium.
![]() FIGURE 8-15 A-V nodal reentry and nonreentrant tachycardia. This is the same patient as in Figure 8-13. The first four complexes are A-V nodal reentry. Retrograde block in the fast pathway terminates A-V nodal reentry, but sinus rhythm results in a nonreentrant tachycardia which is faster than the A-V nodal reentry. This is depicted in the ladder diagram. |
![]() FIGURE 8-17 Induction of A-V nodal reentry by coronary sinus (CS) stimulation. This figure is from the same patient as in Figure 8-16. In this figure, stimulation is carried out from the CS at a basic drive cycle length of 600 msec. A: At a coupling interval of 340 msec, the A-H is shorter than in Figure 8-16A during (HRA) stimulation. B: No jump in A-H intervals occurs at a coupling interval comparable to that in Figure 8-16B. C: Only at a coupling interval of 300 msec, a jump in the A-H interval occurs. However, here the A-H interval is only 220 msec, yet A-V nodal reentry is induced. Thus, despite the fact that a shorter coupling interval was required to initiate A-V nodal reentry from the CS, the critical A-H not only was shorter than the critical A-H in Figure 8-16C but was shorter than the A-H interval in (B) in which not even a single A-V nodal echo occurred. See text for discussion. |
If single APDs do not result in block in the fast pathway or, if block does occur, but does not result in sufficient A-V nodal delay, then repeated stimulation at shorter drive cycle lengths, which increase A-V nodal refractoriness and prolong conduction of both the fast and slow A-V nodal pathways (Fig. 8-19), or the use of double extrastimuli (Fig. 8-20) or atrial pacing (Fig. 8-6) frequently produce the block and delay required to initiate SVT. Most patients (approximately 85%) with typical A-V nodal reentrant SVT exhibit dual A-V nodal pathways in response to single high-right atrial extrastimuli.15,16,18,24,27,38 Using multiple drive cycle lengths and extrastimuli from both the high-right atrium and coronary sinus, as well as rapid atrial pacing, isoproterenol and/or atropine, dual pathways may be observed in 95% of patients with AVNRT. This is similar to the experience of Chen et al.56 Dual pathways in response to APDs may be observed in as many as 25% of patients without SVT. Despite the jump from fast to slow pathway, with very long A-H intervals, these patients never have an atrial echo; hence, one assumes that the major limitation is retrograde conduction over the fast pathway (Fig. 8-21). The determinants for AVNRT are discussed later in this chapter.
Multiple pathways may be observed in as many as 5% to 10% of patients. These are characterized by multiple jumps of >50 msec with increasingly premature atrial extrastimuli. This is comparable to the data of Tai et al.57 Such patients have AVNRTs with longer cycle lengths and longer ERPs and FRPs of the AVN. It is not uncommon for multiple AVNRTs with different cycle lengths and P–QRS relationships to be present. In general, the pathways with the longest conduction times are ablated more posteriorly in the triangle of Koch, leading some investigators to assert that these fibers are located more posteriorly.56,57 We have found several exceptions to this finding, and believe these findings may be ascribed to anisotropic propagation in a functionally determined circuit.
Failure to demonstrate antegrade dual pathways in a patient with typical A-V nodal reentrant SVT in response to atrial extrastimuli is usually due to one of three factors:
The refractory periods of the alpha and beta pathways may be similar, and more rapid pacing rates, the introduction of multiple atrial extrastimuli, or drugs such as beta blockers, calcium channel blockers, or digoxin may be required to dissociate them.58,59,60,61 An inadequate stimulation protocol is the most common cause for failure of atrial premature stimuli to demonstrate dual pathways. Typically, the use of multiple drive cycle lengths and/or multiple extrastimuli can obviate this problem. As noted, in rare cases, stimulation from one atrial site may be critical in inducing dual pathways and SVT when other sites cannot.
A long atrial FRP limits the prematurity with which APDs can reach the AVN, which renders the APD incapable of dissociating fast and slow pathways. This problem can be overcome by either performing stimulation at shorter drive cycle lengths, which decreases the FRP of the atrium, allowing shorter A1-A2 coupling intervals to be achieved and to reach the AVN; or by the introduction of double APDs, the first of which shortens atrial refractoriness, allowing the second to conduct to the AVN at an earlier point in time.
Block in the fast pathway has already occurred during the basal drive, and thus conduction always proceeds over the slow pathway. In such cases, SVT will develop in the absence of a jump in A-V nodal conduction time but will depend on achieving sufficiently prolonged conduction over the slow pathway to allow recovery of the fast pathway. As noted previously, the A-V nodal reentrant circuit may be reversed so that the fast pathway is used for antegrade conduction and the slow pathway is used for retrograde conduction (fast/slow, atypical, or uncommon form of AVNRT).18,24,25,26,27,28,62 The ECG in patients with this type of tachycardia exhibits a long R-P interval and a short P-R interval (Fig. 8-2). The nomenclature in this matter is confusing. While many investigators refer to fast/slow and slow/fast forms of AVNRT as signifying P-R and R-P relationships, others use these terms to relate to the site of earliest retrograde conduction (apex of triangle of Koch = “fast” and base = “slow”) in combination with whether antegrade conduction is fast or slow. I find this latter nomenclature too confusing and it implies that pathways are anatomic structures. Thus, I will refer to AVNRT subtypes based on P-R and R-P relationships. The ECG in patients with the uncommon (atypical) form of AVNRT exhibits a long R-P interval and a short P-R interval (Fig. 8-2). Patients characteristically do not have dual A-V nodal pathways in response to APDs or atrial pacing. The tachycardia is initiated with APDs or atrial pacing, producing modest increases of the A-H interval along the fast pathway (Figs. 8-22 and 8-23). Block in the slow pathway is concealed during antegrade stimulation because no jump occurs in A-H intervals. The only manifestation of block in the slow pathway is the development of an atrial echo with a long retrograde conduction time producing a long R-P short P-R tachycardia. This type of tachycardia must be distinguished from a posteroseptal concealed bypass tract (CBT) with A-V nodal-like properties.63,64,65,66
Programmed ventricular premature depolarizations (VPDs) during ventricular pacing or rapid ventricular pacing can also induce typical A-V nodal SVT, but these techniques are less effective than atrial stimulation and often require pharmacologic modulation of autonomic tone; that is, isoproterenol or atropine. In fact, initiation of typical AVNRT by a VPC delivered in NSR is extremely rare because His–Purkinje refractoriness prohibits an early VPC from reaching the AVN (see below). Rarely a late VPC can enter the AVN and produce concealed conduction so that an appropriately timed sinus beat can initiate AVNRT. In our experience, one or more forms of ventricular stimulation can initiate SVT in approximately 40% of patients with typical AVNRT. This is a higher incidence than that found by Sung et al.26 and Wu,24,66 who found ventricular stimulation–induced typical AVNRT in only 10% to 30% of patients. In contrast, atypical AVNRT is induced almost as frequently from the ventricle as from the atrium. In almost all of these patients, atrial stimulation can initiate SVT. Very uncommonly rapid ventricular pacing is the only method by which typical AVNRT can be initiated. Ventricular pacing is three times more effective than ventricular extrastimuli in initiating typical AVNRT (Figs. 8-24 and 8-25). If VPDs can initiate typical AVNRT, rapid ventricular pacing also can. In patients with typical AVNRT, retrograde conduction is usually very good and occurs over the (fast) beta pathway. Retrograde block to the atrium over the fast pathway rarely occurs in patients with typical AVNRT initiated by ventricular stimulation (Fig. 8-26), and when it occurs, suggests that the atrium is not required for AVNRT. In either case, ventricular stimulation must produce block in the slow pathway (concealed), conduction up the fast pathway, with subsequent recovery of the slow pathway in time to accept antegrade conduction over it to initiate the ventricular echo, and sustained tachycardia. Rapid ventricular pacing can induce concealed block in the slow pathway and SVT more easily than ventricular extrastimuli. With ventricular extrastimuli, the initial site of delay and/or block is in the His–Purkinje system. Even when conduction proceeds retrogradely over the His–Purkinje system, because of delay in the His–Purkinje system, the S1-H2 or V1-H2 remains constant. As a result, the prematurity with which the impulse reaches the AVN is fixed (see Chapter 2). Thus, induction of typical AVNRT by a single VPD during ventricular pacing only succeeds in approximately 10% of patients.
Specific reasons for failure to initiate typical AVNRT by VPDs include (a) block in the His–Purkinje system; (b) the
FRP of the His–Purkinje system exceeds the effective refractory period (ERP) of the slow pathway in the retrograde direction; (c) the ERP of the slow pathway is equal to that of the fast pathway in the retrograde direction; (d) the antegrade slow pathway ERP exceeds the ventricular-paced cycle length, therefore it cannot accept an input from the fast pathway at that cycle length; (e) following its engagement from the fast pathway, there is insufficient antegrade delay in the slow pathway to allow the fast pathway to recover; and (f) block in a lower final common pathway in the AVN (i.e., proximal to the slow and fast pathways in the retrograde direction), which thereby prohibits block in one of these pathways. Pacing at shorter drive cycle lengths may increase the percentage of patients in whom typical AVNRT can be induced by ventricular stimulation because of shortening the ERP and FRP of the His–Purkinje system.
FRP of the His–Purkinje system exceeds the effective refractory period (ERP) of the slow pathway in the retrograde direction; (c) the ERP of the slow pathway is equal to that of the fast pathway in the retrograde direction; (d) the antegrade slow pathway ERP exceeds the ventricular-paced cycle length, therefore it cannot accept an input from the fast pathway at that cycle length; (e) following its engagement from the fast pathway, there is insufficient antegrade delay in the slow pathway to allow the fast pathway to recover; and (f) block in a lower final common pathway in the AVN (i.e., proximal to the slow and fast pathways in the retrograde direction), which thereby prohibits block in one of these pathways. Pacing at shorter drive cycle lengths may increase the percentage of patients in whom typical AVNRT can be induced by ventricular stimulation because of shortening the ERP and FRP of the His–Purkinje system.
Many of the problems imposed by His–Purkinje refractoriness on induction of typical AVNRT by ventricular stimulation can be overcome by rapid ventricular pacing, during which the AVN is the primary site of delay. This explains why rapid pacing is more likely to longitudinally dissociate fast and slow pathways in the AVN than extrastimuli. The observation that ventricular pacing does not always induce typical A-V nodal SVT is explained in part by the occurrence of repetitive concealment, not block, in the slow pathway, rendering it incapable of antegrade conduction. Block cannot be distinguished from concealment in humans.
The specific mechanisms by which ventricular stimulation can induce A-V nodal reentrant SVT follows:
The most common method of inducing typical AVNRT depends on the retrograde refractory period of the alpha pathway exceeding that of the beta pathway. In this instance, VPDs or ventricular pacing induce typical AVNRT in the absence of the demonstration of retrograde dual pathways. Retrograde conduction proceeds up the fast pathway without prolonged retrograde conduction. Block in the slow pathway is not manifested (i.e., it is concealed) and is only inferred by initiation of the tachycardia. Thus, no critical V-A or H-A interval is required to induce the tachycardia. As mentioned, this is easier to achieve with ventricular pacing than ventricular extrastimuli. An example of the initiation of typical AVNRT by a VPD conducting up the fast pathway is shown in Figure 8-25. Initiation of a tachycardia by rapid ventricular pacing is shown in Figure 8-24.
Interpolated VPDs can result in the production of antegrade dual pathways; that is, retrograde concealed conduction can result in antegrade block in the fast beta pathway and allow slow conduction down the alpha pathway. This produces dual A-V nodal pathway physiology with induction of typical A-V nodal reentrant SVT. This is far less common than method 1.
If the retrograde refractory period of the fast pathway exceeds that of the slow pathway, VPDs or ventricular pacing can produce retrograde dual A-V nodal pathways. This
leads to the development of the uncommon type of A-V nodal SVT because initial block in the beta (fast) pathway results in a long retrograde conduction time up the alpha (slow) pathway, which is followed by antegrade conduction down the fast pathway. This is a common mechanism of induction of the atypical form of AVNRT in the laboratory. As noted, with atypical AVNRT, ventricular stimulation is equally as likely to initiate the rhythm as atrial stimulation. Occasionally, atypical AVNRT can be induced by ventricular extrastimuli demonstrating conduction over both the fast and slow pathways in response to the single ventricular extrastimulus (Fig. 8-27).42 In Figure 8-27, conduction of S2 occurs first over the fast, and then over the slow pathway. The H-A interval over the slow pathway at initiation of the tachycardia is much longer than the H-A interval during the tachycardia because of concealment into the slow pathway by the initial conduction over the fast pathway. This is analogous to initiation of AVNRT by atrial extrastimuli producing a one-to-two response (Fig. 8-12). Ventricular pacing, which readily demonstrates dual pathways during retrograde Wenckebach cycles, is another mode of initiation for this atypical form of AVNRT (Fig. 8-28).
Determinants for the Induction of Atrioventricular Nodal Reentry
Some generalizations can be made regarding the determinants for developing typical AVNRT. Although dual A-V nodal pathways are required for AVNRT, they are insufficient. Many patients can demonstrate “dual pathways” but do not have spontaneous or inducible AVNRT. The most common finding in patients with dual A-V nodal pathways who do not develop SVT is the failure of the impulse to return up the fast pathway once antegrade conduction has proceeded over the slow pathway. There are four possible explanations for this phenomenon: (a) longitudinal dissociation of the AVN is not really present, but the pattern of conduction of “pseudo-dual” pathways is produced by electrotonic propagation across a nonhomogenous linear area in the AVN, as described previously29; (b) the APD that “blocks” in the fast pathway produces postdepolarization refractoriness (i.e., a manifestation of concealed conduction) resulting in the inability of the fast pathway to recover excitability in time to be reexcited; conduction down the slow pathway is insufficiently slow to allow the fast pathway to recover; (c) the fast pathway has a long retrograde refractory period, which renders it incapable of retrograde conduction despite slow antegrade conduction of the slow pathway; (d) there is no distal connection between the fast and slow pathways. Based on our data and that of others67 the most common reason for failure to induce typical AVNRT in patients with antegrade dual pathways is that the fast pathway is incapable of rapid retrograde conduction.
This can be evaluated by using the maximum rates of 1:1 antegrade and retrograde conduction as indices of antegrade slow pathway refractoriness and retrograde fast pathway refractoriness. Denes et al.67 first analyzed fast and slow pathway properties in detail and clearly showed that the ability to induce typical AVNRT required 1:1 V-A conduction. Absence of induction of typical AVNRT in patients with antegrade dual pathways was associated with either no V-A conduction or V-A Wenckebach at relatively long drive cycle lengths.58 Most often, the ability to induce typical AVNRT requires the capability of one-to-one retrograde V-A conduction at paced ventricular cycle lengths of ≤400 msec.
Although retrograde fast-pathway characteristics determine if reentry can occur, slow-pathway conduction time determines when it will occur. Thus, the “critical A-H” concept depends on fast-pathway recovery at a given A2-H2 interval. When echoes do not occur as soon as the impulse blocks in the fast pathway and goes down the slow pathway, concealed conduction into the fast pathway by A2 may be present, requiring a critical A-H for recovery. This can mimic a primary impairment of V-A fast-pathway conduction as the determinant of reentry. One can recognize the likely presence of concealment if the A-H interval not producing an echo exceeds the shortest cycle length of 1:1 retrograde conduction up the fast pathway.
Although an isolated A-V nodal echo can occur as long as V-A conduction is present, the ability to initiate sustained SVT also requires the capability of the slow pathway to sustain repetitive antegrade conduction. Denes et al.67 found that sustained, typical AVNRT was initiated only if the slow pathway maintained 1:1 antegrade conduction at cycle lengths less than 350 msec. Patients who only had single A-V nodal echoes in response to APDs usually developed antegrade Wenckebach in the slow pathway at relatively long cycle lengths. Thus, the ability to initiate sustained SVT depends on the balance of a retrograde fast-pathway conduction and antegrade slow-pathway conduction.
Retrograde fast-pathway conduction appears to be the major determinant of the ability to initiate isolated A-V nodal echoes and/or sustained SVT. This is further supported by the fact that there is no difference in maximal A-H intervals during slow-pathway conduction in patients with and without echoes or SVT. The ability to initiate A-V nodal echoes by APDs thus depends primarily on the capability of V-A conduction over the fast
pathway. This has been our experience almost universally. It is therefore obvious that patients with retrograde dual pathways will have a low incidence of sustained typical AVNRT, because the presence of dual pathways suggests relatively poor retrograde conduction over the fast pathway. Thus, even if 1:1 V-A conduction is present at cycle lengths of less than 400 msec, AVNRT usually occurs only in patients in whom retrograde conduction is maintained over the fast pathway. In an analysis of retrograde dual A-V nodal pathways, Strasberg et al.68 found that they could induce typical AVNRT in approximately 20% of patients presenting with retrograde dual A-V nodal pathways. In those cases, retrograde dual pathways (i.e., block in the retrograde fast pathway) occurred at relatively short coupling intervals or short-paced cycle lengths. In our experience, only 5% of patients with retrograde dual A-V nodal pathways will develop typical AVNRT in the baseline state. We have found the use of atropine or isoproterenol, which shortens the retrograde refractory period of the fast pathway and improve retrograde fast-pathway conduction, increases the number of patients with retrograde dual pathways in the control state in whom sustained typical AVNRT can be induced by APDs to 20%. Others have found a slightly higher incidence.67,68,69,70 In our patients, facilitation of induction of typical AVNRT depended on improving V-A conduction such that 1:1 V-A conduction over the fast pathway at cycle lengths of 425 msec or less was achieved in all patients in whom typical AVNRT could be induced. Thus, the markers that are predictive of a low likelihood of inducibility of typical AVNRT are (a) no V-A conduction, (b) V-A conduction worse than A-V conduction with retrograde Wenckebach at long cycle lengths (500 msec or more), and (c) retrograde dual pathways. Exceptions can occur if failure of V-A conduction results from retrograde block in the HPS or in a lower final common pathway in the AVN (see subsequent discussion). In the latter case, catecholamines and/or atropine can overcome the block. In addition, as noted earlier in the chapter (and to be discussed later) A-V nodal tachycardia can occur with block to the atrium; retrograde fast pathway is present but not manifested by atrial activation.
pathway. This has been our experience almost universally. It is therefore obvious that patients with retrograde dual pathways will have a low incidence of sustained typical AVNRT, because the presence of dual pathways suggests relatively poor retrograde conduction over the fast pathway. Thus, even if 1:1 V-A conduction is present at cycle lengths of less than 400 msec, AVNRT usually occurs only in patients in whom retrograde conduction is maintained over the fast pathway. In an analysis of retrograde dual A-V nodal pathways, Strasberg et al.68 found that they could induce typical AVNRT in approximately 20% of patients presenting with retrograde dual A-V nodal pathways. In those cases, retrograde dual pathways (i.e., block in the retrograde fast pathway) occurred at relatively short coupling intervals or short-paced cycle lengths. In our experience, only 5% of patients with retrograde dual A-V nodal pathways will develop typical AVNRT in the baseline state. We have found the use of atropine or isoproterenol, which shortens the retrograde refractory period of the fast pathway and improve retrograde fast-pathway conduction, increases the number of patients with retrograde dual pathways in the control state in whom sustained typical AVNRT can be induced by APDs to 20%. Others have found a slightly higher incidence.67,68,69,70 In our patients, facilitation of induction of typical AVNRT depended on improving V-A conduction such that 1:1 V-A conduction over the fast pathway at cycle lengths of 425 msec or less was achieved in all patients in whom typical AVNRT could be induced. Thus, the markers that are predictive of a low likelihood of inducibility of typical AVNRT are (a) no V-A conduction, (b) V-A conduction worse than A-V conduction with retrograde Wenckebach at long cycle lengths (500 msec or more), and (c) retrograde dual pathways. Exceptions can occur if failure of V-A conduction results from retrograde block in the HPS or in a lower final common pathway in the AVN (see subsequent discussion). In the latter case, catecholamines and/or atropine can overcome the block. In addition, as noted earlier in the chapter (and to be discussed later) A-V nodal tachycardia can occur with block to the atrium; retrograde fast pathway is present but not manifested by atrial activation.
The A-H interval may be a useful marker in predicting the capability of rapid V-A conduction. In our experience, as well as that of Bauernfeind et al.,71 the shorter the A-H interval, the better the V-A conduction. In fact, the shorter the A-H interval, the shorter the antegrade refractory period of the fast pathway, the shorter the cycle length at which block is produced in the fast pathway, and the shorter the cycle length at which 1:1 V-A conduction is maintained. In addition, although overlap existed, Bauernfeind et al.71 found statistically significant relationships between retrograde H-A intervals and antegrade A-H intervals, shorter cycle lengths maintaining 1:1 V-A conduction and A-H interval, and a higher incidence of inducible SVT in patients with short A-H intervals and dual pathways than in those with longer A-H intervals and dual pathways.64 These findings provide a potential explanation for the high incidence of SVT in patients with short P-R intervals giving rise to the so-called Lown–Ganong–Levine syndrome. It is of interest that Akhtar18 also found that the shortest cycle length of typical A-V nodal SVT correlated best with the shortest cycle length of 1:1 V-A conduction, a finding that correlates with a short A-H interval. In contrast, we have found that the cycle length of SVT is more closely related to conduction down the slow pathway than the A-H interval in sinus rhythm or the H-A during SVT.72 Although in patients more than 45 years old the H-A interval and cycle lengths are longer than in patients less than 45, the absolute duration of the A-H is more closely related to the AVNRT cycle length than to the H-A interval.72 Spontaneous or pharmacologic-mediated changes in SVT cycle lengths are also more closely associated with changes in slow-pathway conduction. Our findings, which appear to contradict those of Akhtar,18 grew out of a study examining a different aspect of AVNRT than Akhtar’s study. He was trying to determine the limiting factor for tachycardia rate while we were analyzing factors controlling the tachycardia rate. Spontaneous termination of typical AVNRT can occur by either retrograde block in the fast pathway or antegrade block in the slow pathway. Although it is not always possible to predict which pathway will be the major limiting factor in maintaining AVNRT in an individual patient, a short P-R interval suggests that the fast pathway is unlikely to be the site of block. In addition, the direction (i.e., A-V or V-A) at which conduction fails at the longest cycle length provides a reasonable, but not absolute, marker for induction of spontaneous block.
Little data exist for the determinants of induction of atypical AVNRT because it is such an uncommon arrhythmia. It is our impression that retrograde slow-pathway conduction is the major determinant inducing this arrhythmia. Antegrade fast-pathway conduction is usually rapid enough and refractoriness is short enough to accept and conduct antegradely the impulse that has conducted retrogradely over the slow pathway. Thus, the development of atypical AVNRT by ventricular stimulation is determined primarily by the ability to demonstrate retrograde slow-pathway conduction, since the fast pathway almost always can return the impulse toward the ventricle. When the uncommon form of AVNRT is induced by atrial stimulation, the major determinant of retrograde slow-pathway conduction is insufficient antegrade concealment into the slow pathway so that retrograde conduction is possible.
Atrial Activation Sequence and the P–QRS Relationship During Supraventricular Tachycardia
The sequence of retrograde atrial activation during typical A-V nodal reentrant tachycardia is more complex than previously believed. While early studies suggested that the initial site of retrograde atrial activation during typical AVNRT is recorded in the His bundle electrogram,16,18,73,74,75 more detailed mapping using a decapolar catheter with 2-mm interelectrode distance to record the His bundle (distal pair) and electrograms along the tendon of Todaro, a deflectable quadripolar catheter (2-5-2–mm interelectrode distance) at the base of the triangle of Koch (area between the os of the coronary sinus and tricuspid annulus), and a decapolar catheter (5-mm interelectrode distance) in the coronary sinus with the proximal pair placed 1 to 2 cm inside the os
(Fig. 8-29).76,77 of the triangle of Koch and coronary sinus shows marked heterogeneity in the pattern of activation.76,77 Patterns were described along the tendon of Todaro as sequential or broad and throughout the triangle of Koch and coronary sinus as having single or multiple breakthrough sites (Fig. 8-30). Multiple breakthroughs were considered to be present when two or more activation times along the His bundle catheter within 5 msec of each other were separated by two later sites, or when one or more sites on the His bundle catheter and any other catheter (i.e., slow pathway or coronary sinus) occurred within 5 msec of each other. VPDs were introduced when necessary to accurately measure the atrial activation sequence. Examples of heterogenous atrial activation during AVNRT are shown in Figures 8-31 and 8-32 In nearly 50% of patients a secondary breakthrough in the coronary sinus was observed (Fig. 8-29); that is, an electrogram within the coronary sinus was bounded by sites with later electrograms.78 Similar findings were observed, but not commented upon by McGuire et al.79 Activation during AVNRT and ventricular pacing were discordant qualitatively and/or quantitatively in 60% of patients (Fig. 8-33). Similar heterogeneity was seen during atypical AVNRT although earliest activation tended to be at the base of the triangle of Koch or in the coronary sinus (Fig. 8-34).76,77,78 Multiple breakthroughs, during detailed atrial mapping, are a useful discriminator of AVNRT from atrial tachycardia or circus movement tachycardia using a bypass tract. Although Jackman’s group have described and depicted a number of large reentrant pathways involving the left atrium and coronary sinus, our mapping data have never found and do not support a macroreentrant circuit. The atrial activation pattern during AVNRT has important implications as to the role of the atrium in AVNRT (see discussion below). From these heterogenous activation patterns, the wave of atrial activation subsequently spreads cephalad and laterally to depolarize the remainder of the right and left atria. These findings suggest that retrograde activation during SVT spreads radially after leaving the AVN. Of note, studies by Ross et al.,80 using intraoperative mapping, demonstrated a relationship of the earliest site of atrial activation to the retrograde H-A interval. In general, the shorter the H-A interval, the more likely the earliest atrial activation is recorded in the His bundle electrograms. As the H-A interval prolongs, the earliest activation moves closer to the base of the triangle of Koch or in the coronary sinus. Of importance is the recognition that identification of an “earliest” site of atrial activation does not mean that atrial activation is sequential from that site. As stated above, multiple breakthroughs are present in ∼50% of patients. Recent experience using left atrial mapping in addition to detailed right atrial and coronary sinus mapping has confirmed the lack of sequential activation compatible with large reentrant circuits during AVNRT.
(Fig. 8-29).76,77 of the triangle of Koch and coronary sinus shows marked heterogeneity in the pattern of activation.76,77 Patterns were described along the tendon of Todaro as sequential or broad and throughout the triangle of Koch and coronary sinus as having single or multiple breakthrough sites (Fig. 8-30). Multiple breakthroughs were considered to be present when two or more activation times along the His bundle catheter within 5 msec of each other were separated by two later sites, or when one or more sites on the His bundle catheter and any other catheter (i.e., slow pathway or coronary sinus) occurred within 5 msec of each other. VPDs were introduced when necessary to accurately measure the atrial activation sequence. Examples of heterogenous atrial activation during AVNRT are shown in Figures 8-31 and 8-32 In nearly 50% of patients a secondary breakthrough in the coronary sinus was observed (Fig. 8-29); that is, an electrogram within the coronary sinus was bounded by sites with later electrograms.78 Similar findings were observed, but not commented upon by McGuire et al.79 Activation during AVNRT and ventricular pacing were discordant qualitatively and/or quantitatively in 60% of patients (Fig. 8-33). Similar heterogeneity was seen during atypical AVNRT although earliest activation tended to be at the base of the triangle of Koch or in the coronary sinus (Fig. 8-34).76,77,78 Multiple breakthroughs, during detailed atrial mapping, are a useful discriminator of AVNRT from atrial tachycardia or circus movement tachycardia using a bypass tract. Although Jackman’s group have described and depicted a number of large reentrant pathways involving the left atrium and coronary sinus, our mapping data have never found and do not support a macroreentrant circuit. The atrial activation pattern during AVNRT has important implications as to the role of the atrium in AVNRT (see discussion below). From these heterogenous activation patterns, the wave of atrial activation subsequently spreads cephalad and laterally to depolarize the remainder of the right and left atria. These findings suggest that retrograde activation during SVT spreads radially after leaving the AVN. Of note, studies by Ross et al.,80 using intraoperative mapping, demonstrated a relationship of the earliest site of atrial activation to the retrograde H-A interval. In general, the shorter the H-A interval, the more likely the earliest atrial activation is recorded in the His bundle electrograms. As the H-A interval prolongs, the earliest activation moves closer to the base of the triangle of Koch or in the coronary sinus. Of importance is the recognition that identification of an “earliest” site of atrial activation does not mean that atrial activation is sequential from that site. As stated above, multiple breakthroughs are present in ∼50% of patients. Recent experience using left atrial mapping in addition to detailed right atrial and coronary sinus mapping has confirmed the lack of sequential activation compatible with large reentrant circuits during AVNRT.
On the surface ECG the narrowest P waves are seen when atrial activation begins at the apex of the triangle of Koch. In our experience 7% of AVNRTs have earliest atrial activation
recorded in the coronary sinus, suggesting atrial activation via the left atrial extension of the AVN (Figs. 8-35 and 8-36) All of these patients were successfully ablated from the right side suggesting that the left atrial activation is an epiphenom. Others81,82 have shown a high variability of the earliest site of activation in all varieties of AVNRT which further makes terms like fast-slow; slow-fast, or slow-slow dependent on the anatomic site of retrograde conduction and the R-P relationship, meaningless.
recorded in the coronary sinus, suggesting atrial activation via the left atrial extension of the AVN (Figs. 8-35 and 8-36) All of these patients were successfully ablated from the right side suggesting that the left atrial activation is an epiphenom. Others81,82 have shown a high variability of the earliest site of activation in all varieties of AVNRT which further makes terms like fast-slow; slow-fast, or slow-slow dependent on the anatomic site of retrograde conduction and the R-P relationship, meaningless.
The relationship of atrial activation to the QRS complex is extremely important from a diagnostic standpoint. In approximately 70% of the cases of typical A-V nodal reentrant SVT, the onset of atrial activation (i.e., earliest intracardiac atrial electrogram) appears before or at the onset of the QRS complex. The P wave sometimes begins so early that it gives the appearance of a Q wave in the inferior leads. This is most likely to occur when there is delay between the reentrant circuit and the ventricles. This is most frequent when there is H-V prolongation (Fig. 8-37) but may be observed with delay in the AVN distal to the circuit (Fig. 8-38). In the former case retrograde activation takes place during the H-V interval, and in the latter case retrograde atrial activation begins before the His deflection. This “pseudo-Q wave” is a rare, but relatively specific finding for AVNRT. The presence of atrial activation appearing at or immediately before the onset of the QRS during SVT excludes the possibility of a concealed atrioventricular bypass tract (see following discussion of concealed bypass tracts). The rapid retrograde atrial activation occurs because, as discussed previously, the retrograde limb of the reentrant circuit is the fast beta pathway. In approximately 25% of the tachycardias, atrial activation begins just within the QRS. In 95% of patients, either no discrete P waves are noted on the surface electrocardiogram (40%), or the terminal part of the QRS is slightly distorted, possibly appearing as a “pseudo-S wave” in the inferior leads, a “pseudo-R’” in V1, or a nonspecific terminal notching (57%). In the remaining patients, the P wave may be seen just after the end of the QRS. Intra-atrial conduction delays can alter the duration of atrial activation so that completion of atrial activation occurs slightly after termination of the QRS. In those patients in whom the P wave distorts the terminal QRS or appears at the end of the QRS, one must distinguish this tachycardia from the circus movement tachycardias using CBTs (see following discussion of concealed bypass tracts). Because completion of atrial activation arising in the midline usually requires at least 50 msec, the appearance of atrial activity as a terminal notch in the QRS, representing the end part of the P wave, suggests that atrial activation begins within the QRS and makes a bypass tract less likely. Sometimes, however, this is hard to distinguish from a bypass tract. In such cases atrial mapping as well as a variety of different responses to ventricular stimulation can help determine whether a bypass tract or atrial tachycardia is present (see following discussion of concealed bypass tracts and atrial tachycardia).
In patients who have the atypical form of AVNRT, the R-P interval is long because retrograde conduction proceeds over the slow pathway. Thus, the R-P/P-R ratio is greater than 1 (long R-P tachycardia). This pattern is extremely uncommon, representing perhaps only 5% of the instances of spontaneous sustained AVNRT in the absence of a prior ablation. The atypical form of AVNRT is often confused with an incessant form of tachycardia caused by a slowly conducting, concealed posteroseptal atrioventricular bypass tract63,64,65 or an atrial tachycardia arising in the low intra-atrial septum. Atypical AVNRT is more often a paroxysmal arrhythmia, while atrial tachycardias
or those using slowly conducting bypass tracts are frequently incessant and difficult to treat methods to distinguish these arrhythmias are discussed later in this chapter. The R-P intervals discussed above are in the absence of prior catheter ablation. We have noted a greater incidence of atypical R-P relationships and multiple tachycardias in patients postablation.
or those using slowly conducting bypass tracts are frequently incessant and difficult to treat methods to distinguish these arrhythmias are discussed later in this chapter. The R-P intervals discussed above are in the absence of prior catheter ablation. We have noted a greater incidence of atypical R-P relationships and multiple tachycardias in patients postablation.
![]() FIGURE 8-32 Examples of single and multiple breakthroughs of atrial activation during A-V nodal reentry. A: Shows a single breakthrough with a broad wave of activation along the HBE. The figure is organized similar to Figure 8-31 with one additional SP recording and five CS recordings. Note that earliest activation occurs along the HBE, at which there is near simultaneous activation of all five poles (broad pattern), following which the rest of the triangle of Koch is activated. B: Shows an example of multiple breakthroughs and a broad wavefront of activation along the HBE. The figure is organized similar to the left panel. AVNRT with an LBBB is present. Atrial activation is easily seen because of the long H-V interval. Note that atrial activation begins simultaneously along the HBE (poles HBEd, 2, 3 are nearly simultaneous, i.e., broad pattern) and at the SPd. Thus the apex and base of the triangle of Koch demonstrate multiple breakthroughs. A Carto map showing multiple breakthroughs is shown in C. See text for further discussion. |
![]() FIGURE 8-33 Discordant atrial activation between ventricular pacing and typical AVNRT. The figure is organized as in Figure 8-29. On the left during typical AVNRT 2 VPDs expose atrial activation, which demonstrates a single breakthrough at the HBE with a broad pattern of HBE activation. During ventricular pacing on the right, atrial activation is single and sequential. The difference in activation is readily apparent. See text for discussion. |
![]() FIGURE 8-35 Typical AVNRT with earliest atrial activation in the CS. This figure is organized similar to Figure 8-31. Typical AVNRT with a RBBB is present. Earliest atrial activation is noted at CS3. See text for discussion. |
Effect of Bundle Branch Block During Atrioventricular Nodal Reentrant Supraventricular Tachycardia
Bundle branch block is produced by alterations in His–Purkinje conduction and/or refractoriness and therefore should not modify a reentrant process localized to the AVN. If the turnaround site of the reentrant circuit incorporated one of the bundle branches, then bundle branch block or impaired conduction in that proximal bundle branch could alter the tachycardia. The development of prolonged aberration during AVNRT is very uncommon. Of the last 900 consecutively studied patients with A-V nodal reentrant SVT, only 43 developed sustained bundle branch block following induction by APDs; 25 of these had right bundle branch block, and 18 had left bundle branch block. An additional 85 developed ≥5 consecutive complexes manifesting bundle branch block either following induction by ventricular stimulation (19 patients) or, more commonly, following resumption of 1:1 conduction to the ventricles after a period of block below the tachycardia circuit (66/103 patients; see following discussion entitled “Requirement of the Atrium and Ventricle”). All 103 of the patients manifesting block below the tachycardia circuit manifested transient aberrant complexes before normalization of the QRS. In no patient was the AVNRT cycle length (A-A) or H-H interval influenced by the appearance of bundle branch
block. At the onset of bundle branch block, if the H-V interval is prolonged, an increase in the V-V interval equal to the increment in the H-V interval can occur; however, the following V-V time would be shortened by the same amount. During this transition, the A-A and H-H intervals generally remain constant, although if aberration occurs at the onset of the tachycardia, some oscillation of all electrograms can be observed, with some slight slowing prior to resumption of 1:1 conduction. Thus, it is safest to analyze the effects of bundle branch block after induction of the arrhythmia when the tachycardia is stabilized. During the tachycardia, the introduction of VPDs also may occasionally produce transient bundle branch block
(usually left bundle branch block) by retrograde concealment into the bundle branches and/or by rendering the local ventricular myocardium refractory to antegrade depolarization. As in the spontaneously observed appearance of bundle branch block, no change in the A-A or H-H interval results. Whenever the H-V interval prolongs with the development of bundle branch block, the V-V interval also prolongs for one cycle, as mentioned. In this instance, however, because the circuit is not influenced, the A-A interval remains constant and the atrial electrogram, and even the P wave, can be seen before the QRS. The lack of influence of bundle branch block on A-V nodal reentrant SVT is in contrast to patients with SVTs incorporating free-wall bypass tracts (see following discussion). An example of the failure of bundle branch block to influence the tachycardia cycle length is shown in Figure 8-38. In this instance, after a period of left bundle branch block, A-V conduction normalizes. No change in the A-A or V-V intervals occurs during the stable portion of each pattern of ventricular activation.
block. At the onset of bundle branch block, if the H-V interval is prolonged, an increase in the V-V interval equal to the increment in the H-V interval can occur; however, the following V-V time would be shortened by the same amount. During this transition, the A-A and H-H intervals generally remain constant, although if aberration occurs at the onset of the tachycardia, some oscillation of all electrograms can be observed, with some slight slowing prior to resumption of 1:1 conduction. Thus, it is safest to analyze the effects of bundle branch block after induction of the arrhythmia when the tachycardia is stabilized. During the tachycardia, the introduction of VPDs also may occasionally produce transient bundle branch block
(usually left bundle branch block) by retrograde concealment into the bundle branches and/or by rendering the local ventricular myocardium refractory to antegrade depolarization. As in the spontaneously observed appearance of bundle branch block, no change in the A-A or H-H interval results. Whenever the H-V interval prolongs with the development of bundle branch block, the V-V interval also prolongs for one cycle, as mentioned. In this instance, however, because the circuit is not influenced, the A-A interval remains constant and the atrial electrogram, and even the P wave, can be seen before the QRS. The lack of influence of bundle branch block on A-V nodal reentrant SVT is in contrast to patients with SVTs incorporating free-wall bypass tracts (see following discussion). An example of the failure of bundle branch block to influence the tachycardia cycle length is shown in Figure 8-38. In this instance, after a period of left bundle branch block, A-V conduction normalizes. No change in the A-A or V-V intervals occurs during the stable portion of each pattern of ventricular activation.
![]() FIGURE 8-36 Atypical AVNRT with earliest atrial activation in the CS. Atypical AVNRT is present. Earliest atrial activation occurs at CS 3 (arrow). See text for further discussion. |
![]() FIGURE 8-37 Atrial activation beginning prior to the QRS producing a pseudo-Q wave during typical AVNRT. The figure is organized similar to Figure 8-31. Typical AVNRT with an LBBB is present. The H-V interval is prolonged (75 msec) so that atrial activation begins well before the QRS giving rise to a pseudo-Q wave in lead 2. See text for discussion. |
![]() FIGURE 8-38 The lack of effect of bundle branch block on (A-V) nodal reentry. (The figure is arranged as Figure 8-24.) A-V nodal reentry is present at a cycle length of 315 msec. In the left half of the panel, left bundle branch block is present, and on the right, the QRS is normalized. Between the transition is a complex of intermittent configuration. Left bundle branch block does not alter the atrial or ventricular cycle lengths of A-V nodal reentry. The loss of left bundle branch block via a transitional complex suggests it is due to phase 3 block. Note the normal retrograde activation sequence being earliest in the HBE with subsequent spread to the CS, HRA, and posterior low-right atrium (PLRA). |
Requirement of the Atrium and Ventricle
One of the issues that remains controversial is whether or not the reentrant circuit in AVNRT is entirely subatrial or even intranodal, including “upper and lower” final common pathways, or whether some component of the atrium or His bundle is required (Fig. 8-39). The presence of 1:1 conduction at rapid rates with a short V-A interval, a short H-A interval that remains relatively fixed during SVT, and results of surgical interventions suggest to some that the reentrant circuit involves tissue above and below the AVN.80,83 Results of catheter modification of the AVN have also been interpreted similarly (see Chapter 15). Successful ablation of the “fast” pathway at the apex and “slow” pathway at the base of the triangle of Koch has suggested an anatomic construct in which the impulse goes from the fast pathway to the septal atrium, followed by sequential atrial activation to the slow pathway through which the AVN is engaged. Pathologic specimens demonstrating radiofrequency-induced lesions not involving the compact node (no known extent of physiologic influences)
have been used to further support this construct. Still other investigators have taken the relative ease with which AVNRT can be reset from the base of the triangle of Koch as evidence of atrial participation in the circuit.46 I believe all of these findings do not prove the requirement of the atrium, but only suggest that the circuit has dimension and/or has different inputs and outputs that are determined by the anisotropic nature of the compact node, its extensions, and the transitional fibers that can be more or less easily engaged depending on the site of stimulation.
have been used to further support this construct. Still other investigators have taken the relative ease with which AVNRT can be reset from the base of the triangle of Koch as evidence of atrial participation in the circuit.46 I believe all of these findings do not prove the requirement of the atrium, but only suggest that the circuit has dimension and/or has different inputs and outputs that are determined by the anisotropic nature of the compact node, its extensions, and the transitional fibers that can be more or less easily engaged depending on the site of stimulation.
I believe that the bulk of the evidence, both experimentally and clinically, suggests that, in most patients, AVNRT is a subatrial reentrant circuit based on the anisotropic properties of the AVN and transitional tissue.41,77,78,84,85,86,87,88,89,90,91,92 As stated earlier, Efimov et al. using high-density voltage-sensitive dye mapped the AVN three dimensionally and found reentry was subatrial, involving the AVN +/− transitional cells. Cell-to-cell coupling in the AVN is extremely poor due to heterogenous connexins, both type and distribution (primarily connexin 45, with small amounts of connexin 40 near the His bundle) which is the main reason the node is so slowly conducting.
Clinical data, which come from observations during spontaneous SVT, at the time of induction of SVT, and response to stimulation during SVT are discussed in the following paragraphs and are summarized in Table 8-3. They include (a) initiation of AVNRT in the absence of an atrial echo; (b) maintenance of AVNRT in the presence of either a changing V-A relationship, 2:1 retrograde or V-A block and/or A-V dissociation; (c) depolarization of the atrium surrounding the AVN without influencing the tachycardia; (d) resetting AVNRT without atrial activation; (e) heterogenous atrial activation during AVNRT incompatible with atrial participation; and (f) atrial pacing and entrainment of AVNRT with a longer A-H than during the tachycardia entrainment suggesting that little if any of the atrium is required.
TABLE 8-3 AVNRT: Evidence That the Atrium is not Necessary | |
---|---|
|
Requirement of the Atrium in AVNRT
Initiation of AVNRT in the absence of atrial activation most frequently occurs during ventricular stimulation (Fig. 8-40). In virtually all of these cases the initial retrograde H (if seen) to antegrade H interval was longer than the subsequent H-H intervals, and the tachycardia cycle lengths were relatively long (i.e., >400 msec). As ventricular coupling intervals were decreased, the initial retrograde H (when observed) to antegrade H interval increased. While absence of atrial activation at the initiation of AVNRT is an uncommon observation, it will be missed if ventricular stimulation is not routinely performed. Rarely, retrograde block in the fast pathway can be observed at initiation of AVNRT by atrial stimulation or by induction during atrial flutter or fibrillation. The preceding findings were made only during typical AVNRT. The occurrence of the ventricular echoes during atrial fibrillation, a well-recognized phenomenon, suggests that the atrium is also not required for atypical AVNRT.
Retrograde block to the atrium during AVNRT is uncommon (96/1,798 of our cases) but has been reported.78,85,86,87,88,90,93 In my experience it only occurs in typical AVNRT. AVNRT with 2:1 block to the atrium with resumption of 1:1 conduction and acceleration of the AVNRT following atropine is shown in Figure 8-41. Examples of AVNRT with both 2:1 block and Wenckebach to the atrium and AVNRT with A-V dissociation and intermittent capture are shown in Figure 8-42 (top and bottom, respectively). Adenosine-induced V-A block and A-V dissociation during AVNRT is shown in Figure 8-43. Whenever A-V dissociation occurs with apparent AVNRT, one must rule out the presence of a concealed nodofascicular or nodoventricular bypass tract as the retrograde limb. These extremely rare pathways are suggested by the influence of bundle branch block on the tachycardia cycle length or the ability to reset or terminate the SVT with ventricular extrastimuli or pacing delivered when the His bundle is refractory. An example of AVNRT with A-V dissociation unaffected by the appearance of RBBB is shown in Figure 8-44.
Two other related phenomena support lack of atrial requirement for AVNRT. First is the very common observation at the onset of AVNRT of changing V-A relationships with minimal or no change in tachycardia cycle length. This suggests that atrial activation is determined by the functional output to the atrium from the tachycardia, and not causally related to it. The finding of multiple tachycardias with identical retrograde atrial activation sequences and cycle lengths but different V-A relationships further supports the hypothesis that atrial activation is an epiphenomenon of AVNRT and is functionally determined by nodoatrial coupling and the anisotropic nature of the subatrial nodal structures (Fig. 8-45).
Responses to Stimulation During AVNRT—Role in Defining Atrial and Subnodal Participation
During A-V nodal reentrant SVT, APDs can produce several responses (Fig. 8-46). Relatively late APDs fail to alter the cycle length of SVT; therefore, full compensatory pauses result (Fig. 8-45A) because of the inability of the atrial stimulus to depolarize the entire atria to reach the AVN or, if the atria are depolarized, failure to penetrate the reentrant circuit. The ability to depolarize the atria, specifically, the atrial tissue surrounding AVN (i.e., the atrium recorded at the His bundle electrogram and at the os and within the coronary sinus), without affecting the tachycardia provides evidence that the atria are not a necessary link in the reentrant circuit (Fig. 8-47). Demonstration of premature excitation of all the recordable atrial tissue around the AVN is imperative (i.e., the atrial electrograms at the os and within the CS, and the HBE), because these are the areas where earliest activity is recorded during mapping of SVT in the laboratory24,25,26,77,78 or intraoperatively.90 Because a noncompensatory pause may reflect delay in the slow pathway, which exactly compensates for the prematurity of the atrial extrastimulus (an unlikely but theoretically possible event), the investigation should attempt to demonstrate this response over a range of coupling intervals of ≥30 msec to ensure that the APD has not entered the node and fortuitously increased the A-H interval to produce a fully compensatory pause. If possible, stimulation should be performed from both the high-right atrium and os of the coronary sinus because stimulation at both those sites yields different A-H intervals, as mentioned earlier. If the same fully compensatory response occurs from stimulation at both sites, it is unlikely that compensatory A-H delay is responsible. This is clearly demonstrated in
Figure 8-46. Simultaneous stimulation from the right atrium and coronary sinus can yield the same result (Fig. 8-48). The same phenomenon can occasionally be seen using double extrastimuli (Fig. 8-49), a situation that makes compensatory A-V nodal delays even more unlikely. The demonstration of this phenomenon may even be observed during atrial pacing or atrial fibrillation or flutter. This can be seen in Figure 8-50 in which the first two beats of AVNRT are unaffected by atrial flutter/fibrillation. The third QRS is advanced by ≈20 msec and AVNRT resumes without requiring an atrial echo (see prior discussion). It is important for the investigator to recognize that recordings from the os and within the coronary sinus as well as the His bundle electrogram are necessary to demonstrate local atrial capture around the AVN. The demonstration of a full compensatory pause after the introduction of an APD does not prove the atrium is unnecessary unless it can be shown that the atrial tissue surrounding the AVN is captured before it would have been depolarized by spontaneously occurring atrial echoes without altering the arrhythmia. Although, theoretically, a protected, nonrecordable bridge of atrial tissue could be involved in the circuit, entrance block to the area would be required to prevent its depolarization by APDs, and exit block would be required to prevent recording an atrial electrogram. Since the atria are normal in the vast majority of patients with AVNRT, and the APDs are delivered at intervals in excess of local atrial refractory periods, this is hardly conceivable.
Figure 8-46. Simultaneous stimulation from the right atrium and coronary sinus can yield the same result (Fig. 8-48). The same phenomenon can occasionally be seen using double extrastimuli (Fig. 8-49), a situation that makes compensatory A-V nodal delays even more unlikely. The demonstration of this phenomenon may even be observed during atrial pacing or atrial fibrillation or flutter. This can be seen in Figure 8-50 in which the first two beats of AVNRT are unaffected by atrial flutter/fibrillation. The third QRS is advanced by ≈20 msec and AVNRT resumes without requiring an atrial echo (see prior discussion). It is important for the investigator to recognize that recordings from the os and within the coronary sinus as well as the His bundle electrogram are necessary to demonstrate local atrial capture around the AVN. The demonstration of a full compensatory pause after the introduction of an APD does not prove the atrium is unnecessary unless it can be shown that the atrial tissue surrounding the AVN is captured before it would have been depolarized by spontaneously occurring atrial echoes without altering the arrhythmia. Although, theoretically, a protected, nonrecordable bridge of atrial tissue could be involved in the circuit, entrance block to the area would be required to prevent its depolarization by APDs, and exit block would be required to prevent recording an atrial electrogram. Since the atria are normal in the vast majority of patients with AVNRT, and the APDs are delivered at intervals in excess of local atrial refractory periods, this is hardly conceivable.
Another observation suggesting that the “upper common pathway” is located within the node (or at least, subatrial transitional zone) is the ability of an APD to retard the subsequent atrial echo without influencing the tachycardia (i.e., H-H interval). Hariman et al.89 demonstrated that this phenomenon was most likely due to the APD producing concealed conduction into the AVN above the dual pathways so that, when the impulse returned up the fast pathway, the upper final common pathway had not recovered excitability completely, and retrograde nodal-atrial conduction was delayed.
Earlier APDs frequently penetrate the reentrant circuit and reset it (Fig. 8-46B). Depending on how slowly the APD is conducted down the antegrade limb of the reentrant circuit, less or greater than compensatory pauses can result. On occasion, an APD can produce such marked conduction delay in the slow pathway antegradely, or in a lower common pathway, that a greater than compensatory pause results. If an APD produces a long enough pause, spontaneous sinus P waves that depolarize the atrial tissue surrounding the AVN can occur (i.e., in the HBE and the os and/or within the coronary sinus), confirming a subatrial site of the reentrant circuit (Fig. 8-51). In addition, one or two atrial extrastimuli can produce delay in a lower final common pathway, which can be recognized by a shift in the relationship of the retrograde atrial activation and the His bundle deflection. Delay in the lower common pathway (see subsequent discussion) causes delay of the impulse reaching the His bundle without influencing the return to the atrium through the fast pathway. This results in atrial activation preceding the His deflection (Fig. 8-52) during the tachycardia instead of following the His bundle deflection, as it normally does. Continuous resetting of the A-V nodal reentrant tachycardia by atrial pacing can almost universally be achieved. Portillo et al.87 also demonstrated that, although the tachycardia could be reset, rapid pacing could produce A-V dissociation and/or 2:1 nodoatrial block during the SVT, both of which further support a subatrial origin of the tachycardia.87
Finally, if the APD penetrates the circuit early enough, it will block antegradely in the slow pathway and usually collide with the circling wavefront in the beta pathway to terminate the arrhythmia (Fig. 8-53). If the rate of typical AVNRT is slow enough, and if the APD is appropriately timed, the presence of a wide and fully excitable gap (in the fast pathway) can be demonstrated by the ability of the APD to conduct down the fast pathway, capture the His bundle, and terminate the tachycardia before the wavefront in the slow pathway reaches the lower final common pathway. Thus, the last H and QRS are prematurely activated and advanced. This phenomenon is far more common in atypical AVNRT (Fig. 8-54). Here, the tachycardia is terminated by stimulation from either the high-right
atrium (Fig. 8-54A) or the coronary sinus (Fig. 8-54B), which conducts down the fast pathway during retrograde slow-pathway activation, and blocks retrogradely in the slow pathway. This is confirmed by termination of the SVT by an APD before the expected atrial echo that would have occurred over the slow pathway. The ability to conduct over the fast pathway during slow-pathway conduction and to terminate the tachycardia (whether typical or atypical AVNRT) suggests that an excitable gap exists in the reentrant circuit. (Further evidence of an excitable gap is described later in the discussion of the response to VPDs.)
atrium (Fig. 8-54A) or the coronary sinus (Fig. 8-54B), which conducts down the fast pathway during retrograde slow-pathway activation, and blocks retrogradely in the slow pathway. This is confirmed by termination of the SVT by an APD before the expected atrial echo that would have occurred over the slow pathway. The ability to conduct over the fast pathway during slow-pathway conduction and to terminate the tachycardia (whether typical or atypical AVNRT) suggests that an excitable gap exists in the reentrant circuit. (Further evidence of an excitable gap is described later in the discussion of the response to VPDs.)
The ability of an APD to terminate the arrhythmia depends on (a) the cycle length of the tachycardia, (b) the distance of the site of stimulation from the reentrant circuit, (c) the refractoriness of the intervening tissue, (d) the conduction velocity of the APD, and (e) an excitable gap in the reentrant circuit. A short SVT cycle length, a distant site of stimulation, a long atrial refractory period, a slowly conducting APD, and
the absence of an excitable gap, singly or in combination, will result in failure of the APD to penetrate and/or terminate the SVT. In my experience, AVNRTs with cycle lengths of ≤325 msec are rarely terminated by single APDs unless stimulation is carried out adjacent to the AVN. Slower tachycardias can frequently be terminated by a single APD, even though it is delivered from distant atrial sites. Two or more APDs will usually succeed in terminating rapid AVNRT when a single APD fails. The first or earlier APDs shorten atrial refractoriness, allowing the subsequent APDs to reach the reentrant
circuit at the critical time required for termination. This is also the mechanism by which rapid atrial pacing terminates arrhythmias.
the absence of an excitable gap, singly or in combination, will result in failure of the APD to penetrate and/or terminate the SVT. In my experience, AVNRTs with cycle lengths of ≤325 msec are rarely terminated by single APDs unless stimulation is carried out adjacent to the AVN. Slower tachycardias can frequently be terminated by a single APD, even though it is delivered from distant atrial sites. Two or more APDs will usually succeed in terminating rapid AVNRT when a single APD fails. The first or earlier APDs shorten atrial refractoriness, allowing the subsequent APDs to reach the reentrant
circuit at the critical time required for termination. This is also the mechanism by which rapid atrial pacing terminates arrhythmias.
![]() FIGURE 8-48 Simultaneous right and left atrial extrastimuli resulting in biatrial capture without affecting A-V nodal reentrant tachycardi a. The figure is organized similar to Figure 8-44. A-V nodal tachycardia is present at a cycle length of 425 msec. Simultaneous extrastimuli from HRA and proximal CS are delivered at 350 and 340 msec coupling intervals to their respective electrograms without affecting the tachycardia. See text for discussion. |
In an analogous fashion to atrial stimulation, VPDs can produce similar responses.94,95,96 The application of progressively premature VPDs during SVT can provide useful information regarding the presence of a lower common pathway. This depends critically on the ability to record a retrograde His potential and the capability of the VPD to reach the His bundle. As noted in Chapter 2, in approximately 85% of patients a
retrograde His deflection can be seen during ventricular pacing. The ability to reach the His bundle during a tachycardia by ventricular extrastimuli depends on the tachycardia cycle length, the local ventricular refractory period, the obligatory time required for the impulse to reach the bundle branches from the site of stimulation, and retrograde conduction time up the bundle branches to the His bundle. Thus, although VPDs can be introduced without any effect on AVNRT in all patients with A-V nodal reentrant SVT, the ability to document premature depolarization of the lower part of the AVN is limited to perhaps 60% of patients. This number can be augmented by using maneuvers or pharmacologic agents to slow the tachycardia. If one analyzes only those patients in whom retrograde His bundle depolarizations can be observed during ventricular extrastimuli during SVT, in nearly 90% of these patients the His bundle can be retrogradely captured prematurely by single (Fig. 8-55) and even double (Fig. 8-56) ventricular extrastimuli without affecting the tachycardia as manifested by a constant atrial cycle length.96
retrograde His deflection can be seen during ventricular pacing. The ability to reach the His bundle during a tachycardia by ventricular extrastimuli depends on the tachycardia cycle length, the local ventricular refractory period, the obligatory time required for the impulse to reach the bundle branches from the site of stimulation, and retrograde conduction time up the bundle branches to the His bundle. Thus, although VPDs can be introduced without any effect on AVNRT in all patients with A-V nodal reentrant SVT, the ability to document premature depolarization of the lower part of the AVN is limited to perhaps 60% of patients. This number can be augmented by using maneuvers or pharmacologic agents to slow the tachycardia. If one analyzes only those patients in whom retrograde His bundle depolarizations can be observed during ventricular extrastimuli during SVT, in nearly 90% of these patients the His bundle can be retrogradely captured prematurely by single (Fig. 8-55) and even double (Fig. 8-56) ventricular extrastimuli without affecting the tachycardia as manifested by a constant atrial cycle length.96
As with APDs, earlier VPDs can reset the tachycardia. This usually requires a very premature extrastimulus and/or a rather slow tachycardia (usually cycle lengths greater than 350 msec). Rosenthal et al.,11 in our laboratory, demonstrated that if one can reset A-V nodal reentrant tachycardia by VPDs, the presence of an excitable gap can be demonstrated in the common form of AVNRT. During a slow typical A-V nodal reentrant tachycardia, he demonstrated premature depolarization of the His bundle retrogradely over a zone of coupling intervals exceeding 100 msec without demonstrating decremental conduction in the retrograde limb (Fig. 8-57). This
finding suggests that the retrograde limb of the circuit maintained full excitability during the tachycardia and that there was a gap of more than 100 msec between the head of the impulse coming down the slow pathway and the entrance of the lower final common pathway into the fast pathway retrogradely. This is schematically shown in Figure 8-58. The presence of an excitable gap supports the concept of dual pathways based either on an anatomically determined substrate or, more likely in this instance, one based on nonuniform anisotropy in the AVN. Schuger et al.94 confirmed this observation by using verapamil to expose the excitable gap. If single VPDs cannot reset, double VPDs can.
finding suggests that the retrograde limb of the circuit maintained full excitability during the tachycardia and that there was a gap of more than 100 msec between the head of the impulse coming down the slow pathway and the entrance of the lower final common pathway into the fast pathway retrogradely. This is schematically shown in Figure 8-58. The presence of an excitable gap supports the concept of dual pathways based either on an anatomically determined substrate or, more likely in this instance, one based on nonuniform anisotropy in the AVN. Schuger et al.94 confirmed this observation by using verapamil to expose the excitable gap. If single VPDs cannot reset, double VPDs can.
![]() FIGURE 8-55 Retrograde capture of the His bundle by a ventricular extrastimulus without influencing the tachycardia. (The figure is arranged identically to Figure 8-50.) Following three complexes of atrioventricular (A-V) nodal reentry at a cycle length of 380 msec, a ventricular extrastimulus is delivered and captures the His bundle retrogradely (H′, arrow) without influencing the tachycardia, as shown by a constant A-A interval. See text for discussion. (From Miller JM, Rosenthal ME, Vassallo JA, et al. Atrioventricular nodal reentrant tachycardia: studies on upper and lower ‘common pathways’. Circulation 1987;75:930–940.) |
![]() FIGURE 8-56 Sequential retrograde capture of the His bundle without influencing A-V nodal reentry. (The figure is arranged the same as Figure 8-53.) Two ventricular extrastimuli capture the His bundle retrogradely (H′, arrow) without influencing the tachycardia. The dotted lines show where the His bundle would have been activated spontaneously during the tachycardia. See text for discussion. (From Miller JM, Rosenthal ME, Vassallo JA, et al. Atrioventricular nodal reentrant tachycardia: studies on upper and lower ‘common pathways’. Circulation 1987;75:930–940.) |
Resetting AVNRT by VPDs can occasionally be observed in the absence of atrial activation over the fast pathway (Fig. 8-59). In Figure 8-60 two VPDs reset AVNRT associated with block to the atrium (and ventricle) followed by resumption of AVNRT with intermittent retrograde block to the atrium. The schema demonstrates continuation of AVNRT with retrograde and antegrade block during the pause and intermittent block to the atrium following return of conduction to the ventricle. This finding also supports the concept that AVNRT is subatrial. Scherlag et al.97 recently demonstrated that retrograde block can occur between the slow pathway and the atrium during rapid retrograde activation of the slow pathway. Retrograde conduction from slow pathway to atrium was slower than conduction from atrium to slow pathway. This was consistent with the more “nodal-like” action potentials of the slow pathway as compared to atrial fibers.
Termination of A-V nodal reentrant tachycardia by single ventricular extrastimuli is even more difficult than with atrial extrastimuli because of the limitations imposed on the prematurity with which ventricular extrastimuli can be delivered and reach the circuit (i.e., tachycardia cycle length, conduction time to the bundle branches, and conduction through the bundle branches to the His bundle and AVN). Termination of tachycardias with cycle lengths less than 400 msec by a single ventricular extrastimulus is extremely rare. The ability to terminate a rapid SVT by an isolated extrastimulus strongly favors the diagnosis of circus movement tachycardia using a CBT (see following discussion of concealed bypass tracts). Termination of AVNRT by a ventricular premature complex can occur either in the retrograde or antegrade limb. Usually,
the slower the tachycardia, the more likely termination will be in the slow pathway. In contrast to isolated ventricular extrastimuli, rapid ventricular pacing can usually terminate even rapid tachycardias when single VPDs fail to do so. At longer-paced cycle lengths than those that terminate AVNRT, the AVNRT will be reset with a V-A-V response (see discussion later in this chapter regarding the use of overdrive ventricular pacing to distinguish AVNRT, AVRT, and AT).
the slower the tachycardia, the more likely termination will be in the slow pathway. In contrast to isolated ventricular extrastimuli, rapid ventricular pacing can usually terminate even rapid tachycardias when single VPDs fail to do so. At longer-paced cycle lengths than those that terminate AVNRT, the AVNRT will be reset with a V-A-V response (see discussion later in this chapter regarding the use of overdrive ventricular pacing to distinguish AVNRT, AVRT, and AT).
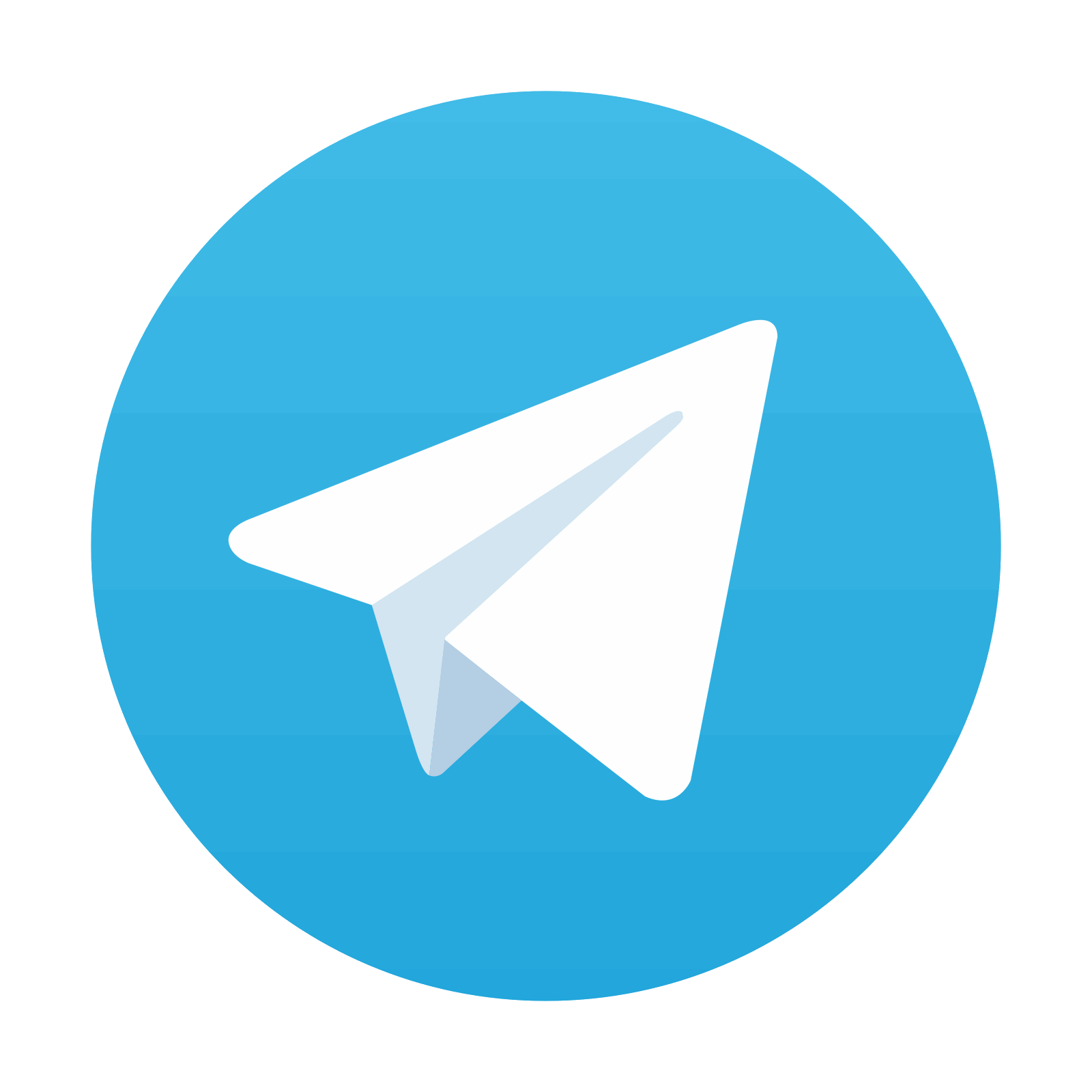
Stay updated, free articles. Join our Telegram channel

Full access? Get Clinical Tree
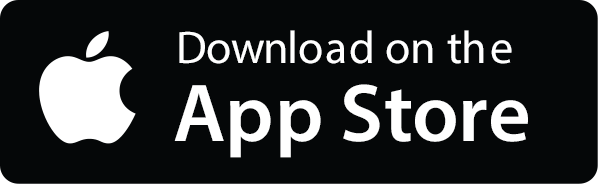
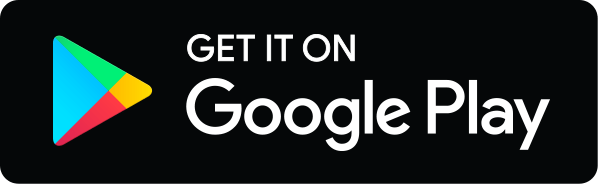
