Abstract
When infants die suddenly and unexpectedly, the causes can be effectively considered using a triple risk model of individual infant susceptibility, developmental vulnerability, and environmental stressors. Sleep practices, particularly prone sleeping and bed sharing, increase the risk posed to vulnerable infants by environmental factors.
Infants born prematurely are at increased risk for sudden unexpected death, most likely because of immaturity of respiratory control that causes hypoxemia and long apneas.
Infants coming to an emergency department because of a possible cardiorespiratory event that was worrisome to a caregiver should be managed along a spectrum that includes reassurance but is informed by an awareness of potentially serious problems presenting as a nonlethal event.
Keywords
ALTE (apparent life-threatening event), BRUE (brief, resolved, unexplained event), SIDS (sudden infant death syndrome), apnea of prematurity
Infants experiencing an apparent life-threatening event (ALTE) and premature infants with apnea may later die suddenly and unexpectedly, with a diagnosis of sudden infant death syndrome (SIDS). However, because about 80% of victims who died suddenly and unexpectedly were born at term and were never known to be apneic until they died, discussing ALTE, apnea of prematurity (AOP), and SIDS together has become controversial, particularly when the issue of apnea monitoring is raised.
The American Academy of Pediatrics has recently promulgated a new category for this discussion—BRUE, Brief Resolved Unexplained Event. This new designation attempts to address the appropriate clinical approach to young infants coming to emergency departments with a history of events that, far more often than not, prove to be benign, and may be properly managed by reassuring parents and caregivers.
Acute Life-Threatening Events (ALTE) and BRUE
Epidemiology
The term apparent life-threatening event (ALTE) was first coined following the 1986 National Institute of Health Consensus Conference on Infant Apnea. The intention at the time was to replace the term “near-miss sudden infant death syndrome.” ALTE is defined as “an episode that is frightening to the observer that is characterized by some combination of apnea (central or occasionally obstructive), color change (usually cyanotic or pallid but occasionally erythematous or plethoric), marked change in muscle tone (usually marked limpness), or choking or gagging.”
In 2016, the American Academy of Pediatrics (AAP) released a clinical practice guideline with the objective of replacing the term ALTE with a new term: BRUE. This guideline was published to allow the clinician a practical approach to the evaluation of a child with the features of an ALTE who is considered low risk. BRUE was specifically defined as an “event occurring in an infant younger than 1 year” who is reported to have a “sudden, brief, and now resolved episode of one or more of the following: (1) cyanosis or pallor; (2) absent, decreased, or irregular breathing; (3) marked change in tone (hypertonia or hypotonia); and (4) altered level of responsiveness.” A BRUE is distinguished from an ALTE in that it is defined as both brief and resolved ( Table 80.1 ). Therefore children who have not returned to their baseline state of health would not fit into this category. By providing a more specific definition of these events, it is anticipated that clinicians will avoid overuse of medical interventions and testing in the group that is considered low risk. In addition to clarifying what an event entails, the term BRUE is favored by the AAP over ALTE, because the term “life-threatening” is often misleading and has the potential to reinforce caregivers’ perceptions that the event was, in fact, life-threatening, making it more challenging to provide reassurance when often that is all that is needed.
Includes | Excludes | |
---|---|---|
Brief Resolved |
|
|
Unexplained | Not explained by an identifiable medical condition |
|
Event Characterization | ||
Cyanosis or pallor |
|
|
Absent, decreased, or irregular breathing |
|
|
Marked change in tone (hypertonia or hypotonia) |
|
|
Altered responsiveness |
| Loss of consciousness associated with breath holding spell |
The incidence of ALTE in the population worldwide is estimated between 0.6 and 4.1 per 1000 live births ; however, the true incidence of ALTEs is unknown, especially given that the International Classification of Disease (ICD) code for ALTE was not introduced until 2012. It is estimated that ALTE accounts for 0.6%–1.7% of emergency department visits for children younger than 1 year. The usefulness of the BRUE designation and its impact on ALTE statistics remains to be seen.
Diagnosis and Differential Diagnosis
As described above, BRUE refers to an event ( Table 80.1 ) with specific parameters. BRUE should be diagnosed only when there is no explanation for a qualifying event after conducting an appropriate history and physical examination.
When evaluating the child who presents for medical attention following an event, a thorough physical examination and history is required to determine the risk of severe sequelae, as management guidelines for BRUE pertain only to those individuals who meet lower risk criteria, which are as follows:
- ▪
Age more than 60 days
- ▪
Prematurity: gestational age ≥32 weeks and postconceptional age ≥45 weeks
- ▪
First BRUE (no previous BRUE ever and not occurring in clusters)
- ▪
Duration of event less than 1 minute
- ▪
No cardiopulmonary resuscitation (CPR) required by trained medical provider
- ▪
No concerning historical features
- ▪
No concerning physical examination findings
Respiratory syncytial virus, pertussis
Sepsis with apnea
Syndromes compromising the upper airway (e.g., Pierre Robin sequence)
Breath-holding spells
Seizures
Intracranial hemorrhages (e.g., caused by vascular malformations, child abuse, vitamin K deficiency)
Exaggerated laryngeal chemoreflex with or without gastroesophageal reflux
Drugs (e.g., phenothiazines, over-the-counter cold remedies)*
Tachyarrhythmias (e.g., Wolfe-Parkinson-White syndrome, prolonged QT syndrome)
Inborn errors of metabolism (e.g., MCAD deficiencies)
Hypoventilation during bed sharing or because nose and mouth become covered with bedding
Etiology and Pathology/Pathogenesis
We will now discuss potential explanations for ALTE as that term is currently used. Box 80.1 outlines considerations when the findings of hypotonia, cyanosis, and possible apnea persist or recur, thus exceeding the criteria for a BRUE.
It is our experience, and the published experience of others linking GER to ALTE, that the history in ALTE infants often suggests apnea after choking. It seems likely that infants whose ALTE resembles apnea after choking have an exaggerated laryngeal chemoreflex. For this reason, we will discuss Laryngeal ChemoReflex Apnea (LCRA) in detail ( Fig. 80.1 ).

The larynx is a complex structure enriched with hundreds of nonmyelinated sensory nerve endings, including chemoreceptors and mechanoreceptors involved in maintaining upper airway patency. Laryngeal mucosal chemoreceptors function as irritant receptors, water receptors, and C fiber endings. These nerve endings are most numerous in the mucosal epithelium of the epiglottis, aryepiglottic folds, and interarytenoid space. Water receptors respond to either reduced osmolarity or reduced chloride ion concentration. C fibers are stimulated by noxious agents, such as temperature, ammonium, capsaicin, and H + ions. Stimulation of these unmyelinated nerve fibers arising in the laryngeal epithelium and traveling in the superior laryngeal nerve cause prolonged apnea, bradycardia, and marked increases in central venous and arterial blood pressure in experimental animals. Thus, both animals and humans can develop laryngeal chemoreflex apnea.
Any liquid in contact with the laryngeal mucosa will trigger a more or less brisk LCRA. The sequence of response after single-fiber stimulation of these nerves is dramatic and is most marked in younger animals (e.g., piglets, lambs, and puppies). Apnea beginning within 0.25 seconds of the stimulus may last 20–90 seconds and may be fatal in the laboratory. Saline elicits little or no response, whereas water and cow’s milk elicit a dramatic LCRA.
As in experimental animals, among human preterm infants, water is more potent than normal saline in eliciting severe LCRA. In infants, should swallowing be delayed or fail to occur, the apneic phase of the laryngeal chemoreflex can be prolonged, extending over 20 seconds and causing hypoxemia. The duration of the apneic phase of the laryngeal chemoreflex (LCR) seems to be inversely proportional to postnatal age. Taken together, these findings suggest that in immature subjects, airway protection from aspiration takes precedence over ventilation in some circumstances when even small quantities of fluids (~0.1 mL), including water, come in contact with the larynx.
Human infants attempt to clear fluid that elicits LCRA by laryngeal closure and swallowing during a central apnea (see Fig. 80.1 ). After the central apnea, any breaths attempted are obstructed so long as upper airway closure persists. When swallowing clears the fluid, the upper airway is opened with a return to eupneic breathing. The LCR sequence in more mature infants and experimental animals does not involve prolonged apnea, and is as follows: cough, swallowing, and arousal if asleep. The cough-swallow-arousal sequence is much less common when elicited during sleep in immature subjects.
With maturation in older infants and older experimental animals, apnea occurs as part of the laryngeal chemoreflex, but it is shorter than in preterm infants and newborn animals. Maturation is associated with reduction in central respiratory inhibition. Cough and arousal from sleep are also more likely to occur among mature subjects when water, acid, or milk come in contact with the larynx. Nevertheless, in susceptible infants, delays in maturational influences could put them at risk for prolonged apnea as part of the laryngeal chemoreflex.
The role of gastroesophageal reflux (GER) in eliciting apnea through the LCR and therefore as a cause of ALTE or BRUE is controversial. Indeed, the new guidelines defining BRUE explicitly state that any event consistent with GER is not a BRUE, perhaps because GER in and of itself may be associated with a changed respiratory pattern. As noted above, in animals and infants, milk and water can have a profound response from LCR. Stimulation need not be acidic to elicit a LCRA. There are no distal esophageal receptors known to elicit apnea; therefore reflux must be to the level of the pharynx to stimulate LCRA. Reflux to the level of the larynx may occur in 60%–70% of episodes of GER. The temporal sequence of GER eliciting a subsequent apnea and potential pathogenesis in term infants has been demonstrated, but the association among preterm infants is less clear, perhaps because the high background rate of central apneas in preterm infants makes establishing a link to antecedent GER events more challenging. Therefore GER may be one explanation for ALTE, particularly in select term and premature infants, however, proving the association is difficult and, moreover, reflux may occur in the absence of any abnormal respiratory patterns.
Clinical Features of an Apparent Life-Threatening Event
Symptoms
Most infants who come to medical attention following an ALTE are asymptomatic at the time of presentation. The history should attempt to provide a clear understanding of the timing and nature of the event as well as pertinent past medical history, family history, environmental exposures, and social history. The attending physician supervising the evaluation should make every effort to obtain the history himself or herself, including the duration of the event, time of day, adequacy of lighting, infant’s position within his or her surroundings, and whether the episode began while awake or asleep. The infant’s color, tone, activity, and movements at the time of event (e.g., repetitive motions) must be noted. Was blood or pink froth coming from the infant’s mouth or nose, for instance, or did the caregiver notice any other unusual findings? Were any measures taken to stop the event (stimulating the infant through touching or blowing on the face) and did the infant require resuscitation (i.e., CPR)? Who was present and witnessed the event and who was the responsible caregiver at the time that this occurred? The appropriateness of the caregivers’ concern should be noted. Any glaring inconsistencies in the history should raise suspicion for nonaccidental trauma or attention-seeking behavior. Beyond the event itself, a thorough medical and family history should be obtained. Has the infant been growing and developing normally? Is there a history of prematurity, prior hospitalization, GE reflux, prior injury, snoring, or noisy breathing? Was the infant in the early stages of a respiratory illness, or was he or she having coughing paroxysms? Family history of seizures, arrhythmias, sudden death, or serious illness with coma among young people must be ascertained. A thorough list of pertinent questions to ask when taking a history for an ALTE or BRUE is available in the BRUE guidelines.
Physical Findings
Just as the majority of infants are asymptomatic at presentation, physical exam findings are often normal. Nevertheless, a thorough physical examination is essential in detecting a possible etiology for the event, and suggesting specific needs for further diagnostic evaluation. As with any disease presentation, accurate vital signs are an essential initial assessment. Fever, tachypnea, hypotension, or hypoxemia should clue the clinician into an underlying illness such as focal infection, generalized sepsis, or underlying cardiovascular or respiratory disease. Growth parameters can also be clues, with poor weight gain since birth suggestive of chronic underlying disease, and large head circumference suggesting a neurological disorder or bleeding related to head trauma. A thorough neurological examination should consider the infant’s level of responsiveness and alertness as well as tone, symmetry of reflexes and movement, pupillary responses, and size; it should also include a funduscopic exam for retinal hemorrhages. A limb that does not move spontaneously or one that is deformed, bruised, swollen, or painful when palpated suggests an underlying fracture and possible child abuse. Nasal congestion, coryza, cough, and other abnormal respiratory findings (crackles, wheezes, tachypnea, stridor, or retractions) might suggest an upper or lower respiratory tract infection. A hyperdynamic precordium, cardiac murmurs, gallop rhythms, or heart rhythm irregularities warrant further investigation for an underlying congenital or infectious cardiac disorder such as cardiomyopathy or myocarditis. Abdominal distension or organomegaly could be clues to infection or a metabolic disorder. Infants younger than 2 months of age should be considered at risk for bacteremic sepsis when they present with apnea or hypotonia and should be treated accordingly.
Management and Treatment of Apparent Life-Threatening Event
A systematic review for the management of ALTEs was published in 2013 that attempted to address some of the challenges of the clinical management of patients who experience such an event. The challenges increase when trying to address the patient who is asymptomatic by the time of presentation to the medical team. While events called ALTE are ultimately benign, they may be an initial presentation of a significant underlying disorder. Trying to determine which child deserves a more extensive evaluation and what that evaluation may entail, including diagnostic testing and need for hospitalization, is not only challenging but fraught with controversy. Indeed, some institutions have developed clinical decision rules to determine which infants may be safely discharged home from the ER following an ALTE. The newer BRUE guidelines attempt to further address these issues and outline management recommendations based on available research to apply to those infants deemed low risk. The guidelines were developed by a multidisciplinary subcommittee of the AAP composed of a group of experts from various fields of medicine, epidemiologists, methodologists, and parent representatives. They performed an extensive review of the literature related to ALTEs from 1970 through 2014 to develop their definition and recommendations. The authors recognized that the strength of their recommendations for low-risk infants is moderate to weak because of lack of high-quality evidence and studies. It is essential to understand that the guidelines for BRUE only apply to low-risk infants who have returned to baseline and any abnormal physical examination findings or history should warrant further investigation.
Management guidelines for higher risk infants have not yet been established but the available studies to date would recommend a tailored approach based on the findings from the history and physical examination (for further reading, we recommend reviewing the tables within Tieder et al., Pediatrics 2016 outlining the historical features to be considered in the evaluation of a potential BRUE). That is, while there are many laboratory studies and diagnostic tests that could be performed to determine the etiology of the event, only certain tests may be necessary or appropriate in individual cases.
Imaging and Laboratory Findings
The 2016 guidelines for BRUE suggest minimal testing for those infants who meet the criteria for low risk. These recommendations advise against the routine use of chest radiographs, blood gases, polysomnograms, echocardiograms, neuroimaging, and blood evaluations for culture, white blood cell count, or metabolic disorders. They do recommend that caregivers be offered resources for CPR training and given education on BRUEs. Furthermore, recommendations and testing should be done through a shared decision-making platform between medical provider and caregiver.
The following tests may be informative when the criteria for a BRUE are exceeded, for example, recurrent events over a short period of time, abnormal physical findings, or worrisome history. Initial laboratory evaluation may include measurement of hemoglobin and white blood cell count with differential cell count; culture of blood and urine; nasal swabs for respiratory syncytial virus (RSV) and pertussis; a chest radiograph; and serum glucose, sodium, potassium, chloride, and bicarbonate. The child who has a concerning history and is not back to baseline should be admitted to a unit where cardiorespiratory monitoring is available so that abnormalities in cardiac or respiratory rate can be recorded. If the infant continues to be limp, or if apnea recurs, one should measure arterial blood gases, serum lactate, and ammonia, and screen the urine for abnormal levels of amino and organic acids, and drugs. In children who continue to appear ill or who have altered mental status with no other cause detected, video electroencephalography (EEG) should be conducted and imaging of the central nervous system by computed tomography or, preferably, magnetic resonance imaging, should be performed.
The clinical approach to an ALTE should, of course, be based on one’s assessment of the validity of the caregivers’ observations: “benign physiological events in babies may sometimes cause an overreaction by parents, particularly those who are anxious, are attention-seeking, or suffer a personality disorder.” On the other hand, one’s approach should also be informed by the knowledge that 89% of infants with documented prolonged apnea, bradycardia, and marked hypoxemia when subsequently hospitalized for an ALTE had appeared well in the emergency department ( Fig. 80.2 ).

Prevention: Home Apnea Monitors and Feeding Infants With Exaggerated Laryngeal ChemoReflex Apnea
Because the pathophysiology and underlying causes of ALTEs or BRUEs are usually difficult to identify, how to prevent these events is not known. That said, much concern regarding BRUE and ALTE arises from the worry that they are a signal of an underlying condition or an indication of risk for a future SIDS event. Therefore these children are often discharged home with apnea monitors in the hope of preventing SIDS. Home monitors are often prescribed by practicing physicians when a child is deemed at risk for a cardiorespiratory event. In a retrospective review of 88 infants prescribed home monitors at a tertiary hospital, indications for monitoring included family history of SIDS in 21%, apnea of prematurity in 25%, history of previous ALTE in 21% and a choking episode in 15%.
The CHIME (Collaborative Home Infant Monitoring Evaluation) study was an NIH-funded study developed in order to determine whether home monitors were effective in identifying episodes of apnea or bradycardia that were potentially threatening to an infant’s health. It enrolled a total of 1079 infants between 1994 and 1998, and included a group of healthy term infants, preterm infants, infants who had experienced an ALTE, and infants whose siblings died from SIDS. Extreme and conventional events were determined based on degree and duration of bradycardia and apnea. The CHIME monitor included both EKG and pulse oximetry recordings as well as Respiratory Inductance Plethysmography (RIP) bands. The RIP bands allowed the researchers to evaluate episodes of apnea as obstructive or not and found that 70% of “extreme” events and 50% of “conventional events” were associated with obstructed breaths. By using RIP bands, they were able to show that apnea identified by impedance cardiorespiratory monitoring was concordant with that found by RIP. The authors concluded that events, in general, were common, including in the healthy term infants. Extreme events, defined as apneas ≥30 seconds and heart rate less than 50 bpm (≥44 weeks PCA) or 60 bpm (<44 weeks PCA) for at least 10 seconds were more likely in preterm infants, and tended to recur within 6 weeks. The CHIME study did not detect an association between extreme events and death or neurologic injury, with only 6 deaths occurring during the study, and it did not address whether use of monitors would decrease SIDS rates. In a retrospective review of a similar, smaller cohort of infants, 36% of infants had a significant event on home monitoring, which occurred most often within the first month, and more so the first week, of monitoring. This retrospective study also found that preterm infants were more likely to have events than those with prior ALTE, a SIDS sibling, or infants monitored due to parental anxiety. An event during a hospitalization also increased the likelihood of finding an event on home monitoring (see Fig. 80.2 ).
The American Academy of Pediatrics’ (AAP) last review and guidelines for the use of home monitors was published in 2003. The AAP recommends monitoring in two specific circumstances: (1) it “may be warranted” in selected premature infants until “43 weeks postmenstrual age,” or until “extreme episodes” of apnea, bradycardia, and hypoxemia stop; (2) infants who are usually supported at home by mechanical ventilation through a tracheotomy. Routine monitoring is not recommended by the AAP for infants with an ALTE or among siblings of SIDS victims. In a list of instructions, the AAP states: (1) “Home cardiorespiratory monitoring should not be prescribed to prevent SIDS”; (2) “Parents should be advised that…monitoring has not been proven to prevent sudden unexpected deaths…” The AAP’s recommendations reflect an accurate interpretation of published reports but also reflect compromises that are believed by some to be too generous toward or too restrictive of monitoring.
A 2011 systematic review of the reduced rates of SIDS identified 11 prospective studies, but only one provided high-level evidence. The majority of studies reviewed were cohort studies from the 1980s. Many of these studies were flawed in their methodology or description of study design, and the type of home monitoring device varied among the studies and even within a single study. A total of 2210 infants had been studied over an average of 5.5 months with a rate of SIDS deaths of 5.0 deaths per 1000 (95% CI 1.4–11.0). While the review concludes that there is little evidence that home monitoring may prevent SIDS, the authors go on to say that “the lack of high-level evidence of effectiveness of home monitoring does not prove that it is ineffective. Indeed, the lack of evidence may be more of a reflection of the difficulty in designing an appropriate and rigorous study in this sensitive area, where the well-being of any child enrolled must be given much higher priority than the more abstract needs of researchers.”
In our opinion, approaches to monitoring that are dogmatic, either pro or con, and not sympathetic to parents’ concerns, should not be the norm. Thus, although it is the official recommendation of the AAP that ALTE patients not be monitored, this recommendation remains controversial, and home monitoring appears to be a common practice.
Apnea in Premature Infants
Diagnosis and Differential Diagnosis
During their initial hospitalization, 50%–100% of premature infants born weighing less than 1500 g will require mechanical or pharmacological interventions because of apnea. Apnea of prematurity (AOP) is defined as cessation of airflow for 10–20 seconds or longer. Shorter pauses in respiration associated with falls in Sp o 2 % (e.g., to <90%) or in heart rate (e.g., to <100 beats/min) are also seen as important episodes of apnea in most neonatal intensive care units (NICUs). However, if apnea is less than 10 seconds, there is little consensus about the amount of reduction in Sp o 2 % that is worrisome.
Other possibilities in the differential diagnosis of AOP besides immaturity of ventilatory control include: intracranial hemorrhage, sedation crossing the placenta, sepsis with or without meningitis, heat or cold stress, a patent ductus arteriosus, hypoglycemia, electrolyte abnormalities (particularly hyponatremia), anemia, necrotizing enterocolitis, feeding-related apnea, heart block or heart failure lowering cerebral perfusion, and excessive sedation given to the infant directly.
The majority of apnea among premature infants is “idiopathic,” and attributed to immaturity of ventilatory control.
Pathogenesis of Apnea of Prematurity
Ventilatory control among premature infants is sufficiently unstable that apnea, even prolonged, is provoked by problems (e.g., hyponatremia, heart failure) that would not be sufficient explanations beyond term postmenstrual age (PMA). Thus, the primary instability of ventilatory pattern and drive among premature infants, even when they are “well,” is important.
It is simplistic to state that most apnea among premature infants is central, although most episodes can be prevented with stimulants whose primary action is central. Indeed, it is probable that the majority of apneic events in premature infants have both obstructive and central components (i.e., they are mixed). Furthermore, it seems likely that all three types of apnea described in preterm infants—central, obstructive, and mixed—occur when the frequency of output from the respiratory controller is at a low ebb, “suggesting that all 3 patterns had one common underlying mechanism.”
There is little recent published work that describes the prevalence of the various types of apnea (central, obstructive, or mixed) or the impact of sleep state, or that explores critically the continued relevance of what has become “cribside” dogma. Moreover, clinical rules have often been based on few observations; for example, the dogma that apnea is much more common in active than in quiet sleep is based on studies done on nine term infants. With many smaller and more premature infants surviving, since the introduction of artificial surfactant over 25 years ago, these dicta should be reexplored.
It was observed in the 1980s that the majority of central apneic episodes were either preceded or followed by evidence of upper airway obstruction. During apnea in premature infants, respiratory effort often continues, or resumes, before airflow returns, with phasic contraction of the diaphragm (electromyogram) associated with generation of progressively more negative intrathoracic pressures ( P esoph ). Near the end of a typical mixed apnea, genioglossus contraction (submental electromyogram) opens the airway and flow resumes. It was thus recognized that apneic episodes in premature neonates could also appear on recordings to be bradycardia with desaturation, because impedance chest movement monitors would correctly indicate that respiratory efforts continued.
During quiet sleep, premature neonates with frequent apnea are less able than nonapneic controls to compensate for progressive increases in upper airway resistance. Neonates with apnea have much shorter and weaker inspiratory efforts in response to end-expiratory occlusion. Premature neonates with apnea also have a higher Pa co 2 (~9 mm Hg higher) and a much flatter ventilatory response to breathing 4% CO 2 ( V E /min/kg) compared to controls. In addition, infants, in general, have a flatter CO 2 response when breathing progressively more hypoxic gas mixtures.
A series of explanations for the most common type of apnea among premature infants—mixed apnea—can be constructed from these classic studies:
- 1.
Upper airway obstruction, for which the infant has shorter and weaker “load compensation,” is preceded by brief central apnea, or leads to longer central apnea.
- 2.
Infants with a lower minute volume response to increasing CO 2 become progressively more hypercarbic and hypoxemic.
- 3.
The development of hypoxemia further blunts CO 2 response, and the increasing CO 2 makes the activities of the diaphragm and genioglossus asynchronous, perpetuating the cycle of obstructive and central (i.e., mixed) apneas.
Other “classic” studies from the mid-1980s suggested additional physiologic mechanisms to explain why premature infants might be more at risk for mixed apnea and its complications during active sleep:
- 1.
The inspiratory “load” for which premature infants are unable to “compensate” is due to upper airway narrowing, which usually occurs at the pharynx.
- 2.
Loss of intercostal tone during active sleep would increase wasted “distortional” work during the paradoxical breathing caused by pharyngeal airway narrowing.
- 3.
Loss of intercostal tone would also diminish functional residual capacity and thus worsen hypoxemia during compromised breathing.
Furthermore, the LCRA, described above, may also play a role in AOP. Swallowing interrupts LCRA. Premature infants swallow more frequently during apnea than during eupneic breathing. This suggests that exaggerated LCRA, such as that caused by spontaneous pooling of saliva, may explain some apneic episodes. LCRA is, in fact, mixed apnea, and similar to the most frequent type of AOP.
Although it may be simplistic to describe AOP as “primarily central,” inadequate responses to upper airway obstruction do reflect a significant “central immaturity” among premature infants. Other evidence of the “central immaturity” of younger infants is the marked increase in periodic breathing particularly during active sleep. After the onset of periodic breathing, transient upper airway obstruction at the pharynx is common during the first inspiratory effort after the apneic pause. Thus, excessive periodic breathing, which is considered pathognomonic for “central immaturity” of respiratory control, frequently has both central and obstructive (i.e., mixed) apnea components.
During epochs of periodic breathing, preterm infants have falls in Sp o 2 % and are widely believed to be more susceptible to prolonged apneas ( Fig. 80.3 ). A recent longitudinal study of 24 infants born between 27 and 36 weeks gestation showed that all but one had periodic breathing during 2–3 hour recordings during naps. The infants were 2, 4, and 6 months postterm corrected age. For most infants, the periodic breathing runs were short and benign, though falls in Sp o 2 % and cerebral tissue oxygenation index were often statistically significant. Three infants had large and sustained falls in heart rate, Sp o 2 % (to <80%), and cerebral oxygenation index. Their susceptibility to periodic breathing may be enhanced by the fact that their Pa co 2 during eupneic breathing may be only 1.3 mm Hg above their apneic threshold. Thus any ventilatory overshoot after a periodic apnea makes preterms particularly vulnerable to resuming apnea. In this regard, evidence from lambs induced to have periodic breathing suggests that increases in lung volume from adding CPAP served to reduce the overshoot, or “loop gain,” after a periodic apnea, followed by shortened epochs of periodic breathing.

Premature infants also have more active sleep than quiet sleep, with the attendant irregularity in tidal volume ( V T ) and ventilatory frequency ( V f ), and increases in both prolonged apnea and periodic apnea/breathing. In the episodes of apnea when obstruction is not found (<50%), irregularities in respiratory pattern ( V f , V T , periodic breathing) often explain a propensity for apnea that is “primarily central.”
Prognosis of Apnea of Prematurity
The natural history of AOP is unclear because interventions to treat it, and thus alter its natural history, have been tried ever since it was first described.
Apneic pauses longer than 5 seconds are common on the first day of life among premature infants without respiratory distress syndrome and can increase to 25 per day by the third day. During a 5-year period (1989–1994), one NICU discharged 457 infants born at 24–28 weeks PMA. All infants enrolled in the study had been treated because all had apnea lasting longer than 20 seconds. In general, infants born earlier had apnea and bradycardia events that persisted until an older PMA. In the study by Eichenwald and coworkers, apnea was diagnosed when chest wall movement was not detected by impedance monitoring. The last events to disappear with maturation were bradycardia episodes without associated central apnea. As noted earlier and as recognized by the Eichenwald et al., the explanation may be that obstructive apneic episodes with bradycardia continued until the age that the bradycardia episodes ceased. Another study using transthoracic impedance but incorporating motion-artifact resistant oximetry has led to similarly plausible speculation. Finally, evidence for persistent propensity for undetected mixed apneas is also suggested by detailed descriptions of maturation of upper airway–stabilizing mechanisms. There seems to be a growth- and age-dependent threshold for improvement in upper airway stability beginning at about 36 weeks PMA.
One group of preterm infants whose ventilatory and general “autonomic instability” may not be appreciated are so-called late preterm infants, born at 33 or 34–37 weeks PMA. The majority (75%) of preterm births in the United States each year are in this group, and over 400,000 infants are born at 33–37 weeks annually. Most attention in diagnosing and treating apnea of prematurity is directed to infants born less than 32 or less than 28 weeks PMA. However, compared to term infants in the CHIME study (Collaborative Home Infant Monitoring Evaluation), late preterm infants had a relative risk less than 5 for “extreme” apnea lasting longer than 30 seconds, or bradycardia (see Fig. 80.2 ). Further, in our experience, late preterm infants not infrequently return to hospital with, for example, excessive periodic breathing, hypoxemia, and apnea at 40 weeks PMA or older, and clinicians seem surprised at the severity of their ventilatory instability. It is clear that postnatal maturation of ventilatory control among very preterm infants is not the same as infants of the same PMA but born at term. Whether and how much this delay in maturation occurs in late preterm infants is not clear. But concerns are growing about respiratory morbidity and about neurodevelopmental milestones in late preterms, particularly with regards to mathematics and reading skills. This has led to trials of interventions such as betamethasone during late preterm labor (34–37 weeks) to begin to address a series of problems that are common and that were underappreciated until recently. Studies are also needed to clarify whether the ventilatory control problems of some late preterm infants, as in the CHIME study, contribute to neurodevelopmental complications.
Management and Treatment for Apnea of Prematurity
The first-line treatment for AOP remains methylxanthines, with approximately 75% of premature infants responding. Caffeine has replaced theophylline as the methylxanthine of choice because it is as effective and less likely than theophylline to cause tachycardia or poor feeding. Caffeine is thought to act by blocking brainstem inhibitory adenosine A 1 receptors, leading to increased respiratory neural output.
Pulmonologists should also know that much enthusiasm has developed recently for the early introduction of caffeine for prematures weighing 500–1250 g, even before apnea of prematurity necessitates increased ventilatory support. It is not clear why, but subjects started on caffeine within the first 10 days of life in a randomized, controlled trial involving 2006 infants had: (1) shorter need for supplemental O 2 and CPAP ; and (2) less neurodevelopmental disability at 18–21 months postterm corrected age. Caffeine was begun before chronic lung disease had developed, and continued until apnea, if it developed, had resolved. How caffeine given more or less prophylactically might reduce the incidence of chronic lung disease is not clear. Studies in animal models suggest that its effect may be through adenosine receptors both on lung inflammatory cells and on airway smooth muscle that determine the responses to inflammation or through prevention of the functional, structural, and inflammatory changes induced by hyperoxia. Furthermore, although it reduces apnea frequency and, presumably, the associated hypoxemia, whether these effects on ventilatory stability provide the explanation for caffeine’s apparent impact on neurodevelopmental outcome was not addressed in the large randomized trial. The longer-term effects of caffeine on oxygenation are suggested by a study showing that earlier cessation of caffeine treatment according to nursery protocol was associated with longer periods of intermittent hypoxemia at 35 and 36 weeks PMA than if caffeine was continued longer until clinical concerns regarding apnea had resolved.
Infants who fail to respond to methylxanthines will often respond to nasal continuous positive airway pressure (NCPAP) or nasal intermittent positive pressure ventilation. However, concerns about toxicity from high-dose methylxanthines and mucosal injury from NCPAP have led to widespread enthusiasm for early use of high-flow nasal cannulas. High-flow nasal cannulas use adequately warmed and humidified air to deliver distending pressures that are presumably comparable to NCPAP, and are often used at flow rates of 2.5 L/min, or more. These catheters have been shown to be as effective as NCPAP in reducing apnea, bradycardia, and episodes of desaturation. High-flow cannulas appear to stave off intubation both by stabilizing respiratory mechanics and treating AOP. Potential explanations for the efficacy of high-flow therapy in treating AOP have not been tested empirically, despite its frequent use for this purpose. However, it seems plausible that distending pressures from the high-flow catheters might mitigate the obstructive component of a typical preterm’s mixed apnea ( Fig. 80.4 ). Also, continued flow per se might stimulate laryngeal flow receptors and, in effect, feed forward to stimulate continued respiratory effort.

Another older intervention for apnea, blood transfusion, remains controversial. The long-term effect of transfusion therapy on reducing AOP is not clear. However, it has recently been shown that infants have more apnea when their hematocrit is lower, and apnea is less frequent for 3 days after a transfusion.
Pediatric pulmonologists who are not based in NICUs should be reminded that premature infants are at much greater risk for serious apnea after general anesthesia. After anesthesia, increased frequency of apnea and bradycardia can continue to beyond 46 weeks PMA, particularly among infants who required prolonged ventilatory support just after birth, or who have significant residual lung injury. Pulmonologists should be aware of the policies of local pediatric anesthesiologists with respect to perioperative monitoring, even for semielective surgery such as herniorrhaphy. A systematic review from Copenhagen emphasized that “Grade 1 evidence (randomized, controlled trial) exists for recommending regional versus general anesthesia when possible, and for the efficacy of prophylactic caffeine (10 mg/kg IV) on the day of surgery for preterms less than 44 weeks PMA.” The Danish authors recommend at least 12 hours of nurse-supervised, postanesthesia monitoring using ECG and oximetry for: (1) all preterms less than 46 weeks PMA; (2) for infants 46–60 weeks PMA with a hematocrit less than 30%, and, (3) those 46–60 weeks PMA with “co-morbidities” including chronic lung disease, a history of apnea, or CNS abnormalities. Six hours of recovery room monitoring is otherwise recommended for preterms 46–60 weeks PMA. A recent study by the Pediatric Sedation Research Consortium involving over 57,000 patients receiving sedation showed that former preterm infants are at a small but significant risk for airway obstruction, lower Sp o 2 %, and other complications, and that risk that can extend into adulthood.
Monitoring Premature Infants at Home
Studies evaluating compliance with apnea monitor use suggest high rates of compliance among infants with AOP, particularly during the first month at home. Abuses because of self-interest among those prescribing monitors notwithstanding, the promise or potential of monitors, when used to prevent deaths among “nursery graduates,” should not be discounted. Infants born at 24–36 weeks PMA are 2.1–3.3 times as likely to die of SIDS as infants born at greater than 37 weeks.
The issue of monitor use is quite complex, however. In their review of more than 37,000 deaths and 3.8 million linked births, Malloy and Hoffman showed that, depending on the estimated gestational age, the age of SIDS deaths among premature infants was, on average, 44.2 weeks to 47.8 weeks PMA (range, 32–85 weeks). On the basis of these data and those of the CHIME study, wherein “extreme” apneic spells among premature infants “disappeared once the infants were 43 weeks postconceptional age,” one editorial writer declared that the usefulness of prescribing monitors to detect extreme apneic spells and to prevent sudden death among premature infants and the “physiological basis for such a practice are more in doubt than ever.” However, because infants who have died were certainly apneic at least once, another possible scenario is that the time course of dangerous apnea activity among those premature infants dying is different from those having apnea and not dying. The vexing problem remains whether it is possible to select candidates who will benefit most from monitoring. Monitoring for apnea in preterm infants must continue to be part of this discussion. Malloy has shown that the adjusted odds ratio for deaths called SIDS for infants born between 24 and 28 weeks was 2.57, even given the propensity for attributing their deaths to causes related to complications of prematurity.
For the time being, we are in agreement with the AAP recommendations for monitoring premature infants having apnea until they are 43 weeks PMA. Because the average time course until cessation of apnea among those infants dying must be different from that for the premature infants in the CHIME study, monitoring infants past 43 weeks PMA who have frequent apnea lasting longer than 20 seconds, especially with reductions in heart rate, also seems prudent.
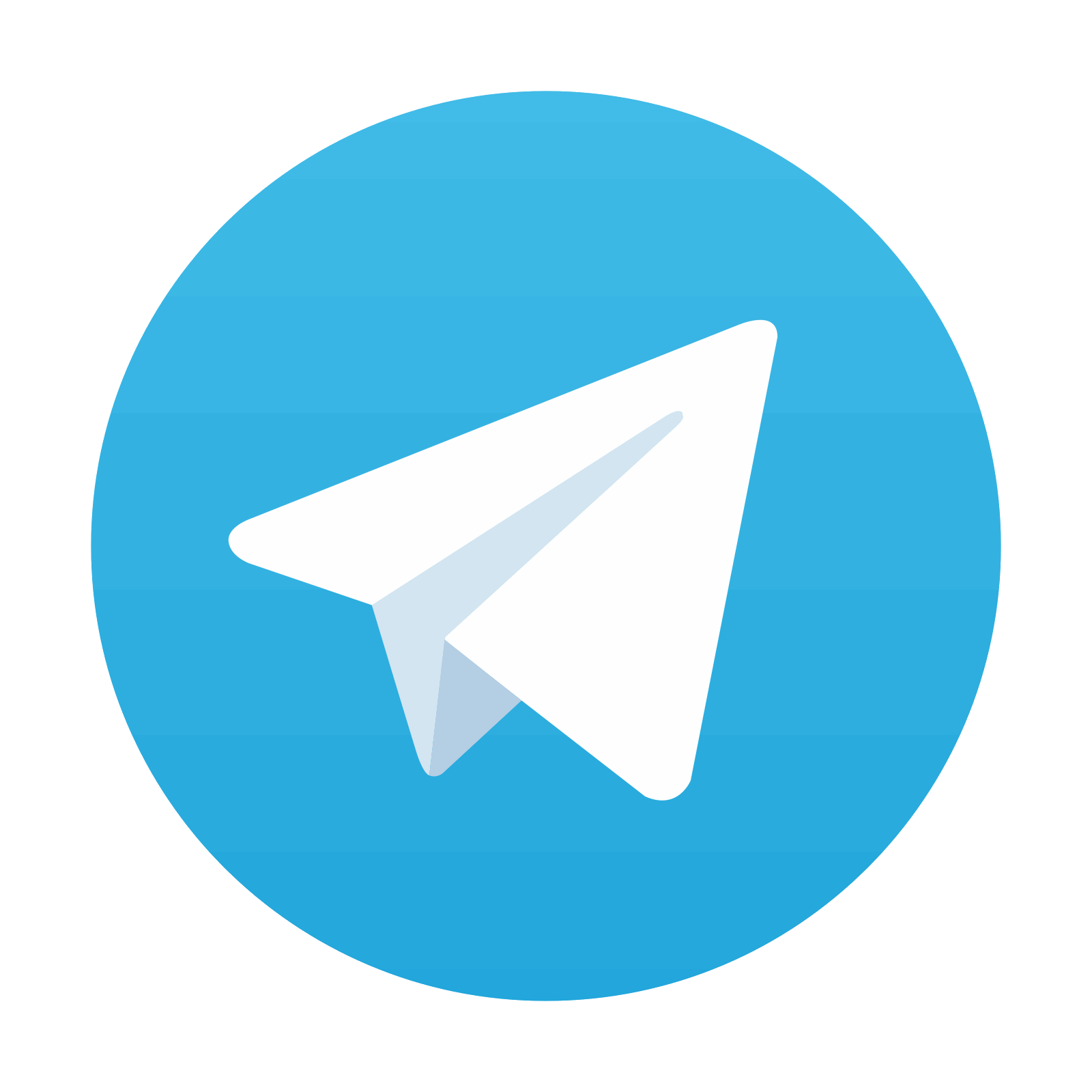
Stay updated, free articles. Join our Telegram channel

Full access? Get Clinical Tree
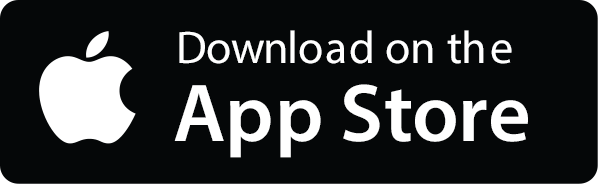
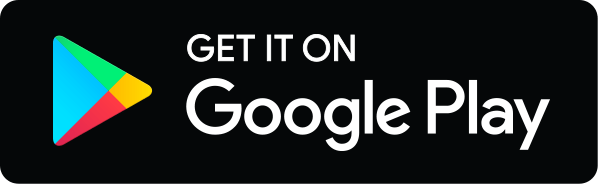