Fig. 16.1
Patent results for the term “cardiac valve” within a US patent database
16.3 Market-Released Cardiac Valves
The most commonly used devices currently available for valve replacement or repair are listed in Table 16.1. It should be noted that detailed explanations for some of these novel percutaneous valve replacement systems are highlighted as the next generation of technologies in Chaps. 7, 8, 9, 10, and 11. Additionally, detailed descriptions of various valve pathologies are described in Chap. 6.
Table 16.1
Examples of market-released cardiac devices used to treat cardiac valve pathologies
Prosthetic valves |
• Mechanical valves |
• Tissue valves |
° Surgically implanted |
° Transcatheter delivered |
Valve annuloplasty rings |
• Surgically implanted |
• Transcatheter delivered |
Valvuloplasty balloons |
In general, the development of surgically implanted prosthetic heart valves for the treatment of valvar stenosis and regurgitation has fallen into two distinct categories: mechanical and tissue valves. Yet, both of these approaches require removal of the diseased valve leaflets and utilize a rigid circular annulus that is sewn into the native valve annulus to support the prosthetic valve. The most common mechanical valve designs employ one of the following: a ball and cage design (such as the Starr-Edwards Silastic ball valve), a tilting disk design (such as the Medtronic Hall valve), or a bileaflet design (such as the St. Jude bileaflet valve). To date, surgically implanted mechanical valves have shown exceptional performance and have also displayed excellent durability. However, patients who have received these implants are required to take anticoagulant medications to minimize the risk of clot formation associated with the biocompatibility and nonphysiological fluid flow properties that often occur with these prostheses. These anticoagulation therapies then impose limits on the patient’s life, as they must always remember to take their medications and avoid activities that could potentially cause bleeding or bruising, including physical contact sports.
To address these challenges, tissue leaflets were subsequently incorporated into replacement valve designs, to better mimic the native cardiac anatomy and minimize the need for anticoagulation requirements. The two most common approaches to this solution include the direct xenotransplantation of a treated porcine valve (such as the Medtronic Mosaic valve) or the construction of leaflets from treated porcine or bovine pericardium (such as the Carpentier-Edwards PERIMOUNT Magna Ease valve). Clinically, the use of tissue valves has been shown to require minimal or no anticoagulation treatments, and currently such valves have exhibited very good performance in humans for 10–15 years. However, their long-term durability—20 years or more—is still under question, and the nature of the material employed within their designs may lead to the possibility that these replacement valves will also elicit calcifications and thus become stenotic again. Note that although both surgically implanted valve technologies show individual merits, they both require highly invasive surgical procedures for successful implantation. Consequently, there has been major efforts to develop percutaneous valve replacement systems, whereby the technological advancements behind surgically implanted tissue valves have been combined with stent designs and delivery technologies. To date, such systems utilize the percutaneous endocardial delivery of a metallic frame, positioned across the valve annulus that, when deployed, replaces the diseased leaflets with tissue leaflets sewn into the center of the frame conduit. Note that these transcatheter-delivered valve replacement therapies are described at length in Chaps. 7, 8, 9, 10, and 11.
Valve annuloplasty rings are almost exclusively used for the treatment of annular dilation and consequent valvar regurgitation of the atrioventricular valves. The majority of annuloplasty products on the market are focused on mitral regurgitation due to the severity of the pathologies in relation to tricuspid regurgitation. Most surgically implanted annuloplasty rings are sewn to the valve annulus, which is saddle-shaped and composed of a dynamic junctional zone of fibrous and muscular tissue between the atrium and ventricle. To accommodate different patient anatomies and physiologies, there are a variety of several ring size, shape, and material property options available to the surgeon, including rigid complete rings (such as the St. Jude Rigid Saddle Ring) and partial flexible bands (such as the Cosgrove-Edwards annuloplasty system) [4]. As with prosthetic valves, there has recently been a push to develop transcatheter-delivered annuloplasty devices to treat mitral valve regurgitation. These devices aim to mimic surgical annuloplasty devices by reducing the mitral annulus in order to achieve better apposition of the native mitral leaflets, utilizing the close proximity of the coronary sinus to the mitral annulus. The advantages and disadvantages of transcatheter-delivered annuloplasty rings are also discussed at length in Chap. 8.
Valvuloplasty balloons have been developed and utilized to treat patients with stenotic heart valves, by inflating a balloon that has been percutaneously positioned across the valve annulus to enlarge the valve opening and improve valve function and blood flow. The technique of balloon dilatation of the stenosed mitral valve was first described in 1984 by Inoue, and was soon adopted into clinical practice replacing older surgical closed valvotomy procedures [5]. These procedures are often used to avoid or delay open-heart surgeries and/or valve replacements.
Again, each of these device categories has been developed to treat a specific form and/or stage of cardiac valvar disease. However, it should be noted that the modern cardiac surgeon or interventionalist will often use a combination of these approaches/devices to treat a given pathology. For instance, a surgeon may utilize a valvuloplasty procedure to open a stenotic valve before delivering a transcatheter aortic valve replacement.
16.4 Typical Anatomy of a Cardiac Device
As noted above, much has recently changed in the fields of cardiac valve replacement and repair. Specifically the improvement of established therapies, the development of novel devices, and the dramatic increase of associated regulatory issues. Currently, in order for any device to be considered acceptable for clinical use, it must demonstrate a number of essential characteristics. The following section will outline the key criteria that need to be fulfilled when developing a cardiac device with a focus on implantable devices.
16.4.1 Functionality
It is vital that any product performs to the standards set by the manufacturer’s claims. Many medical devices are born from the desire to satisfy a previously unmet clinical need. To be successful, a new product must elicit this functionality, at or above the currently available solutions and techniques. The functionality of a cardiac device also encompasses its ability to perform the desired task without compromising any other biological process. For example, a transcatheter-delivered aortic valve replacement should not compromise the function of the mitral valve or the vasculature through which it is delivered to the heart. These specifications are often outlined by regulatory committees such as the International Organization for Standardization (ISO), as seen in Table 16.2 describing the operational specifications for cardiac valve prostheses.
Has reproducible function |
• Allowing forward flow with acceptably small mean pressure difference |
• Preventing retrograde flow with acceptably small regurgitation |
• Remaining fixed once placed |
• Having an acceptable noise level |
• Maintaining its functionality for a reasonable lifetime, consistent with its generic class |
• Maintaining its functionality and sterility for a reasonable shelf life prior to implantation |
Is biocompatible |
• Resisting embolization |
• Resisting hemolysis |
• Resisting thrombus formation |
Is compatible with in vivo diagnostic techniques |
Is deliverable and implantable in the target population |
For implantable cardiac devices such as transcatheter and surgically implanted valves, the question of functionality typically includes the pathway in which the device is delivered. For example, surgically implanted devices require a suitable method of fixation to the heart, such as the sewing ring of a prosthetic heart valve. However, these parameters can be greatly complicated when one chooses to deliver such devices through minimally invasive techniques, such as through the wall of the heart (typically the apex) or through the vasculature without placing the patient on cardiopulmonary bypass. Hence, not only must the delivery system be able to position the device in its intended anatomical location, but additionally it must not induce any adverse effects on the patient. For instance, the patient populations in need of aortic valve replacements will often present with calcified aortic arches. A calcified aorta can be problematic when delivering a device from the femoral artery, since the delivery system must not dislodge the calcifications nor puncture the aorta, both potentially fatal incidents.
Another excellent example of device functionality involves the development of transcatheter-delivered heart valves to treat patients with highly stenotic aortic valves. Note that these technologies are described at length in Chap. 10, along with a discussion of the potential benefits and detriments of device functionality with regard to both the implanting physician and the patient. To date, companies developing these new transcatheter-delivered technologies have been very specific in their description of the patient populations for which the valves are designed, as well as the conditions under which the valves should be implanted. As such, they create extensive documentation of instructions for use (IFU). By doing so, they develop guidelines for acceptable usage of the device and attempt to ensure that the devices are used only in situations that correspond to the conditions under which the device was critically designed to function. Although device manufacturers set guidelines in the IFU regarding how the device is to be utilized, in rare instances a physician may choose to use it otherwise (e.g. use an aortic valve device to replace the pulmonary valve). This action is called off–label use. Off-label use is something that does occur and, in many instances, has saved numerous lives. However, even if a physician has used the device for a particular purpose outside of the IFU, in no way can the device manufacturer encourage or advertise this use unless it has gone through animal and clinical testing for that particular use (see Chap. 17 for additional details regarding preclincial testing).
16.4.2 Biocompatibility
Biocompatibility related to medical devices is generally defined as the ability of a material to perform with an appropriate host response in a specific application [7].
In 1987 Williams described how the biocompatibility of a material can be qualitatively evaluated to assess its relative performance when implanted. The succinctness of this term is often misleading when applying the principles of biocompatibility to cardiac devices, due to the large number of different materials that are commonly used to manufacture such modern devices. For example, tilting disk valve prostheses such as the St. Jude Masters series (St. Jude Medical, MN; Fig. 16.2) are manufactured from a variety of materials including pyrolytic carbon for the disks and Dacron® for the sewing cuff. One needs to consider that each of these materials may elicit different responses from the host body. Hence, they need to be individually assessed and quantified before the cardiac device is implanted. Examples of such tissue responses can be seen in Table 16.3.
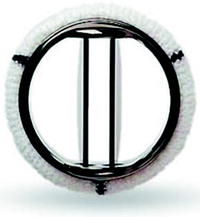
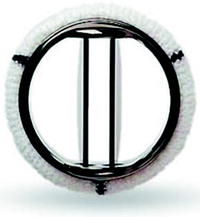
Fig. 16.2
St. Jude Medical bileaflet valve
Adverse local tissue interactions |
• Inflammations |
• Toxicities |
• Carcinogenic responses |
• Calcifications |
• Embolization or lymphatic spread of material fragments |
Induced device migrations: encapsulation or a foreign body response |
Inappropriate or altered healing responses |
Associated infections |
Thrombosis |
• Thrombotic occlusion |
• Thromboembolism |
The mechanisms related to how the host or patient may respond to different components of a cardiac device must be extensively evaluated to ensure that the correct materials are selected for the final device design. This evaluation can be complicated by the fact that it is hard to replicate, in vitro, the range of human immune responses that may exist. To address this, all cardiac devices must also undergo rigorous animal testing, yet it is important to note that this too can sometimes provide misleading results on species-specific bioreactivities. An example of this can be seen in the design and development of the Braunwald-Cutter heart valve ball and cage prostheses (Fig. 16.3), whereby cloth-covered cage struts were designed to encourage endothelialization and subsequently decrease any chance of thrombolytic events [9]. Extensive testing in the mitral position of pigs, sheep, and calves showed promising results, thus the device was approved for human clinical trials. However, the device did not elicit the same host responses when implanted in humans; rather it resulted in aggravated wear on the cloth cage struts and, more critically, the formation of debris embolization. It should also be emphasized that host responses are not limited to immune reactions. For example, implanted tissue heart valves remain susceptible to accelerated prostheses leaflet calcification. More specifically, it has been reported that this type of calcification is initiated by reactions between the extracellular fluid and the leaflet membranes, creating calcium phosphate mineral deposits [10]. As a result, much research is ongoing to enhance leaflet materials and minimize bioreactivities, including calcification inhibitors on the valves that limit mineral deposition on the implanted materials. Furthermore, a device’s material can also display unexpected interactions with the host, as evidenced by the earliest versions of the Starr-Edwards caged-ball mechanical heart valve. The valve design used a silicone ball that was found to absorb lipids from the blood and thus swell [11]. In addition to resultant poor valve function, this also caused the silicone balls to become brittle; in turn, this increased the possibility of ball fracture and consequent embolization of small fragments into the arteries downstream of the valve position.
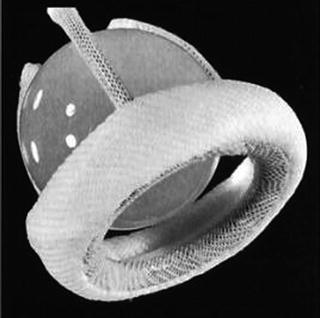
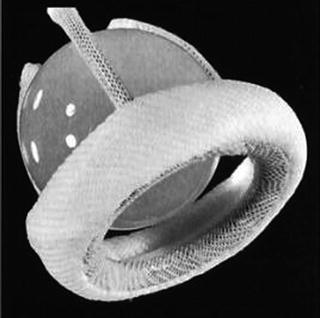
Fig. 16.3
Braunwald-Cutter valve
16.4.3 Durability
As well as exhibiting the appropriate level of biocompatibility, any device implanted into a human heart must also display sufficient durability and longevity. It should be emphasized that this poses a unique problem in the field of implantable cardiac devices, due to the extremely large number of cycles that such prostheses are expected to endure. Furthermore, there are potentially life-threatening implications due to device failures. One needs to consider that the heart is itself a highly dynamic organ which can elicit three to fourfold increases in cardiac output (internal pressures); hence, the mechanical stresses on an implanted device can increase four to sixfold during exercise.
The relative durability of any cardiac device is further challenged by directly interacting with the turbulent and pulsatile flows of blood, which is the case for prosthetic cardiac valves. More specifically, the flow of blood through mechanical prostheses, particularly tilting disk valves, can lead to a particular type of erosion called cavitation whereby gaseous bubbles form and violently collapse on the surface of the valve disks due to the sudden temporary decrease in pressure during closure. This phenomenon has resulted in fractures of the valve disks and was originally observed in the 1980s, in association with the failure of the Edwards-Duromedics valve (Edwards, CA) [12]. Subsequent tilting disk valves, such as the St. Jude bileaflet valve, have been designed to critically reduce this particular material erosion by reducing the area of the tilting disks.
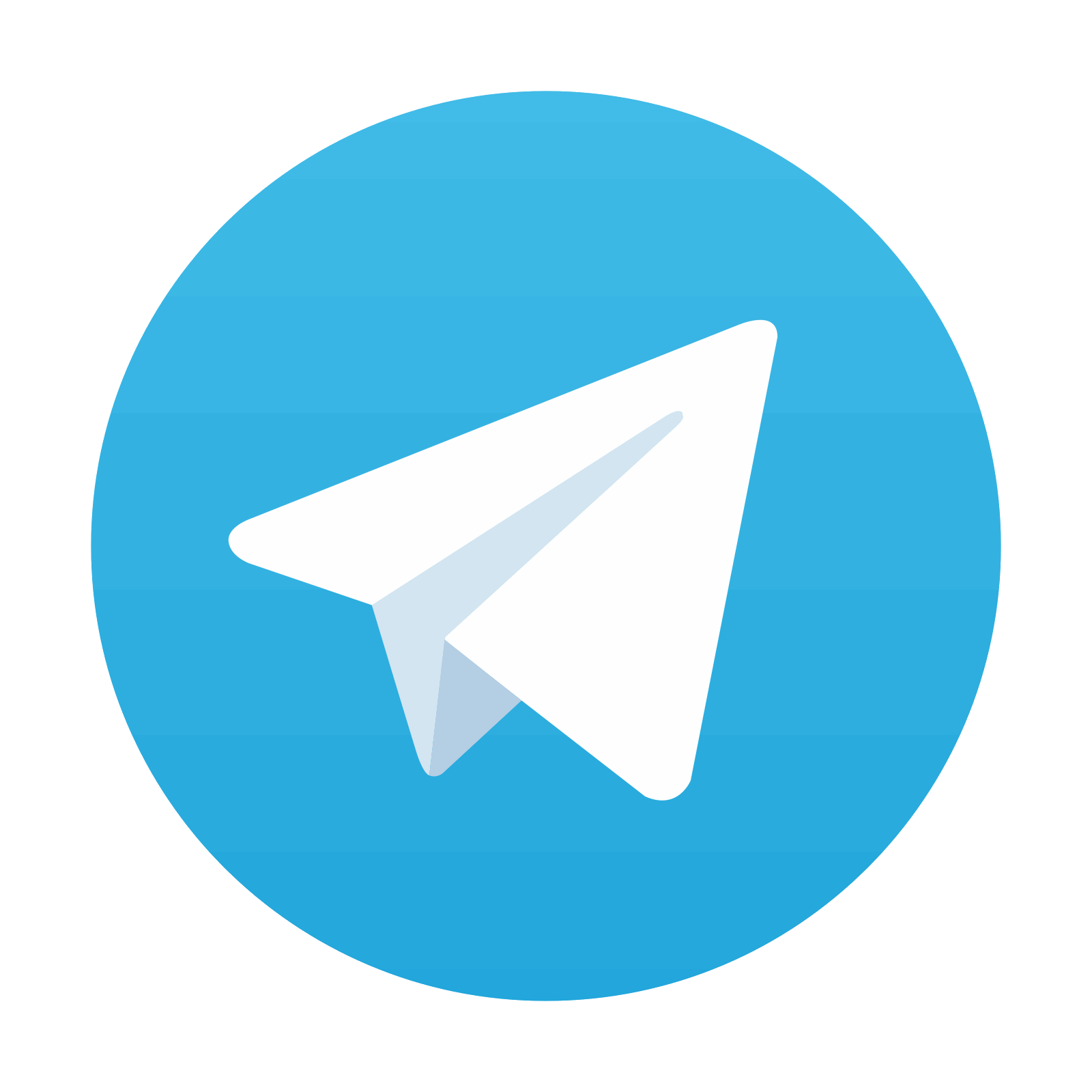
Stay updated, free articles. Join our Telegram channel

Full access? Get Clinical Tree
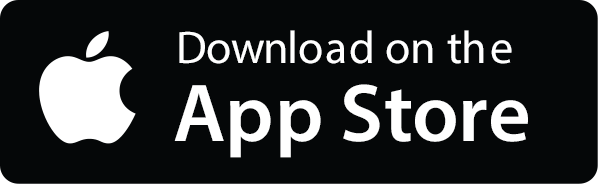
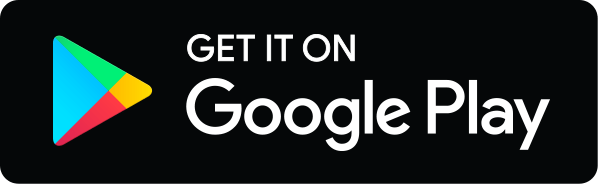