Background
Early detection of right ventricular (RV) failure is required to improve the management of patients with congenital heart diseases. The aim of this study was to validate echocardiography for the early detection of overloaded RV dysfunction, compared with hemodynamic and myocyte contractility assessment.
Methods
Using a porcine model reproducing repaired tetralogy of Fallot, RV function was evaluated over 4 months using standard echocardiography and speckle-tracking compared with hemodynamic parameters (conductance catheter). Sarcomere shortening and calcium transients were recorded in RV isolated myocytes. Contractile reserve (ΔE max ) was assessed by β-adrenergic stimulation in vivo (dobutamine 5 μg/kg) and ex vivo (isoproterenol 100 nM).
Results
Six operated animals were compared with four age- and sex-matched controls. In the operated group, hemodynamic RV efficient ejection fraction was significantly decreased (29.7% [26.2%–34%] vs 42.9% [40.7%–48.6%], P < .01), and inotropic responses to dobutamine were attenuated (ΔE max was 51% vs 193%, P < .05). Echocardiographic measurements of fraction of area change, tricuspid annular plane systolic excursion, tricuspid annular peak systolic velocity (S′) and RV free wall longitudinal systolic strain and strain rate were significantly decreased. Strain rate, S′, and tricuspid annular plane systolic excursion were correlated with ΔE max ( r = 0.75, r = 0.78, and r = 0.65, respectively, P < .05). These alterations were associated in RV isolated myocytes with the decrease of sarcomere shortening in response to isoproterenol and perturbations of calcium homeostasis assessed by the increase of spontaneous calcium waves.
Conclusions
In this porcine model, both standard and strain echocardiographic parameters detected early impairments of RV function and cardiac reserve, which were associated with cardiomyocyte excitation-contraction coupling alterations.
Highlights
- •
Two-dimensional speckle-tracking is a valid tool if compared to the conductance catheter to detect early RV systolic dysfunction.
- •
Despite load dependency, alterations of both echocardiographic standard and strain parameters are consistent with RV and myocyte contractile impairments.
- •
Subclinical alterations of RV systolic standard and strain parameters should be considered as the first sign of RV dysfunction and should require specific management in patients with right heart diseases.
Right ventricular (RV) performance is a major factor in the long-term survival of patients with congenital heart diseases. A reliable noninvasive technique for RV evaluation is essential in managing these patients. Among congenital heart diseases that result in long-term RV dysfunction, repaired tetralogy of Fallot (TOF) is one of the most frequent. Although cardiac magnetic resonance imaging (CMR) provides a good estimation of RV volumes and geometry, CMR could not accurately assess clinical status impairment in adult patients with TOF repair. These observations highlighted the need for more sensitive parameters to detect an early decrease in RV performance to initiate treatments that can affect the progression of RV dysfunction and prevent the development of decompensated RV failure.
In clinical practice, echocardiography is the modality of choice for RV assessment and screening. Developments in echocardiographic techniques with speckle-tracking imaging that assesses strain and strain rate may potentially provide a more sensitive detection of RV dysfunction. Although speckle-tracking has demonstrated its clinical utility for the assessment of left ventricular (LV) dysfunction at an early stage, the accuracy for RV evaluation in pathologic conditions remains questionable. Considering the characteristics of the right ventricle in terms of geometry and mode of contraction, speckle-tracking measurements cannot be achieved with the same level of confidence for the right ventricle as for the left ventricle. The complexity of RV morphology, including geometry and numerous trabeculations, makes it challenging to accurately define endocardial borders for the application of the speckle-tracking algorithm. In recent clinical studies, patients with repaired TOF had decreased RV myocardial deformation, which seemed to be independently associated with CMR-derived RV ejection fraction. These authors have defined thresholds of longitudinal strain for predicting low RV ejection fraction in pediatric and adult populations with TOF repair. Furthermore, speckle-tracking may be helpful to identify the optimal timing for pulmonary valve replacement in patients with TOF repair, which should be performed early to increase the likelihood of complete recovery. To date, speckle-derived parameters assessing RV systolic function have not been validated using the conductance catheter. The conductance technique is the gold-standard method for the evaluation of RV myocardial contractility and function. This was previously demonstrated in experimental models of RV chronic volume and pressure overload and for patients with TOF repair.
To establish correlations between speckle-tracking echocardiography and hemodynamic parameters, an animal model of RV dysfunction is required. Our group validated a porcine model of repaired TOF that presented with early RV dysfunction by chronic combined overload. We used this model to evaluate the accuracy of echocardiographic standard and strain parameters in the detection of early alterations of RV myocardial performance. Because echocardiographic evaluation of RV function is influenced by load conditions, we investigated functional (myocyte contractility and calcium homeostasis) changes at the cardiomyocyte level to support the validity of these echocardiographic parameters to detect myocardial alteration at a subclinical stage.
The objective of this study was to validate echocardiographic standard and strain parameters against hemodynamic and cellular contractility changes for detection of early overloaded RV dysfunction.
Methods
Experimental Design
A surgical procedure mimicking repaired TOF was performed on six Landrace piglets (operated group) that were between 50 and 67 days of age. Six age-matched animals were used as controls (control group). Control animals did not undergo sham surgery. All animals were male to avoid bias related to hormonal variations. Echocardiographic and hemodynamic assessment of RV function were performed at baseline just before the surgical procedure and 4 months later. Clinical status was evaluated daily during the study. After completing the 4-month follow-up period, animals were euthanatized for a cellular study on isolated RV myocytes. All experiments were carried out according to the European Community guiding principles on the care and use of animals (2010/63/UE, September 22, 2010), the local ethics committee (CEEA26 CAPSud) guidelines, and French decree 2013-118 on the protection of animals used for scientific purposes (JORF 0032, February 7, 2013, p. 2199, text no. 24). Authorization to perform animal experiments according to this decree was obtained from Ministère Français de l’Agriculture, de l’Agroalimentaire et de la Forêt (agreement B92-019-01).
Experimental Model of Combined RV Overload
As previously described by our group, after premedication with ketamine hydrochloride (15 mg/kg intramuscularly), general anesthesia was induced with propofol 1% and cisatracurium (0.3 mg/kg every 2 hours), allowing endotracheal intubation, which was maintained with isoflurane in 100% oxygen (Servo 900; Siemens-Elema, Solna, Sweden). Through a left thoracotomy approach, a side-biting vascular clamp was longitudinally placed across the pulmonary valve annulus without obstruction of the RV outflow tract. A pulmonary valve leaflet was excised and the pulmonary infundibulum, annulus, and trunk were enlarged by a 2-cm-long, elliptically shaped polytetrafluoroethylene patch to ensure the loss of valve integrity. This chronic pulmonary valve regurgitation led to RV volume overload. Branch pulmonary arterial obstruction is not uncommon after surgical correction of TOF related to native hypoplasia or surgical complications and is known to increase pulmonary valve regurgitation. In our model, RV pressure overload was achieved by pulmonary artery banding, made of umbilical tape, placed around the artery truncus and secured for a final diameter of approximately 1 cm to ensure progressive pulmonary stenosis with animal maturation.
Echocardiographic Analysis
Echocardiography was performed on closed-chest animals under general anesthesia in the dorsal decubitus position. We used a commercially available Vivid E9 ultrasound machine (GE Medical Systems, Milwaukee, WI) equipped with a 2.5-MHz transducer. The values of all echocardiographic parameters were obtained as the average value of three consecutive cardiac cycles during transient apnea and were analyzed on a comprehensive workstation (EchoPAC 110.1.2; GE Vingmed Ultrasound, Horten, Norway). Measures indexed to body surface area were calculated using the formula body surface area (m 2 ) = (weight [kg] × body length [cm]) 0.5 /60.
In the parasternal long-axis view, RV anterior wall thickness was measured, and the RV/LV end-diastolic diameter ratio was calculated. The tricuspid/mitral annular diameter ratio was measured at the maximal valve opening during diastole in the apical four-chamber view. RV end-diastolic and end-systolic areas were measured in the apical four-chamber view. This allowed the calculation of RV fractional area change (FAC), defined as FAC = [RV end-diastolic area − RV end-systolic area]/RV end-diastolic area. Tricuspid annular plane systolic excursion (TAPSE) was measured as the maximal excursion of the lateral annulus in the apical four-chamber view using M-mode recording through the apex of the right ventricle and the junction between the RV free wall (FW) and the tricuspid annulus. Peak systolic velocity (S′) was measured at the lateral tricuspid annulus using pulsed Doppler tissue imaging. From a parasternal short-axis view, pulmonary annular diameter and transpulmonary gradient through the pulmonary band were both measured using continuous-wave Doppler flow at the maximal pulmonary valve opening during systole. The presence of diastolic flow reversal of color Doppler flow in the main or the branch pulmonary arteries was used to predict severe pulmonary regurgitation.
Regarding the speckle-tracking analysis, RV longitudinal deformation was measured using standard two-dimensional grayscale acquisitions in the apical four-chamber view. All images were recorded at a frame rate of 50 to 80 frames/sec and stored for postprocessing analysis. RV longitudinal peak systolic strain and strain rate were assessed using semiautomatic contouring of the endocardium of the right ventricle with two methods. The first method consisted of contouring of the endocardium of the entire right ventricle (free and septal walls) using six points placed on the image that divided the right ventricle into six standard segments (at the basal, middle, and apical levels). The peak global longitudinal strain (GLS) and global longitudinal strain rate (GLSR) were calculated as the average of the six segments. The second method used a contouring of the endocardium of the FW, excluding the interventricular septum to minimize the influence of the interventricular dependence. The peak global RV FW GLS and GLSR were defined as the average of the three FW segments ( Video 1 available at www.onlinejase.com ). Intra- and interobserver variability was assessed for both FW and RV GLS and GLSR measurements on a random subset of eight pigs. Intraobserver variability was assessed ≥1 week after the initial measurements. Two blinded operators assessed interobserver variability. Echocardiographic assessment of RV myocardial deformation was not studied under stress conditions. Because of the important chronotropic effect obtained with a low dose of dobutamine infusion in both groups, RV speckle-tracking was not feasible.
Hemodynamic Analysis
Under general anesthesia, the conductance catheter (CD Leycom, Zoetermeer, The Netherlands) was inserted into the right ventricle through the femoral vein under fluoroscopic guidance; a balloon was inflated in the caudal vena cava to obtain inflow occlusion. The reference cardiac output was measured using the transpulmonary thermodilution technique (PiCCO; Pulsion Medical Systems, Munich, Germany). This technique allowed us to obtain systemic cardiac output reflecting the efficient pulmonary output independently of the pulmonary regurgitation effect. Data were acquired using dobutamine infusion (5 μg/kg/min) after 10 min of hemodynamic stability. The acquisitions were made during 10 sec of apnea. The pulmonary regurgitation volume was measured from the pressure-volume loops as the increase in RV volume during isovolumic relaxation. Although this measured volume does not represent the total volume of diastolic pulmonary regurgitation, which continues during RV filling, it could provide an accurate estimation of pulmonary regurgitation in patients with TOF. A pulmonary regurgitation volume of >5 mL/beat/m 2 was considered significant. The pulmonary regurgitation fraction was calculated as the ratio between the pulmonary regurgitation volume and the total RV stroke volume. The efficient stroke volume was calculated as the difference between the total stroke volume and the measured volume of the pulmonary regurgitation. The efficient ejection fraction (eEF) was calculated as the ratio between efficient stroke volume and end-diastolic volume. The stroke work corresponded to the pressure-volume loop area. RV contractility was examined using the end-systolic pressure volume relationship. Slopes of end-systolic pressure volume relationship (E max ) were computed during vena cava occlusion. Measurements were acquired at rest and during dobutamine infusion (5 μg/kg/min). The variation of E max slope (ΔE max ) represented the myocardial contractility reserve. In other words, the percentage increase in E max in response to β-adrenergic receptor (β-AR) stimulation reflected the ability of the right ventricle to improve its contractility under stress conditions. An illustration of the changes in shape and characteristics of the RV pressure-volume loop with volume and pressure overload is provided in Supplemental Figure 1 (available at www.onlinejase.com ).
Cellular Study
A detailed methodologic description of the cellular analysis is provided in the supplementary material (available at www.onlinejase.com ). Briefly, animals from both groups were sacrificed at the end of the study by exsanguination. The heart was excised, and the RV myocytes were isolated by enzymatic digestion. Excitation-contraction coupling was characterized on isolated myocytes using the IonOptix system (IonOptix, Milton, MA). This system offers simultaneous measurements of sarcomere length variations using a high-speed contractility camera and cytosolic free Ca 2+ concentration changes using a ratiometric Ca 2+ indicator (Fura-2). Sarcomere shortening and Ca 2+ transient amplitudes and relaxation kinetics were recorded before and after β-AR stimulation by isoproterenol (Iso). Sarcoplasmic reticulum Ca 2+ content (SR-Ca 2+ ) was measured by application of caffeine on isolated myocytes, inducing cytoplasmic Ca 2+ release by activation of reticulum sarcoplasmic ryanodine receptors.
Statistical Analysis
GraphPad Prism 6 (GraphPad Software, La Jolla, CA) and R version 3.1.1 (R Foundation for Statistical Computing, Vienna, Austria) were used for statistical analysis. Clinical, echocardiographic, and hemodynamic data are expressed as medians and interquartile ranges. Nonparametric two-way analysis of variance with paired values using bootstrapping with unrestricted permutation of observations was used to test the significance of changes with time and between groups (D.C. Howel: Manly’s approach). Cellular data were normally distributed (Shapiro-Wilk test) and are expressed as mean ± SEM. Student’s paired t test was used to test the significance of changes between baseline and Iso conditions in each group, and Student’s unpaired t test was used to test the significance of changes between groups. The relationship between echocardiographic and hemodynamic variables was assessed using Spearman correlation. A χ 2 test was used to compare the percentage of cells exhibiting spontaneous calcium waves in RV myocytes. All tests were two tailed, and their level of significance was defined as P < .05.
Results
Clinical Status
The duration of the study was similar between operated and control groups (121 ± 11 and 133 ± 11 days, respectively). Regarding age, weight, body length, heart rate, systemic blood pressure, and central venous pressure ( Table 1 ), groups were similar at each stage. No animals showed clinical signs of heart failure during the study follow-up.
At baseline | At 4 months of follow-up | |||
---|---|---|---|---|
Controls ( n = 4) | Operated ( n = 6) | Controls ( n = 4) | Operated ( n = 6) | |
Clinical characteristics | ||||
Age (d) | 51 (50 to 67) | 55 (50 to 59) | 191 (180 to 197) ∗ | 181 (158 to 190) ∗ |
Weight (kg) | 21.5 (19.5 to 23.6) | 22.8 (19.7 to 25) | 38.9 (36.9 to 39.9) ∗ | 33 (29 to 52) ∗ |
Body length (cm) | 68.5 (67 to 73.8) | 70 (63.9 to 73.3) | 90 (88.5 to 91.5) ∗ | 85 (83.5 to 101.3) ∗ |
BSA (m 2 ) | 0.64 (0.6 to 0.69) | 0.68 (0.59 to 0.71) | 0.98 (0.96 to 1) ∗ | 0.89 (0.82 to 1.2) ∗ |
Heart rate (beats/min) | 92 (85 to 110) | 95 (86 to 96) | 100 (84 to 122) | 102 (85 to 126) |
Systemic pressures (mm Hg) | ||||
Systolic BP | 101.5 (83 to 107.3) | 96.5 (88.3 to 110.3) | 109 (104.3 to 119.3) | 96.5 (92.5 to 121.5) |
Mean BP | 85.5 (62.3 to 92.3) | 77.5 (72 to 92.5) | 97 (90.8 to 100.3) | 83.5 (79.8 to 101) |
SVC pressure | 1 (0.3 to 2.5) | 2 (1 to 4.3) | 3.5 (2.3 to 4) ∗ | 4.5 (2.8 to 7) ∗ |
RV pressures (mm Hg) | ||||
End-diastolic | 1.5 (−1.5 to 3.8) | 3.5 (1.8 to 4.3) | 1 (−0.8 to 4.3) | 6 (4 to 7) † |
End-systolic | 11 (7.3 to 13.3) | 13 (11.8 to 13.3) | 10 (7.3 to 15) | 21.5 (13 to 34) † |
Systolic peak | 17.5 (17 to 23) | 21 (20.8 to 25.3) | 18.5 (15 to 24.3) | 29.5 (22.3 to 40.8) † , ‡ |
Systolic peak/systolic BP | 0.19 (0.17 to 0.22) | 0.23 (0.19 to 0.27) | 0.17 (0.15 to 0.2) | 0.27 (0.24 to 0.39) † , ‡ |
RV volumes | ||||
End-diastolic/BSA (mL/m 2 ) | 131.4 (107 to 134) | 119.7 (109 to 128) | 107.6 (99 to 119) | 148.9 (135 to 155) † , ‡ |
End-systolic/BSA (mL/m 2 ) | 75.1 (51 to 80) | 63.7 (52 to 74) | 56.4 (53 to 58) | 95.8 (80 to 104) ∗ , † , ‡ |
Effective SV (mL) | 34.5 (30.3 to 37.3) | 34 (31.3 to 41) | 47.5 (43.3 to 48.8) | 41 (32 to 53) ∗ |
RV stroke work (mL · mm Hg) | 562 (498 to 663) | 596 (483 to 731) | 732 (496 to 803) | 847 (663 to 1,813) |
∗ P < .05 versus corresponding group at baseline.
† P < .05, operated group versus control group at same step.
‡ P < .05, operated group versus control group, analysis of variance.
Echocardiographic and Hemodynamic Data Confirmed RV Overload
Four months after surgery, operated animals showed significant thickening of the RV FW related to moderate but significant pulmonary stenosis ( Table 2 ). All operated animals had diastolic flow reversal in the branch pulmonary arteries and pronounced RV dilatation, indicating severe pulmonary regurgitation (as shown in Videos 2 and 3 , available at www.onlinejase.com ). The significant increase in the tricuspid/mitral annular diameter ratio, the RV/LV end-diastolic diameter ratio, and both RV end-diastolic and end-systolic areas reflected the RV dilatation ( Table 2 ). The control group displayed normal echocardiographic dimensions during the study ( Table 2 ).
At baseline | At 4 months follow-up | |||
---|---|---|---|---|
Controls ( n = 4) | Operated ( n = 6) | Controls ( n = 4) | Operated ( n = 6) | |
RV dimensions | ||||
RV end-diastolic area/BSA (cm/m 2 ) | 8.8 (8.1–10) | 8.9 (8.3–10.6) | 11 (10.9–11.8) ∗ | 19.9 (18.8–22.2) ∗ , † , ‡ |
RV end-systolic area/BSA (cm/m 2 ) | 5 (4.2–5.8) | 4.9 (4.5–5.8) | 6.2 (6–6.8) ∗ | 12.9 (12.1–14.3) ∗ , † , ‡ |
RV/LV end-diastolic diameter ratio | 0.41 (0.38–0.46) | 0.43 (0.41–0.45) | 0.42 (0.39–0.45) | 0.79 (0.65–0.81) ∗ , † , ‡ |
T/M diameter ratio | 0.83 (0.82–0.85) | 0.83 (0.79–0.86) | 0.77 (0.73–0.84) | 1 (0.97–1.1) ∗ , † , ‡ |
PA annular diameter (cm) | 1.6 (1.5–1.7) | 1.7 (1.5–1.8) | 2 (1.9–2.2) | 2.6 (2.3–2.8) ∗ , † , ‡ |
RV wall thickness (mm) | 3 (2.9–3.3) | 2.9 (2.3–3) | 3.3 (3–3.4) | 5.1 (5–5.4) ∗ , † , ‡ |
Transpulmonary gradient (mm Hg) | ||||
Maximal | 2.8 (2.3–2.9) | 3.7 (2.4–4.3) | 3.7 (2–4.3) | 28.3 (25.2–34) ∗ , † , ‡ |
Mean | 1.5 (1.2–1.6) | 1.7 (1.3–2) | 1.8 (1.2–2.3) | 16.9 (12.4–22.5) ∗ , † , ‡ |
RV systolic function indices | ||||
FAC (%) | 43.6 (41.4–47) | 44.9 (44.7–45.6) | 43.9 (42.7–45.1) | 35.4 (34.6–36.4) ∗ , † , ‡ |
TAPSE (mm) | 17 (16.3–18.5) | 19 (16.5–19.3) | 21 (20–21.8) ∗ | 17.5 (15.5–19) ∗ , ‡ |
Peak S′ (cm/sec) | 7.5 (7–8) | 8.5 (7.8–9.3) | 9.5 (9–10.8) | 7 (6.5–7) † , ‡ |
∗ P < .05 versus corresponding group at baseline.
† P < .05, operated group versus control group at same step.
‡ P < .05, operated group versus control group, analysis of variance.
Regarding RV pressure and volume ( Table 1 ), the two groups were hemodynamically similar at baseline. After 4 months, the hemodynamic assessment confirmed severe pulmonary regurgitation in the operated animals. The median pulmonary regurgitation volume was 12.2 mL/beat/m 2 (interquartile range, 10.6–16.4 mL/beat/m 2 ), and the median pulmonary regurgitation fraction was 25% (interquartile range, 22%–28%). Consequently, end-systolic and end-diastolic volumes were significantly higher at 4 months in the operated animals than in the control group ( Table 1 ) . In the operated group, RV end-systolic pressure significantly increased, and peak pressure rose to a level of one third of systemic pressure at 4 months ( Table 1 ). The pulmonary stenosis was moderate under basal conditions, but it was accentuated after dobutamine infusion, evidence of the increasing RV end-systolic pressure that remained at an infrasystemic level ( Table 3 ).
At baseline | At 4 months follow-up | |||
---|---|---|---|---|
Controls ( n = 4) (Variation %) | Operated ( n = 6) (Variation %) | Controls ( n = 4) (Variation %) | Operated ( n = 6) (Variation %) | |
Heart rate (beats/min) | 151 (140–171) (+64.1) | 153 (143–163) (+61.1) | 138 (129–152) (+38) | 147 (136–152) (+44.1) |
Systemic pressures (mm Hg) | ||||
Systolic BP | 125 (115.5–136) (+23.2) | 139.5 (120–155.8) (+44.6) | 160 (122–182.3) (+46.8) | 156.5 (90.5–183) (+62.2) |
Mean BP | 105 (89.8–117.3) (+22.8) | 115 (100–130.8) (+48.4) | 132 (100.8–149.8) (+36.1) | 133 (74–144.3) (+59.3) |
RV pressures (mm Hg) | ||||
End-systolic | 14.5 (12.3–21.3) (+27.3) | 12 (11–18) (-7.7) | 12.5 (6.5–18.5) (+25) | 49 (29.5–81.8) ∗ , † , ‡ (+128) |
Peak/systolic BP | 0.21 (0.18–0.27) (+10.5) | 0.21 (0.19–0.22) (-8.7) | 0.21 (0.15–0.2) (+23.5) | 0.43 (0.29–1) † , ‡ (+59.3) |
RV stroke work (mL · mm Hg) | 1,011 (971–1,149) (+80) | 954 (721–1,288) (+60.1) | 1,411 (1,340–1,610) (+93) | 3,598 (1,785–5,664) ∗ , ‡ (+325) |
∗ P < .05 versus corresponding group at baseline.
† P < .05, operated group versus control group at same step.
‡ P < .05, operated group versus control group, analysis of variance.
Predominant Volumetric Overload Resulted in Early Alteration of RV Systolic Function
In operated animals, assessment of RV systolic function at 4 months showed a constant cardiac index at rest and under stress ( Figure 1 ). Despite RV dilatation, efficient stroke volume failed to increase ( Table 1 ). The eEF significantly decreased at rest and remained inferior to controls after dobutamine infusion ( Figure 1 ). Stroke work increased 4 months after surgery, without reaching a significant value at rest, but progressed after dobutamine infusion and was significantly higher than in the control group ( Table 3 ). In accordance with our hemodynamic findings of operated animals, echocardiographic measurements of RV FAC, TAPSE, and peak S′ significantly decreased at 4 months and were positively correlated with hemodynamic eEF ( Tables 2 and 4 ).
Coefficient of correlation r | Conductance catheter technique | Echocardiography | ||||
---|---|---|---|---|---|---|
RV EDV | RV ESP | eEF | ΔE max | FW GLS | FW GLSR | |
Echocardiography | ||||||
FAC | −0.6 | −0.82 ∗ | 0.7 ∗ | 0.54 | 0.81 ∗ | 0.78 ∗ |
TAPSE | −0.42 | −0.68 ∗ | 0.67 ∗ | 0.65 ∗ | 0.9 ∗ | 0.76 ∗ |
Peak S′ | −0.44 | −0.72 ∗ | 0.68 ∗ | 0.78 ∗ | 0.88 ∗ | 0.92 ∗ |
FW GLS | −0.37 | −0.83 ∗ | 0.5 | 0.46 | ||
FW GLSR | −0.55 | −0.63 ∗ | 0.67 ∗ | 0.75 ∗ |
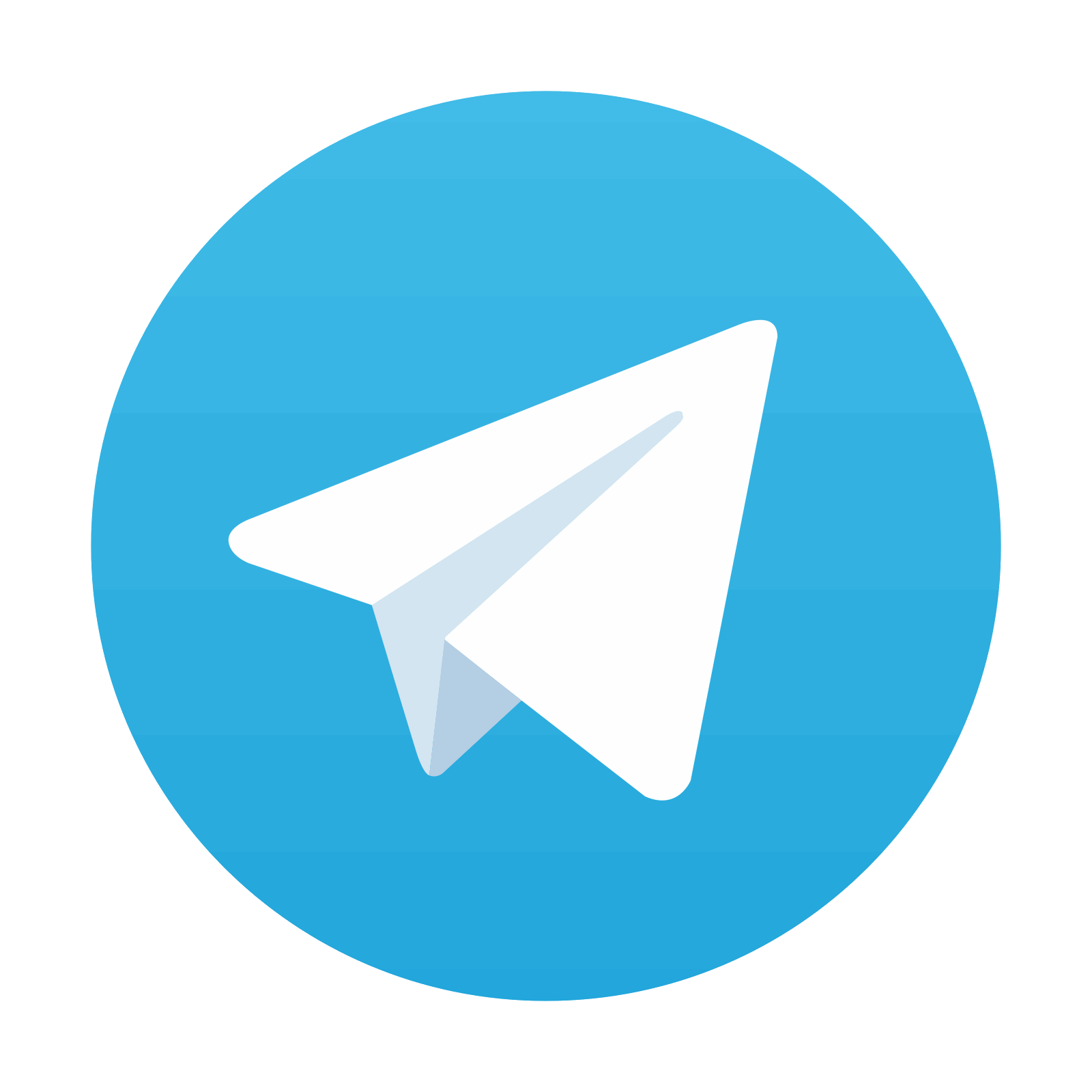
Stay updated, free articles. Join our Telegram channel

Full access? Get Clinical Tree
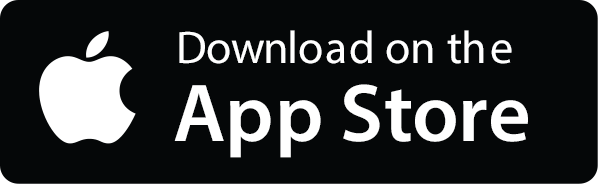
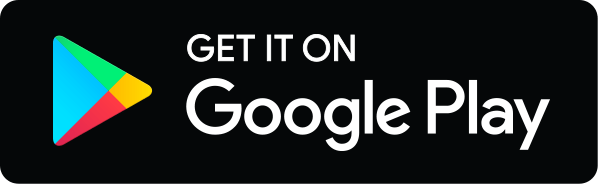
