CHAPTER 9
Stage C: Therapies for Acute Decompensated Heart Failure
A man “about 60. . . his legs were anasarcous, his belly much swelled, and an evident fluctuation of water. His breathing very bad, an irregular pulse, and unable to lie down. His easiest posture was standing with his body leaning over a chair, in which situation he would continue many hours together, labouring for breath, with the sweat trickling down his face very profusely; the urine in very small quantity. Diuretics of every kind I could think of were used with very little or no advantage.”
–William Withering, 17851
Applying the “3 Fs” to Decompensated Heart Failure
Decompensated heart failure is an acute, symptomatic, life-threatening event amplified by neurohumoral activation. The same principles of heart failure assessment at initial presentation also apply to acute decompensated heart failure and guide subsequent management:
Fit: Does the presentation fit the diagnosis of decompensated heart failure?
Function: Is systolic or diastolic function abnormal?
Factors: What factors caused the acute decompensation and are any of them treatable?
FIT
The “fit” with acute decompensated heart failure is supported by history, physical, and chest x-ray findings consistent with congestion or low perfusion. Lab evidence of an elevated natriuretic peptide level can supplement this initial set of findings (see Chapter 2). Vital signs and renal indices help quantitate the severity of the presentation. Oxygen supplementation and assisted ventilation with mask therapies (e.g., BiPAP) can improve hypoxemia. Rarely, respiratory insufficiency requires intubation and mechanical ventilation.
FUNCTION
Echocardiography, if not recently performed, can assess left and right ventricular systolic and diastolic function and associated echo-Doppler findings. Systolic function can dynamically change and findings treated accordingly (see below). In a multicenter OPTIMIZE-HF registry of 41,267 patients admitted with decompensated heart failure, over half (51.2%) had HF-pEF (EF > 40%). In-hospital mortality was 2.9% for HF-pEF versus 3.9% for HF-rEF.2
FACTORS
Decompensated heart failure with an acute coronary syndrome requires special consideration for early revascularization (Figure 9.1). Myocardial ischemia as a contributing factor is suggested by a history of chest pain, abnormal electrocardiogram, or elevated troponin. There are many other factors (List 9.1), and a comprehensive approach to decompensated heart failure involves assessment of etiologies and precipitating factors while stabilizing hemodynamics and fluid balance. Treating precipitating factors associated with circulatory stress, such as infection, may be important to resolve decompensated findings. Identification of patient noncompliance with diet and medication is also important for subsequent prevention of recurrent decompensation.
FIGURE 9.1 The role of coronary artery disease in decompensated heart failure. The presence or absence of coronary artery disease (middle panels) intersects with all four heart failure presentations. (*) Advanced HF is a subset of chronic HF. CAD (†) = coronary artery disease with or without acute coronary syndromes.3 Source: Adapted with permission from Gheorghiade M, Pang PS, J Am Coll Cardiol. 2009;53(7):557-573.
LIST 9.1 Common Factors that Precipitate Hospitalization for Heart Failure
SUBSEQUENT MANAGEMENT
Once the storm of acute decompensation has been calmed, repeat observations of patient status are needed to evaluate the accuracy of initial assessments and efficacy of therapies. Following acute clinical improvement, implementation of evidence-based guidelines improves long-term outcomes (see Chapter 8).
Hemodynamic Profiles in Decompensated Heart Failure
Two conditions are common in acute heart failure: fluid congestion and tissue hypoperfusion. Congestion arising from elevated pulmonary and systemic venous pressures results in dyspnea, orthopnea, jugular venous distension, edema, and ascites. Hypoperfusion with a low cardiac output occurs in more advanced cases or when patients are intravascularly depleted due to over-diuresis or associated dehydrating illness. Hypoperfusion can contribute to fatigue, dizziness, narrow pulse pressure, and renal dysfunction. Therefore, reversing fluid congestion and, when necessary, increasing a depressed cardiac output are essential components for the treatment of decompensated heart failure states.
Stevenson described 4 resting hemodynamic profiles based on the presence or absence, respectively, of hypoperfusion (Cold or Warm) and/or congestion (Wet or Dry) (Figure 9.2).4 Classification of patients with decompensated heart failure as Warm and Wet, Cold and Dry, or Cold and Wet, can then guide therapies to return them to Warm and Dry, which represents the “normal baseline” in compensated heart failure. Most patients with congestion are Warm and Wet and can be treated with intravenous diuretics and oral vasodilators. Patients who are Cold and Dry may respond to cautious rehydration, but may become Wet. Patients who are Cold and Wet are more severely ill and often require intravenous (IV) vasodilator or inotropic drug therapy.4
FIGURE 9.2 Four resting hemodynamic profiles based on congestion and perfusion status used for initial assessment of patients presenting with decompensated heart failure. The Warm and Dry patient (upper left quadrant) is considered “normal” or stabilized with ongoing treatment for heart failure. Percentage in each category based on patients admitted to Brigham and Women’s Hospital with a diagnosis of HF (n = 452).5
FIGURE 9.3 A diuretic treatment algorithm for acute decompensated circulatory congestion.
Volume Management
The majority of decompensated heart failure patients present with fluid overload and pulmonary congestion provoking acute symptoms. Thus, relief of congestion is essential to the management of decompensated heart failure (Figure 9.3). However, diuretics can have both desirable and undesirable effects (Figure 9.4), so optimal dosing is important.
FIGURE 9.4 Effects of diuretics in heart failure.
DIURETIC OPTIMIZATION STRATEGIES EVALUATION (DOSE) STUDY
In the Diuretic Optimization Strategies Evaluation study, patients with acute decompensated heart failure received intravenous furosemide in a 2 × 2 factorial design: via bolus every 12 hours or continuous infusion; and at low-dose or high-dose therapy (Figure 9.5).6 Low-dose therapy consisted of a total daily intravenous dose equal to the previous oral dose. High-dose therapy was 2.5 × previous oral dose. From baseline to 72 hours, high-dose furosemide resulted in greater net fluid loss, weight loss, and improvement in dyspnea (Figure 9.5). However, there was no significant difference in the change of serum creatinine between bolus and continuous infusion, or between high- and low-dose diuretics.6
FIGURE 9.5 Weight loss at 72 hours in the DOSE trial. High dose (2.5 × outpatient loop-diuretic dose) resulted in greater diuresis. Bolus versus continuous intravenous diuretic administration did not lead to a significant difference in diuresis. OP, outpatient; n = 308.6 Source: Adapted with permission from Felker et al., N Engl J Med. 2011;364(9):797-805.
ULTRAFILTRATION
As heart failure progresses, reduced response to diuretics is common.7 Although most patients can be treated with diuretics alone, ultrafiltration can also alleviate excess volume and improve diuretic sensitivity (Figure 9.6).8 Compared with diuretics, ultrafiltration provides a predictable way to achieve euvolemia with minimal electrolyte abnormalities and neurohumoral activation.9 However, when renal function is deteriorating, ultrafiltration may contribute to continued worsening of renal function, and in this setting, high-dose intravenous diuretics supplemented by vasoactive therapy may be more appropriate (see Chapter 10).10
FIGURE 9.6 Diagram of a peripheral venous access ultrafiltration device. In the filtration column the rotary pump creates a pressure gradient for extraction of fluid by the process of convection.11 Source: Adapted from CHF Solutions Inc., Brooklyn Park, MN, with permission.
Vascular access may be from peripheral or central venous catheters. Hematocrit, electrolytes, BUN, and creatinine are monitored every 12–24 hours. To gain predictability of fluid loss and reduce electrolyte abnormalities, loop diuretics can be withheld on days when a patient is receiving ultrafiltration. A nephrology consultation should be considered for patients with marked renal impairment (serum creatinine ≥ 3.0 mg/dL), as dialysis may be a better option to correct volume overload and metabolic abnormalities in these patients.
Effects of Ultrafiltration on Fluid Loss, Electrolyte Balance, and Neurohormonal Activation
Costanzo et al. in the UNLOAD trial observed both greater weight loss and net fluid loss over a 48-hour period with ultrafiltration compared to standard intravenous loop diuretic treatment (Figure 9.7).12 Patients had at least 2 physical findings of fluid congestion and a creatinine less than 3.0 mg/dL. Ninety-day rehospitalization for heart failure, a secondary trial endpoint, was reduced by 50% in the ultrafiltration group.
FIGURE 9.7 Weight loss with ultrafiltration. Mean weight loss at 48 hours with ultrafiltration vs. standard care in patients with decompensated heart failure. Weight loss with ultrafiltration was 5.0 ± 0.68 kg vs. standard care 3.1 ± 0.75 kg. Error bars indicate 95% confidence intervals (CIs).12 Source: Adapted with permission from Costanzo et al., J Am Coll Cardiol. 2007;49(6):675-683.
Ali et al. measured the amount of the electrolytes sodium, potassium, and magnesium in loop diuretic induced urine versus ultrafiltrate in patients with heart failure. Per unit volume, ultrafiltration removed a significantly greater amount of sodium, while reducing the loss of potassium and magnesium (Figure 9.8).13 This difference accounts for a lower incidence of hypokalemia in patients treated with ultrafiltration compared with intravenous diuretics.12
FIGURE 9.8 Electrolyte shifts with ultrafiltration (UF) or with intravenous loop diuretics (IVD). UF removed significantly more sodium and less potassium and magnesium per deciliter (dL) versus loop diuretic–promoted urine (IVD).13 Source: Adapted with permission from Ali et al., Congest Heart Fail. 2009;15(1):1-4.
The type of therapy to remove excess body fluid may have effects beyond the amount of fluid removal alone. Agostoni et al. randomly assigned 16 chronic heart failure patients (NYHA class II–III) to isolated ultrafiltration or intravenous furosemide titrated to 50% decreases in baseline right atrial pressure. Both approaches resulted in similar amounts of fluid loss based on decreases in weight and ventricular filling pressures. Subsequent activation of neurohormonal mediators, however, was greater in the furosemide compared to the ultrafiltration group. Over 3 months, those who received ultrafiltration maintained a reduced body weight, compared to those who received intravenous furosemide (Figure 9.9).9
FIGURE 9.9 Percent changes of circulating levels of norepinephrine, plasma renin activity, and aldosterone (%) and body weight (kg) following one 8-hour ultrafiltration, ( n = 8) or intravenous furosemide ( n = 8) treatment resulting in 50% reductions in right atrial pressure and then followed for 3 months. Symbols: Blue squares, IV furosemide; gold triangles, ultrafiltration. Timepoints on X axis: b, baseline (first and only day of treatment); 0, immediately after ultrafiltration or furosemide treatment; d, days; m, months. Statistical symbols: * P < 0.01 vs. baseline, ◊ P < 0.01 vs. other therapy.9 Source: Adapted with permission from Agostoni et al., Am J Med. 1994;96(3):191-199.
Intravenous Vasoactive Drug Therapy and Acute Heart Failure
Compared to a normal individual, the patient with compensated heart failure has a reduced cardiac reserve for responding to circulatory stressors such as fluid overload, infection, ischemia, or arrhythmia. To treat a decompensated patient with worsening heart failure, short-term intravenous vasoactive therapy may be required. Drugs with vasodilator or positive myocardial inotropic effects can reduce ventricular filling pressures and increase cardiac output beyond the effects from endogenous catecholamines alone. Temporary augmentation of myocardial function may avoid a downhill spiral of respiratory failure, progressive multiorgan dysfunction, and death while the reversible causes of cardiac decompensation are treated. Nevertheless, vasodilator/inotropic therapy should be used cautiously, as it may promote other adverse cardiac events, including arrhythmias, hypotension, and renal dysfunction.14,15
NITRATES AND NITROPRUSSIDE (VASODILATORS)
Similar to oral nitrates, intravenous vasodilator therapy with nitric oxide donors, nitroprusside or nitroglycerin, can improve circulatory function by reducing preload and afterload without direct effect on myocardial contractility.16
Both agents act through increased production of nitric oxide. Nitroprusside generates nitric oxide spontaneously whereas nitroglycerin requires free sulfhydryl groups from the cellular biochemical environment. Nitric oxide activates a soluble guanylate cyclase which catalyses the formation of cyclic guanosine monophosphate (cGMP) from guanosine triphosphate (GTP). Once formed, cGMP acts as an intracellular secondary messenger to decrease intracellular calcium and vascular smooth muscle tone.17
Nitroprusside has a “balanced” action on dilating both arteries and veins. Nitroglycerin’s action is primarily venodilation. Nevertheless, both lead to decreases in mean arterial and venous pressures.18 Nitroglycerin is less likely to shunt blood flow from ischemic myocardium and is preferable in acute myocardial ischemia syndromes, such as heart failure with angina pectoris or myocardial infarction (MI).19
Gaseous nitric oxide acting as a pulmonary vasodilator can be administered as inhaled therapy for the treatment of right heart failure in the setting of increased pulmonary vascular resistance and reduced left ventricular filling pressures. This is generally done in patients receiving mechanical ventilation.
NESIRITIDE (VASODILATOR/DIURETIC)
Nesiritide is an intravenous form of the naturally occurring B-type natriuretic peptide. As such, it has balanced venous and arterial vasodilator properties, in addition to mild renal diuretic effects.
In patients with decompensated heart failure, 3 hours after initiation of therapy, nesiritide compared to intravenous nitroglycerin (median dose 13 mcg/min) was found to have a greater effect to reduce pulmonary capillary wedge pressure and symptoms of dyspnea (Figure 9.10).20 In patients undergoing open-heart surgery, use of nesiritide compared to placebo was associated with less renal dysfunction, a reduced length of stay, and a lower mortality.21
FIGURE 9.10 Comparison of Nesiritide and IV Nitroglycerin. Changes from baseline of pulmonary capillary wedge pressure following placebo, intravenous nitroglycerin, or nesiritide. Asterisk (*) indicates P < 0.05 for nesiritide or nitroglycerin compared with placebo. Dagger (†) indicates P < 0.05 for nesiritide compared with nitroglycerin.20 Source: Adapted with permission from JAMA. 2002;287(12):1531-1540.
A retrospective meta-analysis of trials using nesiritide in acute heart failure, however, suggested that renal function could be impaired with nesiritide.15 Subsequently, a large, 7000-patient trial, ASCEND–HF, did not find an increase in serum creatinine or 30-day mortality compared to placebo.22 A trend to improve dyspnea with the drug did not reach statistical significance in this trial.
When nesiritide is used, the risk of hypotension and associated worsening renal function may be reduced by avoiding doses greater than 0.01 mcg/kg/min, or by omitting a bolus initiation dose.
MILRINONE (INOTROPE/VASODILATOR)
The phosphodiesterase inhibitor, milrinone, acts to increase intracellular cAMP in both heart and vascular smooth muscle cells by blocking its catabolism. This results in both increased cardiac contractility and vasodilatation, which together are effective at reducing elevated left- and right-heart filling pressures and increasing cardiac output in patients with HF-rEF.16 Unlike other intravenous agents used in acute heart failure syndromes, milrinone has a longer biologic half-life of 2–3 hours that is prolonged further with renal insufficiency.23 Because of this, milrinone requires a loading dose to have a rapid onset of action. When stopped, it will persist for hours within the circulation.
< div class='tao-gold-member'>
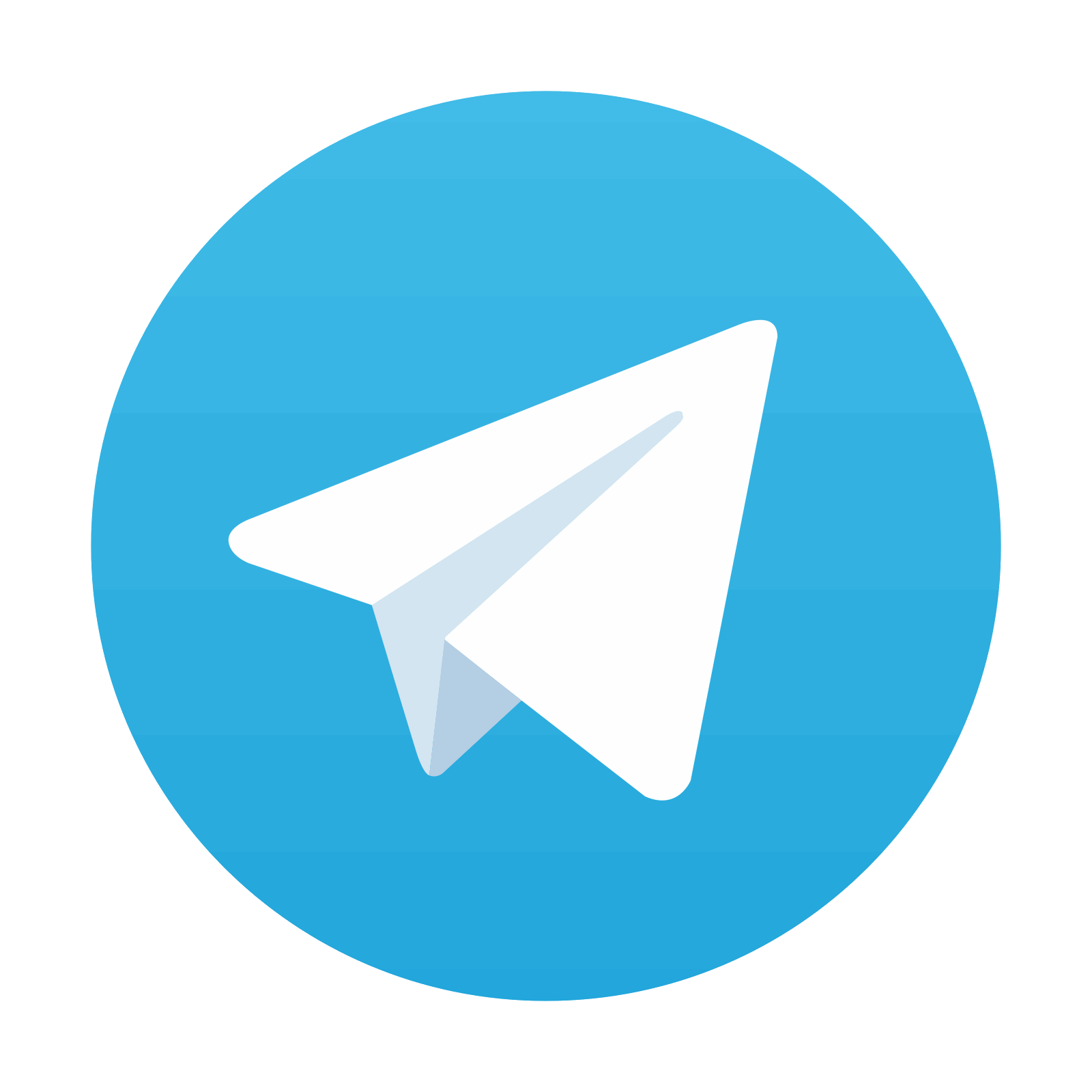