Abstract
Children are usually smaller than adults, but in general ablation techniques used in adults should not simply be miniaturized to fit the size of the pediatric patient. Multiple factors, including the distribution of arrhythmia mechanisms, ongoing myocardial development, and potentially increased risk of vascular injury and AV node damage, as well as the effects of smaller cardiac size, should all influence the ablation technique. An overriding theme in the child should be that safety takes precedence over efficacy, leading to multiple recommendations to consider the use of cryotherapy first in many pediatric cases and conditions. Pediatric patients are more likely than an adult to have the simultaneous presence of structural congenital heart disease, which in itself has a variety of implications for the decision to ablate and the procedural technique. On the other hand, there are numerous similarities between adult and pediatric patients. Specifically, regardless of age, it seems clear that a variety of techniques and approaches are necessary to successfully ablate substrates in all locations of the heart.
Keywords
Ablation, Arrhythmias, Congenital heart disease, Cryoablation, Junctional ectopic tachycardia, Pediatric
Key Points
- •
Children have the same variety of arrhythmia mechanisms as adults, but with a different distribution and often in different clinical settings.
- •
Despite physical similarities of older children to adults, children are not just little adults when it comes to the choice to proceed with and techniques for performing ablation; however, virtually all the techniques used in adults, including epicardial approaches, can be performed in pediatric patients.
- •
Ablation in infants and very small children carries a variety of special risks and should be undertaken only after failure of medical therapy and in the most experienced hands.
- •
The presence of structural congenital heart disease significantly complicates any ablation procedure, demanding that the operator be familiar with both the structural and electrophysiologic (EP) issues at hand, particularly if atrioventricular discordance is present.
- •
Atrial flutter and fibrillation are rare arrhythmias in the pediatric population in the absence of congenital heart disease but may be amenable to catheter-based therapies.
- •
Atrial ectopic tachycardia, junctional ectopic tachycardia, and the permanent form of junctional reciprocating tachycardia can all manifest as incessant tachyarrhythmias with a dilated cardiomyopathy in children. Ablation therapy may be particularly helpful in such cases, if it can be performed safely.
- •
Coronary injury from radiofrequency (RF) ablation can occur when the ablation site is in close proximity to a small coronary artery and is probably unrecognized in most cases where it occurs. Consequently, coronary angiography should be considered before the use of RF ablation of some posteroseptal substrates, particularly in smaller children.
- •
Minimization of fluoroscopy exposure with the use of nonfluoroscopic and lower dose fluoroscopic techniques is feasible and seems prudent during all catheter-based EP procedures in children.
- •
For ablation in children, safety should always take precedence over efficacy. Consequently, when cryotherapy can be effective, it is often the technology of first choice.
- •
The 2016 Pediatric and Congenital Electrophysiology Society/Heart Rhythm Society (PACES/HRS) Expert Consensus Statement on the use of Catheter Ablation in Children and Patients with Congenital Heart Disease is a useful guide for procedural indications and general recommendations in these patient populations.
The explosion in understanding and management of most arrhythmias in adults over the last 30 years is both reflected and amplified in the field of pediatrics. The application of procedures or devices commonly used in the management of arrhythmias in adults remain somewhat delayed in children because of technical issues related to size or lack of regulatory approval. Other issues, such as a smaller number of patients and a higher diversity of clinical characteristics and age, have limited the ability to perform controlled therapeutic trials, even multicenter ones. Despite these limitations, a combination of continued technical developments in the miniaturization of devices, multicenter retrospective reviews and registries, occasional controlled clinical trials, and the use of pharmacologic agents approved by the U.S. Food and Drug Administration for adults has resulted in an equivalence in the armamentarium of adult and pediatric electrophysiologists. However, as is reviewed in this chapter, the parity of tools does not necessarily imply parity of disease and its management.
This chapter concentrates primarily on two categories of rhythm disorders: arrhythmias that are mechanistically similar or identical to those in adults, but whose presentation and management is complicated by the presence of young age or congenital heart disease (CHD), and arrhythmias that are either unique to pediatric patients or are observed only rarely in adults ( Table 40.1 ). Some arrhythmias and their presentations are covered in other chapters and are mentioned here only briefly in the context of differences from the typical adult patient. Although many patients with CHD and arrhythmias are in fact adults, for the purposes of this chapter, the term adult will be used to refer to patients over the age of 18 years, without CHD, and who are not usually cared for by a pediatric cardiologist.
Arrhythmias/Conditions Not Typically Observed in Adults
|
Are Children Just Little Adults?
The notion that children are simply small adults is not entirely inaccurate, particularly when it comes to catheter ablation for some arrhythmias in relatively older and larger children, perhaps after 10 to 12 years of age. However, for many procedures, what seems like only a difference of scale can have dramatic implications, which begin with the diagnosis, extend from the initial recommendation for an ablation to the technical performance of the procedure, and end with the long-term risks of producing radiofrequency (RF) lesions in developing myocardium and near coronary arteries. In addition, the 2016 Consensus Statement on the use of Catheter Ablation in Children and Patients with Congenital Heart Disease included a number safety recommendations that define institutional, procedural, and personnel recommendations for performing catheter ablation in children based on age and patient size. These will be reviewed in detail in the relevant sections of this chapter.
On average, children are obviously smaller than adults, resulting in smaller cardiac chambers, thinner and perhaps more fragile tissues, smaller coronary arteries, and smaller distances between structures, such as the posterior septum and the atrioventricular (AV) node, or the AV ring and the coronary arteries. Not so obviously, children may be dissimilar from adults in ways other than size. Arrhythmia mechanisms overlap but are proportionately very different. Incessant arrhythmias are much more common, probably based on lack of symptom recognition in infants and small children, better early tolerance, and late morbidity and mortality if left untreated, which ensures that most patients will not reach adulthood with their arrhythmias. Accessory pathway (AP) locations are skewed toward the right side, at least partially related to the simultaneous presence of structural CHD. In addition, developing myocardium appears to have the potential for spontaneous cure of an arrhythmia but also the potential for both RF and cryothermal lesion growth with time. Finally, children are generally less cooperative and tolerant than adults, a feature that necessitates special attention in all aspects of the ablation procedure. These issues are discussed in detail in this chapter, along with a review of possible solutions and the implications for management of arrhythmias treatable by catheter ablation in both children and adults.
Arrhythmia Mechanisms
The arrhythmias seen in pediatric patients are typically the more treatable varieties. Ventricular tachycardia is relatively rare in children, accounting for less than 5% of all tachycardias and only 20% of wide-complex tachycardias. In addition, when ventricular tachycardia does occur in a child without structural heart disease, it is more likely than in an adult to have one of the ablatable mechanisms. Supraventricular tachycardia (SVT), which accounts for the vast majority of arrhythmias in children, is most likely to result from a concealed or manifest accessory AV pathway ( Fig. 40.1 ), again portending well for possible catheter ablation therapy. In the absence of a history of surgery for structural CHD, APs probably underlie about 75% of all SVTs in children and account for about 95% of SVTs in neonates, compared with 30% to 40% of SVTs in adults. Atrioventricular nodal reentrant tachycardia (AVNRT) and primary atrial tachycardias (both reentrant and automatic) each appear to account for about half of the remaining SVTs (see Fig. 40.1 ). Atrial fibrillation is seen uncommonly in pediatric patients in the absence of structural or CHD (so-called “lone” AF) and only rarely is problematic enough to justify catheter ablation with pulmonary vein (PV) isolation. However, there are circumstances under which either ablation of an isolated ectopic PV focus or full wide area PV isolation are reasonable approaches. Further, an increasing number of older patients with CHD are developing atrial fibrillation as a consequence of aging and left ventricular dysfunction, leading to a need for more invasive approaches to management.

The Decision to Ablate: Safety Versus Efficacy
One overriding theme in the management of arrhythmias in children compared with adults is an emphasis on safety over efficacy. Although there are few cases at any age in which safety is not an important concern, the relatively benign course of many arrhythmic conditions in childhood, the potential disruption that therapies such as permanent pacing cause for a child, and the fact that parents are usually the decision-making surrogate for the child often lead to a different decision tree for children than for adults. Further, for some situations and technologies (e.g., the potential for coronary damage with RF energy application at the AV groove), the size of the patient and of the heart may be important.
Ablation in patients with Wolff-Parkinson-White (WPW) syndrome is an excellent example of how decision making may be highly age dependent. In this chapter, the term WPW will be used to describe the condition of preexcitation on the surface electrocardiogram (ECG), with or without coexisting tachycardia. Because of a variety of concerns, even the most symptomatic infant with WPW and paroxysmal SVT is only rarely a candidate for ablation. Myocardial injury and potentially severe coronary injury are more likely with RF ablation in this age group. Although the introduction of cryoablation has changed this situation somewhat, there remain a number of reasons to be cautious in managing infants with ablation procedures. Perhaps most important is that about 40% of APs in infants spontaneously stop functioning during the first year of life, and an additional third of patients are unlikely to have symptoms between infancy and early childhood. In children larger than 15 kg who have symptomatic arrhythmias, the balance between risks and benefits clearly shifts toward ablation therapy, but usually only if the ablation can be performed safely. In contrast to the situation in infants, even asymptomatic WPW patients between the ages of 10 and 18 years may be managed more aggressively than adults. Unlike asymptomatic adults older than age 28 years, who are unlikely to ever have symptoms, the older child with a high-risk pathway is exactly the type of patient who may present with sudden arrhythmic death as their initial symptom, leading to the recommendation that such patients should undergo risk stratification, with those who are high risk being offered catheter ablation as a therapeutic option. Further, the guidelines for sports participation in patients with WPW recommend considering risk stratification before approval for this age group. Other age-dependent differences in management decisions are addressed in the discussions of individual arrhythmias.
Use of Cryoablation in Children
Catheter-based cryotherapy was approved for use in the United States in 2003 for ablation of a variety of cardiac arrhythmias. Since then there have been a variety of reports in children, primarily focused on its use in AVNRT and other septal substrates. Cryoablation has several potential advantages over RF ablation, including (1) reversible cryomapping before the production of a permanent lesion; (2) adherence of the catheter tip to the endocardium upon freezing; (3) a well-defined edge of the cryolesion; (4) minimal effects on adjacent coronary arteries; and (5) a lower incidence of thrombus. The first four of these issues are particularly relevant to small children because of the close proximity of a variety of critical cardiac structures to the ablation target and the reported potential for RF lesion growth in immature myocardium. In fact, the most common major complication during RF ablation in pediatric patients is AV block, and there appears to be a higher potential for coronary artery injury in this patient group, even during slow pathway modification (see full discussion later under AVNRT).
Typical cryotherapy systems allow for both ice mapping at a tip temperature of –30 to –40°C where the catheter adheres and nearby tissue loses electrical activity but few cells are killed, and ablation at a tip temperature of less than –65°C where a lesion will be formed. Once cells freeze, they expand and burst. After 4 minutes at the ablation temperature, a typical lesion size is 3 to 6 mm in diameter, smaller than those seen for RF. One of the contrasting features of cryoablation compared with RF is that there is a much larger zone of reversibility as the lesion expands because tissue cooling above the freezing point will lead to loss of electrical activation well before the loss of viability. This feature has dramatically enhanced the safety profile in clinical trials to date. In fact, despite frequent use of the technology for septal tachycardia substrates, there are no reports of AV block with cryoablation, even in children as small as 20 kg, in the presence of a His potential ( Fig. 40.2 ).

The primary disadvantage of cryoablation is that inherent in its high level of safety is a smaller lesion size than for RF ablation. For ablation of septal tachycardia substrates (AV node modification, anterior and posterior septal pathways), cryoablation success rates have been statistically similar to those for RF techniques. However, most operators are less aggressive with RF in septal areas and have had to be highly aggressive with cryotherapy to achieve success. Further, with few exceptions, even aggressive application of cryoenergy has not yielded similar success rates to RF for ablation of nonseptal accessory pathways. Although the data is limited in very small children and infants, anecdotal evidence suggest that cryoablation may be effective for all accessory pathway locations in these unique patient groups. Given the aforementioned considerations, as discussed later for individual arrhythmia substrates, the use of cryoablation is most important for septal substrates, small children, and patients with abnormal anatomy where the precise location of the AV conduction system is not known.
Alternative Presentations or Management of Arrhythmias Commonly Observed in Adults
The complexity of an arrhythmia and its management may result from either the nature of the abnormal rhythm or the setting in which it occurs. The latter aspect is addressed in this section of the chapter, including AVNRT in the child, preexcitation syndromes in the infant and small child, preexcitation syndromes in the patient with CHD, and atrial reentry (flutter or fibrillation) in the pediatric patient without CHD. Atrial and ventricular tachyarrhythmias in the patient with CHD are addressed in Chapter 13 , Chapter 34 , respectively.
Atrioventricular Nodal Reentry Tachycardia in the Child
The issue, addressed earlier, of rebalancing safety and efficacy in the decision-making process for a child is particularly important in the management of AVNRT. A number of factors that are distinctly different in children compared with adults must be considered. These factors are related to particular risk in children that may affect the decision to ablate, the diagnosis of dual AV nodal physiology and AVNRT, and the technical aspects of the ablation procedure.
Medical Management
No natural history data exist for the medical management of AVNRT presenting in childhood. However, at presentation AV node reentry appears easier to manage medically in children than in adults, and particularly in infants.
Atrioventricular Node Physiology in Children
Based on the classic definition for dual AV node physiology—a 50-ms increase in the atrium-to-His bundle (AH) interval for a 10-ms decrement in the atrium-to-atrium (A-A) interval—many fewer pediatric than adult patients with inducible AVNRT have demonstrable dual AV nodal physiology (about 60% vs. 90%–100%, respectively). Because the mechanism of AVNRT induction is similar in children with or without demonstrable dual AV nodal physiology, the presence of two pathways must be assumed. The notion is that the difference in the baseline conduction properties of the two pathways does not reach the threshold for dual physiology in about 40% of children, suggesting that less stringent or more specific criteria may be necessary to define dual AV nodal physiology in children. For instance, the transition from fast to slow pathway conduction may occur with a change in the slope of the AH response to a change in A-A interval, but without a change in AH interval that meets the 50-ms criterion. In fact, a change in conduction pathway could theoretically take place without any change in the AH interval. One might speculate that the magnitude of the AH change at the transition from the fast to the slow pathway would indeed be related to heart size and therefore to age, because normal AV nodal conduction times, expressed as either the AH or the PR interval, increase with age. Younger children have also been shown to have faster conduction in the slow pathway than older children and adults. Consequently, in children, the slope change of AH versus A-A is probably a more reliable and specific measure of the transition between the fast and slow pathways than AH jump criterion alone.
Decision to Ablate and Safety Issues
Once a decision has been made to perform slow pathway modification in a child, identification of appropriate locations for modification is not particularly different from that in adults. Further, the ablation technique and end points for either RF ablation or cryoablation do not vary significantly in children and adults, with the exception that a smaller catheter should generally be used in smaller children (<20 kg or so) to minimize the lesion size. With the use of such techniques, it appears that more than 95% of AVNRT can be eliminated in adults or children. However, two safety issues and their implications should make a significant difference between adults and children in the decision to use RF ablation: the risk of AV block and the risk of coronary damage.
Atrioventricular Block
The possibility of ablation-induced complete heart block deserves special attention in the pediatric patient for two reasons. First, the risk of heart block is theoretically higher in smaller patients, simply because of a number of geometric differences. Because the size of an average RF lesion does not depend on patient size, the typical lesion is relatively large compared with the size of the heart in a smaller patient. The smaller the patient, the closer the AV node is to the area of the slow pathway and to the posterior and anterior septum, common locations for APs. Further, the AV node itself is smaller in small hearts and therefore can be included in a typically sized lesion more easily than in an adult heart. Despite these potential problems, the reported incidence of ablation-induced complete heart block does not appear to be significantly higher in infants and children than in adults. However, a second consideration is the technical details of pacing if heart block occurs. Superior vena cava thrombosis complicating transvenous pacing can occur with both single and dual chamber systems, leading most clinicians to place epicardial pacing systems in children who weigh less than 10 to 20 kg, and only single-chamber transvenous systems in patients weighing under 20 to 25 kg. For this reason, pacing may require chest surgery for epicardial lead placement and may leave the patient physiologically compromised by asynchronous pacing. In addition, the pacing system in a child may need to be maintained for as many as 70 to 80 years, and its presence will almost certainly restrict the child from many competitive sports, thereby setting lifestyle limits that are of less concern for most adult patients. These issues should affect both the decision to ablate and the energy choice for the procedure itself when ablating near the normal AV conduction system, if either the AV node or an AP is targeted. In particular, there are still no reported cases in the literature of permanent AV block with the use of cryoablation; however, a theoretical risk still exists.
Coronary Artery Damage—General Considerations for Atrioventricular Nodal Reentrant Tachycardia and Accessory Pathway Ablation
Acute and late coronary artery injury has been reported after RF ablation for AP-mediated tachycardias in children, adults, and animals. These cases have involved both left- and right-sided coronary arteries, as well as a posterior descending branch of the right coronary artery during ablation of a right posteroseptal AP.
In 2004 we reported a case of coronary damage during slow pathway modification in a 30-month-old, 15.5 kg child with recurrent AVNRT resistant to drug therapy. Approximately 100 seconds after a fourth application of RF energy, ST elevation was noted ( Fig. 40.3 ); it lasted about 15 minutes and was not accompanied by hemodynamic compromise or echocardiographic abnormalities. Selective coronary angiography revealed a dominant right coronary artery giving off a posterior left ventricular branch artery that had an 80% stenosis ( Fig. 40.4 ). The vessel course was within 2 to 3 mm of where the catheter tip had been placed during the last RF application ablation. Acute management was conservative, and after 2 days, repeat angiography revealed some improvement, with an approximately 50% stenosis. Repeat selective right coronary angiography 2 months later revealed complete resolution of narrowing (see Fig. 40.4 ), and repeat electrophysiology studies with and without isoproterenol failed to induce any AVNRT. ST segments remained normal with the isoproterenol infusion. Follow-up off medical therapy has been unremarkable.


Although this case is anecdotal, it reveals several important issues regarding the potential for coronary artery injury during RF ablation in children. First, coronary artery injury may occur with slow pathway ablation for AVNRT. Second, acute coronary artery injury has the potential to be missed and is probably an underreported phenomenon. Third, infants and young children may be at particular risk. The inflammatory component of tissue injury caused by RF energy has been shown to invade layers of the right coronary artery, leading to acute narrowing, when RF energy is applied to the atrial side of the lateral tricuspid annulus in pigs. Further maturation of this injury can result in significant late coronary stenosis. Therefore with RF energy application, coronary stenosis may occur acutely or be delayed. Our patient’s injury was almost missed because ST segment changes did not occur until 100 seconds after the last RF application and resolved spontaneously within minutes, despite a significant persistent stenosis of the involved artery. Other instances of coronary artery injury after RF ablation were also nearly missed because of this delay. In large retrospective and prospective studies in which there were no coordinated attempts to investigate coronary injury after RF ablation, the reported incidences of injury were 0.03% in children and 0.06% to 0.1% in adults. However, in a study in which coronary angiography was performed before and after RF ablation for AP-mediated tachycardias, Solomon et al. reported a 1.3% incidence of coronary artery injury in 70 patients. In fact, we have had an unreported case of 95% occlusion of the posterior descending artery in a 41-kg, 10-year-old patient undergoing ablation of a left posteroseptal AP in the proximal coronary sinus. The stenosis was completely asymptomatic without ECG changes and was discovered only because we performed routine coronary angiography pre- and post-RF ablation for posteroseptal AP locations. Therefore unless evidence for coronary artery injury is actively sought, it will go undiagnosed and underreported.
Smaller children may be at particular risk for coronary injury, because the distance between the ablation catheter and the coronary arteries is significantly less than in adults. Although coronary blood flow probably helps reduce this risk, the flow in small coronaries in any patient may be inadequate to prevent damage. Understanding these factors is critical to preventing injury.
Ablation Energy Cryo Versus Radiofrequency
Although RF energy is the time-honored ablation technology for slow pathway modification to treat AVNRT, over the last 5 years, since the publication of the first edition of this book, the use of cryothermal energy has gained gradual acceptance, particularly in the pediatric population for AVNRT. This growth in acceptance has come from a combination of the safety features of cryoablation discussed in the introductory aforementioned sections, and increasing evidence of equivalent acute success and recurrence rates compared with RF. Earlier reports did find lower acute success rates and higher recurrence rates for AVNRT therapy in both children and adults. However, as experience has accumulated and technology has progressed both acute and long-term results have improved to the point that multiple authors have reported acute success rates above 95% and recurrence rates below 5% with cryoablation, equivalent to those with RF energy. This improvement in outcomes has been caused in part by additional experience with cryo techniques, which appear to have a longer learning curve than that for RF techniques, but also to further refinements in the use of cryothermal energy with the use of additional freeze-thaw-freeze cycles and larger catheter tips. Further, as noted earlier, there are no reports of permanent AV block with cryoablation, despite its most common use being for ablation of septal arrhythmia substrates. The data discussed previously have led to multiple debates at scholarly meetings and conferences on the use of RF versus cryo energy for slow pathway modification in children. Consistent with a formal survey in the literature from 2011, informal surveys at these meetings suggest that despite the higher safety of cryo, because of longer procedure times with cryo and an excellent safety experience with RF, about half of the operators queried continue to use RF as first choice in all larger patients and reserve cryo for smaller patients or specific concerns with RF safety during individual procedures.
Optimal End Point for Slow Pathway Modification in Children
The optimal end point for slow pathway modification ablation in children is not particularly different from that in adults when RF energy is used—a maximum of single AV nodal echo beats. However, when the same end point was used in a large multicenter prospective trial comparing RF to cryoablation in adults, the recurrence rate for cryoablation was more than double that seen in the RF group (9.4% vs. 4.4%, P = .029). Despite some cryoablation studies showing similar recurrence rates with and without complete slow pathway ablation and elimination of dual AV node physiology as a procedural end point, many clinicians do use complete slow pathway ablation as an end point to reduce recurrences. Eliminating sustained SP conduction when it is present before ablation also appears to reduce recurrences. In summary, following RF or cryoablation, the presence of single AVN echo beats in the absence of sustained slow pathway conduction is an acceptable endpoint. My interpretation of available literature along with my personal experience suggest that eliminating all slow pathway conduction as an endpoint, which is safely achievable with cryoablation, helps prevent recurrences.
It is not uncommon that during EP studies performed under general anesthesia in pediatric patients with clinical ECG documented SVT, or palpitations consistent with SVT but without documentation, it is not possible to induce SVT during the procedure. Further, although AVNRT is strongly suspected, it may be difficult to conclusively demonstrate dual AV nodal physiology or AV nodal echo beats. Thus, when indicators of SP conduction are subtle or absent at the start of the case, determining when to terminate the procedure becomes much more challenging. As an example, a single AV nodal echo beat at the end of a case is a much less tolerable end point when this was the only positive finding at the start of the procedure. Multiple reports have demonstrated that anatomic slow pathway modification or complete elimination of all slow pathway conduction are end points that predict clinical success in eliminating recurrences of either documented SVT or sustained palpitations. In such cases, voltage mapping of the AV node region to identify the slow pathway may be useful.
Atrioventricular Nodal Reentrant Tachycardia in Children—Summary and Recommendations
Based on many of the aforementioned discussion points, as of the 2016 Consensus Statement, the indications for ablation to treat AVNRT in larger patients, defined as greater than 15 kg, are very similar to those in adults. In particular, for patients greater than 15 kg, the guidelines provide a class I recommendation: “ablation is recommended for documented SVT, recurrent or persistent, when the family wishes to avoid chronic antiarrhythmic medications” with a Level of Evidence C. However, in smaller patients less than 15 kg, the recommendation is class III if the SVT can be controlled with medication, and only becomes class I after failure of multiple Vaughan-Williams classes of antiarrhythmic medications.
When AVNRT is not inducible at the ablation study, the recommendation is class IIa for slow pathway modification, if there has been clinically documented SVT and there is evidence of dual AV nodal physiology during the study. However, it is class IIb if there are similar findings but without a history of clinically documented SVT. In both of these cases, it is recommended that cryotherapy be considered as first choice for the ablation. These recommendations are based on the data presented earlier that if an operator wishes to absolutely minimize the chance of coronary injury and AV block in children undergoing slow pathway modification for AVNRT, cryoablation is the preferred ablation methodology. Although RF energy is a reasonable choice for patients over 15 kg because of its high safety experience in many centers, cryoablation is strongly recommended for patients under 15 kg. In addition, if RF energy is to be used for children who weigh less than 15 kg ( Table 40.2 ), selective coronary angiography of the artery supplying the posterior septum should be considered before ablation. If a small coronary artery is within 2 to 3 mm of the expected ablation location, RF energy should not be delivered, and if any RF energy is delivered, acute follow-up angiography should be performed after ablation.
|
Preexcitation Syndromes in Infants
There are three special considerations in infants that make management of symptoms secondary to an AP different from those in an older child or adult. First, the risk of a sustained reentrant primary atrial tachycardia, such as atrial fibrillation, is very close to zero in the small, structurally normal heart, making the risk of sudden death in infants with WPW very low. Second, as noted earlier, AP function may spontaneously disappear by 1 year of age. Finally, the known risks of any catheterization, combined with the specific risks of catheter ablation in this age group, suggest that pharmacologic control should be aggressively pursued before ablation is attempted. This last issue deserves further discussion.
In humans, myocardial cell division probably occurs through approximately 6 months of age. Although this finding could potentially protect the myocardium from long-term complications secondary to early injury, ventriculotomy scars produced in newborn puppies, RF ablation lesions in immature lambs, and cryoablation lesions in infant swine appear to increase in size during subsequent development. In addition, in contrast to mature ablation scars from adult animals, late lesions from the neonatal lambs were often histologically invasive and poorly demarcated from the surrounding tissue. The potential clinical importance of these results is underscored by a reported sudden death 2 weeks after an AP ablation in a 5-week-old, 3.2 kg infant. An echocardiogram from the infant at the time of a brief resuscitation, as well as autopsy findings, revealed relatively large lesions extending into the left ventricle from the intended mitral groove ablation site ( Fig. 40.5 ). Another heightened risk in infants is coronary artery damage because of the potentially close proximity of the coronaries to the ablation catheter and the reduced capacity for protective cooling during RF application in any small coronary artery. Although most reports of coronary damage in the literature have been limited to the posterior septum or a nondominant right coronary artery, complete occlusion of the left circumflex artery was reported in a 5-week-old, 5.0 kg infant undergoing RF ablation of a left lateral AP.

Despite these disturbing cases, nonpharmacologic therapy will be necessary in a small subset of infants with AP-mediated tachycardia. Until accurate methods are available to assess lesion size in real time, alternative methodologies should be used in all infants. As discussed previously, data on the effects of cryotherapy suggest that this form of energy is at least less harmful to coronary arteries, even when they are in very close contact, because of the differing effects of cold and heat on connective tissue and the vascular inflammatory response. Coronary artery flow also protects the vessel through local warming during cryotherapy, similar to the way in which blood flow protects through local cooling during RF energy application.
Cryotherapy may offer other advantages for small children. Cryolesions are smaller than RF lesions, contributing to the ability to safely create cryolesions even adjacent to the His bundle. In addition, cryoablation allows for reversible loss of tissue function. In the largest cryoablation clinical study in small children, defined as children less than 15 kg or younger than 5 years of age, cryoablation was found to have a success rate of 74% and recurrence rate of 20%, whereas RF, which was used after failed cryoablation, had a success rate of 81% and a recurrence rate of 30%. Regardless of technique, these outcomes are not as good as in older children; however, importantly no complications were found in the cryoablation alone group, which was statistically lower than the 12.5% major complication rate for the RF after failed cryoablation group.
If technical or other considerations require the use of RF energy, considerable caution should be used. RF lesion size is related to catheter tip size, RF power, tip temperature, and lesion duration. Therefore the following technical modifications should be adopted ( Table 40.3 ): (1) deliver energy in as atrial a location as possible; (2) use a 5 French (5 F) catheter tip; (3) use low temperature mapping (50–55°C) to identify the correct location before higher-temperature RF application; (4) use a lower temperature set point of 60°C for the ablation lesion; and (5) use lesions of shorter duration (7–10 times the time to effect, with a maximum of 30–40 seconds). The future development of real-time ultrasonographic or other modality monitoring of lesion size may also help reduce the procedural risks.
|
The 2016 Consensus Statement provided multiple recommendations related to ablation in infants and small children. Most importantly, based on a variety of data, the document recommends an approximate cutoff of 15 kg to differentiate indications for ablation, recognizing that an exact size or age should not be specified for individual patients. Further, it specifies that catheter ablation in the very smallest children (3–7 kg and <6 months of age) should be reserved for life-threatening arrhythmias, or refractory ones after multiple failed attempts at medical management, which may include various combination therapies. Some of the general recommendations are that ablation should be performed by an experienced pediatric electrophysiologist who undertakes the various strategies to reduce risk described earlier. Further, the use of alternate sources of energy like cryoablation before RF should be strongly considered.
Preexcitation Syndromes in Patients With Structural Congenital Heart Disease
Although it is not strictly a pediatric issue, the combination of structural heart disease and arrhythmia is clearly encountered more often by pediatric electrophysiologists than by others. In agreement with previous studies, a review of this issue in unselected patients with CHD at the Children’s Hospital in Boston found that preexcitation syndromes are statistically increased in patients with Ebstein’s malformation, l-transposition of the great arteries, or hypertrophic myopathy ( Table 40.4 ). Of course, preexcitation also occurs in other patients with CHD, but with an incidence not statistically higher than in the general population.
Lesion | No. of Patients | No. of Patients with WPW Syndrome | Prevalence (%) |
---|---|---|---|
Ebstein | 234 | 21 | 8.97 a |
l -TGA | 588 | 8 | 1.36 a |
HCM | 300 | 3 | 1.00 a |
Pulmonary valve disease | 424 | 2 | 0.47 |
Tricuspid atresia | 458 | 2 | 0.44 |
VSD-membranous | 2659 | 10 | 0.38 |
DORV | 955 | 3 | 0.31 |
HLHS | 666 | 2 | 0.30 |
MVP | 1096 | 3 | 0.27 |
Dextrocardia | 427 | 1 | 0.23 |
Trisomy 21 | 916 | 2 | 0.22 |
TOF-pulmonary atresia | 556 | 1 | 0.18 |
TOF | 2520 | 3 | 0.12 |
AV canal defect | 2058 | 2 | 0.09 |
d -TGA (all) | 2156 | 2 | 0.09 |
Coarctation | 2862 | 1 | 0.04 a |
d-TGA/IVS | 704 | 0 | 0.00 |
Mitral atresia | 417 | 0 | 0.00 |
Total | 20,303 | 66 | 0.33 |
a P < .01 compared with general population estimate of 0.30%.
Anatomy
The association of WPW with Ebstein’s anomaly and with the left-sided tricuspid valve in l-transposition probably has its basis in the embryology of tricuspid valve formation. The leaflets of the AV valves normally develop through a process of undermining or delamination of the interior surface of the embryonic ventricular myocardium. Separation of the atrium from the ventricle occurs through completion of this process and encroachment of fibrous tissue from the AV sulcus. The mitral valve and the anterior leaflet of the tricuspid valve are fully delaminated early in development; however, the posterior and septal leaflets of the tricuspid valve are not even fully formed by 3 months’ gestation. Ebstein’s anomaly appears to occur when there is arrested development of tricuspid valve formation sometime between delamination of the anterior and of the posterior leaflets. The high prevalence of preexcitation combined with anatomic findings of accessory connections in a number of selected cases of Ebstein’s anomaly suggests that the arrested valve development results in remnants of muscular or specialized tissue connections that cross the AV groove. In fact, multiple pathways are common in these patients, often with a combination of a posteroseptal pathway and one or more additional free wall pathways.
Pathophysiology
The electrophysiology of the APs in patients with CHD is not particularly unique. Bidirectional, anterograde-only, and retrograde-only APs have been reported. Further, these patients have the same range of tachyarrhythmias found in patients with structurally normal hearts: orthodromic and antidromic AV reciprocating tachycardias and other SVTs (e.g., AVNRT, atrial flutter/fibrillation) with bystander participation of an anterogradely conducting AP. However, the physiologic and clinical implications of the tachycardia may be markedly different in patients with CHD.
Abnormal hemodynamics, increased incidence of isolated atrial and ventricular ectopy, sometimes poor tolerance of antiarrhythmic therapy, and the need for surgical repair that accompanies CHD all contribute to an increased need for aggressive arrhythmia management in this patient population. However, abnormal anatomy and atypical conduction systems may also enhance the difficulty and risks of either surgical or catheter ablation ( Fig. 40.6 ). Although RF catheter ablation is difficult in these patients, results have been good enough to recommend the procedure in all patients who require subsequent surgical repair to avoid postoperative arrhythmias as a complication, and in most symptomatic patients older than 1 year of age who have significant structural lesions.

A review of the reported cases of RF ablation in patients with CHD revealed that most of the patients had Ebstein’s malformation of a right-sided tricuspid valve. However, a significant proportion had more complex anatomy, with AV valve discordance (right atrium to left ventricle, left atrium to right ventricle—S,L,L or I,D,D) and often heterotaxy. Multiple pathways are extremely common in this group, occurring in 30% to 80% of patients, compared with 5% to 10% of patients without CHD. Like the patients with Ebstein’s malformation, those with AV discordance had all of their APs associated with the tricuspid valve regardless of atrial situs, AV relationship, or valve function. This finding is in contrast to the more random location of APs reported for patients without CHD. Hypertrophic cardiomyopathy is the exception: APs are more likely to be on the normal left-sided mitral valve.
Mapping and Ablation in Patients With Ebstein’s Anomaly
Some aspects of the procedure in patients with Ebstein’s malformation are of special note. First, differentiation of atrial and ventricular signals and precise localization of the AV groove can be difficult, leading to a lack of specificity for what appear to be excellent signals in predicting a successful ablation site. In fact, very early ventricular activations, which might be termed “pseudo” AP potentials can often be seen near the AV groove ( Fig. 40.7 ). This issue is particularly important for older patients who have large hearts with poorly defined AV grooves. The true AV groove is best identified by the right coronary artery. Use of a right coronary electrode wire or guidewire can be considered but may be difficult because of a diminutive right coronary artery, and the wire may need to be in place for long periods if multiple pathways are present. A safer and recommended alternative is continual display of the relevant coronary angiogram using a real-time biplane image storage and display system. As with any AP, searching for balanced atrial and ventricular electrograms during mapping is important. Despite these maneuvers, it may still be very difficult to define the AV groove in these patients, necessitating more test applications of the ablation modality to identify the correct location. Catheter stabilization for free wall pathways in the largest hearts is difficult and is not sufficiently improved through the use of a long sheath or a variety of approaches. The overall issue of patient size is addressed later.

As expected, it appears to be impossible to approach the ventricular side of the tricuspid valve in patients with Ebstein’s malformation. No specific reports have noted the use of nonstandard ablation technologies for these patients, but a few observations can be made. Coronary damage has been reported on multiple occasions in patients with Ebstein’s anomaly, probably because of the thin RV wall and often diminutive right coronary artery. Consequently, despite the tendency to use higher power ablation systems with active or passive cooling for difficult cases, such technologies should be used only if an adequate distance between the catheter tip and the artery has been documented. The definition of adequate here depends on the size of the nearby coronary artery: the larger the size, the safer the ablation. Further, strong consideration should be given to the use of cryotherapy, at least as a mapping tool. Safety is enhanced, and the adhesion of the catheter may be particularly useful in larger patients.
If multiple pathways are present, persistence may be the electrophysiologist’s best weapon for successful ablation. In general, 80% to 90% of patients can be rendered arrhythmia free by the procedure, with relatively infrequent major complications such as permanent AV block. However, recurrence rates have been reported to be as high as 40%, particularly if multiple pathways are present.
Atrioventricular Discordance
Ablation procedures in patients with heterotaxy and/or AV discordance require special considerations. First, detailed echocardiography and angiography are instrumental in defining the complex anatomy of the atria, the AV ring, and the coronary sinus, so that the cameras and catheters can be positioned appropriately (see Fig. 40.6 ). Second, careful attention must be given to locating the normal conduction system. In virtually all patients with AV discordance, the AP has been associated with the tricuspid valve, whereas the His bundle has been associated more closely with the mitral valve. As predicted by Ho and Andersen, the normal conduction axis is often located at an anterior position along the AV groove. Once the “normal” and abnormal conduction fibers are located, electrophysiologic study and RF ablation of the APs can proceed with less risk of damage to the normal conduction system.
Mapping and ablation require detailed knowledge of the anatomy and often innovative approaches. For instance, in cases of atrial inversion (right atrium on the left, or vice versa) with AV discordance (I,D,D), an atrial approach to the right-sided tricuspid valve may require a reverse transseptal procedure from the left-sided inferior vena cava and right atrium to the right-sided left atrium. If present, the coronary sinus in such cases will also be reversed. AVNRT may also be present in these patients, requiring identification of the slow pathway of an AV node that is typically along the anterior mitral annulus. Clearly, the need for a detailed understanding of the anatomy in these cases cannot be overemphasized. Ablation technologies similar to those recommended for patients with Ebstein’s anomaly are applicable to patients with AV discordance as well. Cryoablation may be the referred modality for slow pathway modification to treat AVNRT in these patients.
Double Atrioventricular Nodes
In hearts with normal or inverted atrial situs, but discordant AV connections (S,L,L or I,D,D), the anterosuperior ventricle is a morphologic left ventricle that carries with it the mitral valve. When all four valves are well formed in such hearts, the condition is often referred to as corrected transposition, because the physiologic connections are all correct (i.e., systemic venous return flows to the lungs and pulmonary venous return flows to the aorta). As Anderson and others have described, the AV node in corrected transposition is typically situated superior and anterior in the atrial wall, near the anterolateral quadrant of the mitral valve ( Fig. 40.8A ). The penetrating bundle then runs in the fibrous continuity between the right-sided mitral valve and the anterior cusp of the posterior great artery, eventually linking to the left bundle branch on the right side of the ventricular septum. The right bundle branch then penetrates the ventricular septum to emerge in the inferior left-sided right ventricle. A second AV node that is often present more inferiorly, in the normal area of the triangle of Koch, can also link to the ventricular conduction fibers posteriorly, usually inferior to a ventricular septal defect. If the posterior and anterior ventricular bundle branches link together, a conduction sling ( Fig. 40.8B ), sometimes referred to as a Monckeberg sling , is formed. These anatomic findings provide the substrate for a host of different modes of ventricular excitation or preexcitation and AV reciprocating tachycardias. However, before the publication of a 1994 report, there had been no electrophysiologic documentation of this phenomenon.

In 2001 we reported on seven of these patients, all of whom had AV discordance (2-S,L,L and 1-I,D,D) and characteristics consistent with the diagnosis of two separate AV nodes (twin or double). Five of the seven patients also had a malaligned AV canal defect. The electrophysiologic findings included (1) the existence of two discrete, nonpreexcited QRS morphologies, each with an associated His-bundle electrogram and a normal His-to-ventricle (HV) interval; (2) decremental as well as adenosine-sensitive anterograde and retrograde conduction; and (3) inducible AV reciprocating tachycardia with anterograde conduction over one AV node and retrograde conduction over the other. Ventricular premature beats placed into tachycardia while the His was refractory could preexcite the atrium, indicating that the tachycardia involved two AV connections. In all cases, applications of RF energy at the site of the bidirectional pathway resulted in transient junctional acceleration with an identical QRS morphology to that generated by anterograde conduction over the targeted AV node, and modified or eliminated both anterograde and retrograde conduction at that site. Although there is a possibility that one or the other of these pathways was a Mahaim-type AV fiber, their locations and the presence of near-normal HV intervals, retrograde conduction, orthodromic tachycardia, and junctional-type acceleration during RF ablation all favor a second AV node. The precise etiology may make little difference for the medical management of such patients but is important if damage to the better of the two conduction systems is to be avoided during ablation procedures performed before surgery in these patients with complex anatomy.
One patient with AV discordance and complete common AV canal was particularly illustrative of the features described. Two QRS morphologies were seen during normal rhythm, each with a normal PR interval ( Fig. 40.9 ). One of the QRS morphologies (#1) was more typical of an endocardial cushion defect with a superior axis. At electrophysiology study, QRS morphology #1 could be produced by posterior atrial pacing and had an HV interval of about 50 ms with the His potential found posteriorly in the ventricle ( Fig. 40.10 ). The other QRS morphology (#2) could be produced by anterior atrial pacing and had an associated HV interval (from the posterior His) of only 20 ms, but the QRS appearance did not strongly suggest preexcitation (see Fig. 40.10 ). The anterograde effective refractory period (ERP) of this anterior pathway was approximately 200 ms. Earliest ventricular activation was near the septal remnant regardless of QRS morphology. A regular tachycardia involving (1) QRS morphology #1 (see Fig. 40.10 ); (2) a 1:1 A/V relation; (3) a minimum ventriculoatrial (VA) interval of 70 ms; and (4) earliest retrograde atrial activation at the left anterior AV groove near the midline (tricuspid side of common valve) could be reproducibly induced with ventricular pacing. Ventricular premature beats placed into tachycardia while the His was refractory could preexcite the atrium, indicating that the tachycardia involved two AV connections. Retrograde VA conduction was all via the anterior pathway, was decremental, and had an ERP of 230 ms. Application of RF energy near the point of earliest retrograde conduction (anterior and to the left) resulted in transient junctional acceleration of QRS rhythm #2, followed by a sudden change to a regular slower rhythm of QRS #1 ( Fig. 40.11 ). After RF application, VA conduction was eliminated and tachycardia was noninducible. These findings illustrate the following important features (1) there were two anterograde conduction pathways, one posteroseptal and one anteroseptal; (2) both pathways were on the left (tricuspid) side of the common AV ring; (3) both pathways had slow conduction properties; (4) retrograde conduction occurred over only the anterior pathway; and (5) tachycardia was orthodromic, anterograde posterior, and retrograde anterior.



Clearly, an extensive understanding of the anatomy and electrophysiology should be obtained in such patients before proceeding to mapping and ablation. Further, lack of clarity in defining the anatomy of AV conduction in these patients suggests that ablation should first be undertaken using cryotherapy, proceeding to RF energy only if cryotherapy is unsuccessful or after a recurrence. The one caveat to this recommendation is that low-power RF application may be helpful in identifying the location of the anterior and posterior AV nodes through their acceleration response when heated.
Patient Size and Structural Disease
One observation that is difficult to prove statistically but seems clear from our own experience is that the smaller chamber size in smaller patients with structural heart disease is a technical asset in catheter ablation. In our initial series, a total of seven procedures lasting an average of 4.1 hours were required to ablate seven of nine accessory connections in six patients weighing less than 40 kg, whereas seven procedures lasting an average of 6.5 hours were used to ablate only three of seven connections in four patients who weighed more than 40 kg. Although larger patient size may be useful when the retrograde approach to left-sided accessory connections is used, smaller patient size appears to be helpful for catheter stabilization during an atrial approach, particularly in patients with structural heart disease and potentially very large hearts. Consequently, earlier intervention during childhood is preferable to waiting for near-adult size.
Recurrence Risk
The incidence of recurrence of tachycardia or preexcitation in patients who were initially successfully ablated has been reported to be as high as 40%. Although this recurrence risk is higher than the range of 8% to 12% reported in other large series, the authors did note an increased risk of recurrence with right-sided pathways and with multiple pathways. Both of these conditions occur with increased frequency in this patient population, and, in combination with the complex anatomy, this probably accounts for the high recurrence rate.
Summary
The 2016 Consensus Statement classified ablation as a class I indication for recurrent or persistent SVT related to accessory AV connections or twin AV nodes in CHD patients, as an alternative to medical therapy in patients larger than 15 kg, and when medical therapy is either not effective or associated with intolerable side effects in any patient. The Level of Evidence was B. Ablation for AVNRT was considered class IIa for these patients because of the added risk and consequences of creating inadvertent AV block. Ablation of any tachycardia substrate before planned surgical intervention for CHD was also considered as a class IIa recommendation.
Based on our own observations, the 2016 Consensus Statement and the literature reviewed previously, a few procedural recommendations for catheter ablation in patients with CHD can be made: (1) an attempt should always be made to carefully identify the location of the normal AV conducting system, particularly in patients with AV discordance; (2) the anatomic tricuspid valve is the most likely location for accessory connections; (3) smaller patient size may be an asset; (4) an atrial approach should probably be attempted first for connections around the tricuspid annulus (right- or left-sided); (5) the true AV groove should be well identified, using either atrial and ventricular electrogram balance, a coronary angiogram, or, if available, a coronary mapping wire in larger patients; and (6) consider double AV nodes as the substrate for reentrant tachycardia in patients with AV discordance and malaligned AV canal defects who have two distinct QRS morphologies that both have normal PR intervals. With these caveats in mind, it appears that, despite the difficulties of unusual anatomic landmarks and abnormally positioned conduction systems, most APs in patients with structural CHD can be safely and effectively ablated.
Atrial Flutter or Fibrillation in the Absence of Other Heart Disease
In the pediatric patient, atrial reentry tachycardias are relatively rare in the absence of either structural or functional heart disease. The term lone atrial flutter or fibrillation has been applied here, referring to the isolated nature of the arrhythmia findings. However, both of these tachyarrhythmias are occasionally observed in pediatric patients. There are two age ranges for presentation. Perhaps the most common is during the third trimester of fetal life, when atrial flutter accounts for up to one third of fetal tachycardias, often lasting through delivery and leading to ventricular dysfunction. Neonatal atrial flutter almost universally resolves without recurrence if it can be managed successfully during fetal and early neonatal life. Consequently, ablation therapy for such infants should not be necessary and has never been reported.
A second presentation peak occurs during adolescence, when both atrial flutter and fibrillation may occur in the absence of any identifiable gross structural, hormonal, or chemical cause. However, in one multicenter series of 18 patients ≤ 21 years of age, presenting with atrial fibrillation, seven patients (39%) were noted to have inducible AVNRT or AV reentrant tachycardia involving a concealed AP, all of whom were successfully ablated with no recurrences over a mean follow-up of 1.7 years. Based on this observation, an electrophysiology study with possible ablation should be strongly considered in pediatric patients with lone atrial fibrillation, particularly when recurrent and the initiation has not been documented. In contrast to the case in infants with atrial flutter, fibrillation in this group is likely to recur, 39% of the time in another multicenter study of 42 patients. Consequently, although initial management should be conservative, multiple recurrences in this age group despite medical therapy may lead to a need for ablation therapy similar to the scenario in adults. The use of catheter ablation has been reported for both flutter and fibrillation in young patients. Success rates were greater than 90% for the flutter subgroup in a relatively large series of patients in the Pediatric Ablation Registry. Acute success has also been reported in a few small series of pediatric patients. In one series, seven of eight pediatric patients with paroxysmal fibrillation were managed successfully with either ablation of a single ectopic atrial focus or PV electrical isolation. Further, one of the cases that we included in a series of ablations for patients with ectopic atrial tachycardia (EAT or AET) in 1992 was a 12-year-old boy who presented with recurrent atrial fibrillation that was permanently eliminated after ablation of a single left PV ectopic focus.
Specific technical details for ablation of either atrial flutter or fibrillation in the larger child are not particularly different from those in adults and are not repeated here; however, there are some differences in decision making and approach that may be important. Most importantly, the decision of when to ablate can be quite different, particularly for fibrillation. After conversion from a first episode of one of these arrhythmias, and a paroxysmal SVT substrate is either ruled out or not suspected, either no therapy or a drug to block the AV node response is adequate. After recurrences, the threshold for ablation of atrial flutter can be similar to that in adults.
The use of ablation therapy for the rare cases of atrial fibrillation in pediatric patients is also appealing, but the high emphasis on safety over efficacy for all children mandates that a decision to use RF ablation in this age group be considered only after failure of multiple antiarrhythmic agents. Further, the technique chosen should be the most conservative in terms of safety, because complications such as PV stenosis and stroke can be devastating to a child. Finally, an operator experienced with ablation for atrial fibrillation should be included in the procedure. There are no large reports of such patients, but a reasonable more conservative approach would be to consider looking for single ectopic foci first in individual PVs and limiting the procedure to a minimal wide area circumferential PV isolation with either RF or a cryoballoon. This is a population where coming back for a second procedure should take precedence over a higher risk first procedure.
These general recommendations were formalized in the 2016 Consensus Statement where there were no class I indications for ablation of lone atrial fibrillation in the pediatric age group. Even ablation of a paroxysmal SVT substrate was made class IIa, but a reasonable argument could be made for ablation being first-line therapy in this age group when SVT is the initiating substrate. Empiric wide area circumferential isolation of all PVs in the absence of a documented triggering focus or arrhythmia was considered class IIb, and only if the arrhythmia is multiply recurrent, requiring repeated cardioversion, and medical therapy is either not effective or associated with intolerable side effects. Empiric wide area PV isolation is not recommended (class III) for rare episodes of paroxysmal fibrillation, not requiring cardioversion or well controlled on medical therapy.
Atrial and Ventricular Tachycardias in Patients With Congenital Heart Disease
Atrial and ventricular tachycardias in patients with CHD are covered in Chapter 14 , Chapter 34 .
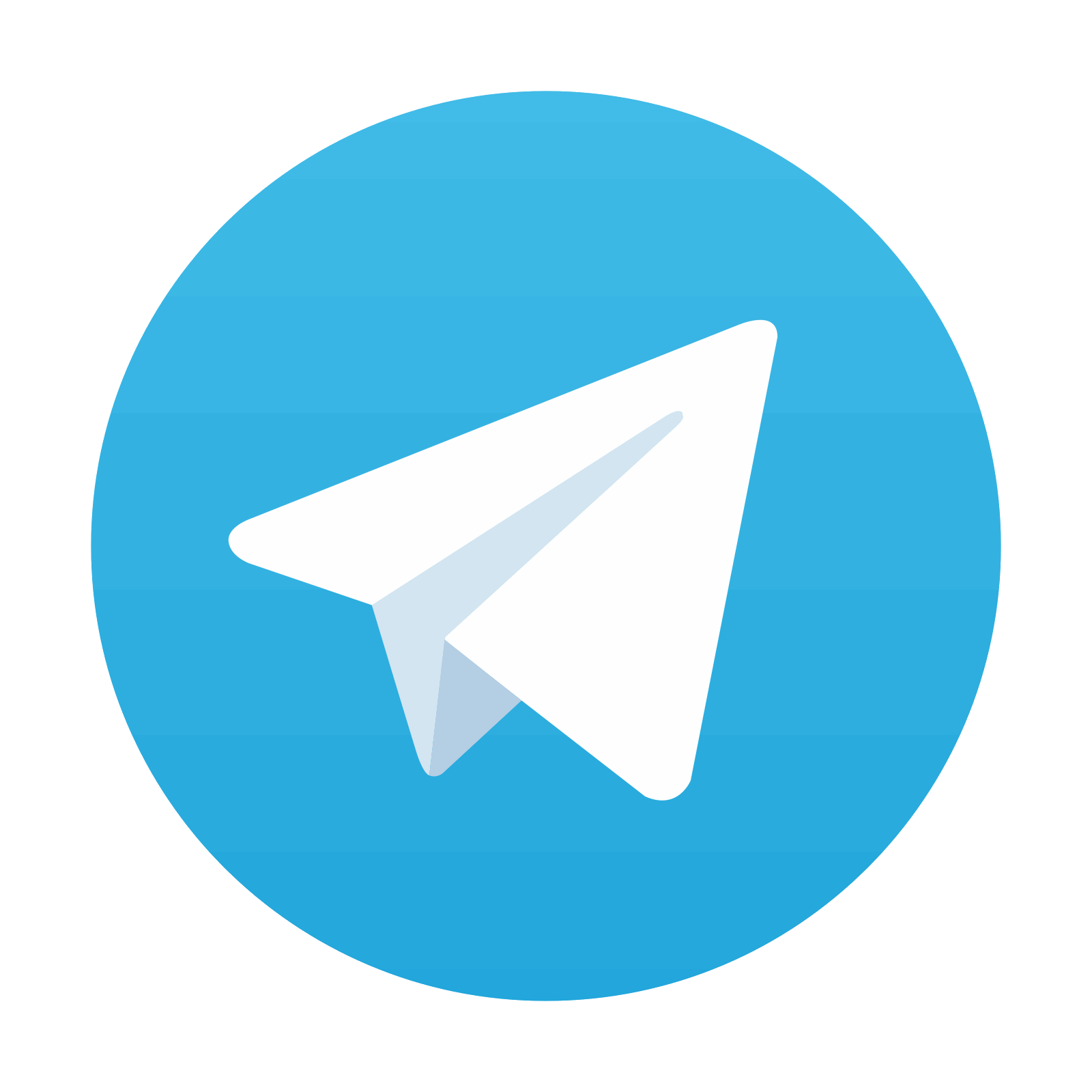
Stay updated, free articles. Join our Telegram channel

Full access? Get Clinical Tree
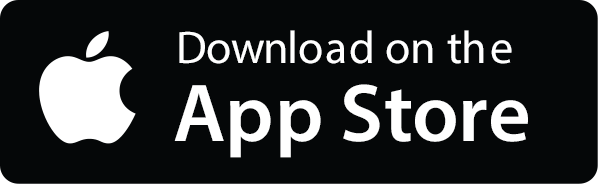
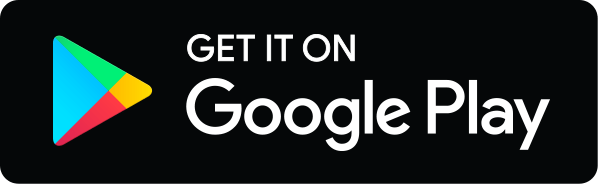