Chapter 50 Small Intestine
Embryology
The primitive gut is formed during the fourth week of fetal human gestation.1 The endodermal layer gives rise to the epithelial lining of the digestive tract and the splanchnic mesoderm surrounding the endoderm gives rise to the muscular connective tissue and all the other layers of the intestine. Except for the duodenum, which is a primitive foregut structure, the small intestine is derived from the midgut. During the fifth week of fetal development, when the intestinal length is rapidly increasing, herniation of the midgut occurs through the umbilicus (Fig. 50-1). This midgut loop has a cranial and caudal limb, with the cranial limb developing into the distal duodenum, jejunum, and proximal ilium and the caudal limb becoming the distal ilium and proximal two thirds of the transverse colon. The juncture of the cranial and caudal limbs is where the vitelline duct joins to the yolk sac. This duct structure normally becomes obliterated before birth; however, it can persist as a Meckel’s diverticulum in approximately 2% of the population. This midgut herniation persists until about 10 weeks of fetal gestation, when the intestine returns to the abdominal cavity. After completing a 270-degree rotation from its initial starting point, the proximal jejunum reenters the abdomen and occupies the left side of the abdomen, with subsequent loops lying more to the right. The cecum enters last and is located temporarily in the right upper quadrant; however, with time, it descends to its normal position in the right lower quadrant. Congenital anomalies of gut malrotation and fixation can occur during this process.
Anatomy
Gross Anatomy
The entire small intestine, which extends from the pylorus to the cecum, measures 270 to 290 cm, with duodenal length estimated at approximately 20 cm, jejunal length at 100 to 110 cm, and ileal length at 150 to 160 cm. The jejunum begins at the duodenojejunal angle, which is supported by a peritoneal fold known as the ligament of Treitz. There is no obvious line of demarcation between the jejunum and the ileum; however, the jejunum is commonly considered to make up the proximal two fifths of the small intestine and the ileum makes up the remaining three fifths. The jejunum has a somewhat larger circumference, is thicker than the ileum, and can be identified at surgery by examining mesenteric vessels. In the jejunum, only one or two arcades send out long, straight vasa recta to the mesenteric border, whereas the blood supply to the ileum may have four or five separate arcades with shorter vasa recta (Fig. 50-2). The mucosa of the small bowel is characterized by transverse folds (plicae circulares), which are prominent in the distal duodenum and jejunum.
Neurovascular-Lymphatic Supply
The small intestine is served by rich vascular, neural, and lymphatic supplies, all traversing through the mesentery. The base of the mesentery attaches to the posterior abdominal wall to the left of the second lumbar vertebra and passes obliquely to the right and inferiorly to the right sacroiliac joint. The blood supply of the small bowel, except for the proximal duodenum, which is supplied by branches of the celiac axis, comes entirely from the superior mesenteric artery (Fig. 50-3). The superior mesenteric artery courses anterior to the uncinate process of the pancreas and the third portion of the duodenum, where it divides to supply the pancreas, distal duodenum, entire small intestine, and ascending and transverse colons. There is an abundant collateral blood supply to the small bowel provided by vascular arcades coursing in the mesentery. Venous drainage of the small bowel parallels the arterial supply, with blood draining into the superior mesenteric vein, which joins the splenic vein behind the neck of the pancreas to form the portal vein.
Microscopic Anatomy
The small bowel wall consists of four layers, the serosa, muscularis propria, submucosa, and mucosa (Fig. 50-4).
The mucosa can be divided into three layers, the muscularis mucosae, lamina propria, and epithelial layers (Fig. 50-5). The muscularis mucosae is a thin layer of muscle that separates the mucosa from the submucosa. The lamina propria is a connective tissue layer between the epithelial cells and muscularis mucosae that contains a variety of cells, including plasma cells, lymphocytes, mast cells, eosinophils, macrophages, fibroblasts, smooth muscle cells, and noncellular connective tissue. The lamina propria, the base on which the epithelial cells lie, serves a protective role in the intestine to combat microorganisms that penetrate the overlying epithelium, secondary to a rich supply of immune cells. Plasma cells actively synthesize immunoglobulins and other immune cells in the lamina propria and release various mediators (e.g., cytokines, arachidonic acid metabolites, histamines) that can modulate various cellular functions of the overlying epithelium. The epithelial layer is a continual sheet of epithelial cells covering the villi and lining the crypts. The main functions of the crypt epithelium are cell renewal and exocrine, endocrine, water, and ion secretion; the main functions of the villous epithelium are digestion and absorption. Four main cell types are contained in the mucosal layer: (1) goblet cells, which secrete mucus; (2) Paneth cells, which secrete lysozyme, tumor necrosis factor (TNF), and the cryptidins, which are homologues of leukocyte defensins thought to be related to the host mucosal defense system; (3) absorptive enterocytes; and (4) enteroendocrine cells, of which there are more than 10 distinct populations that produce the gastrointestinal hormones.
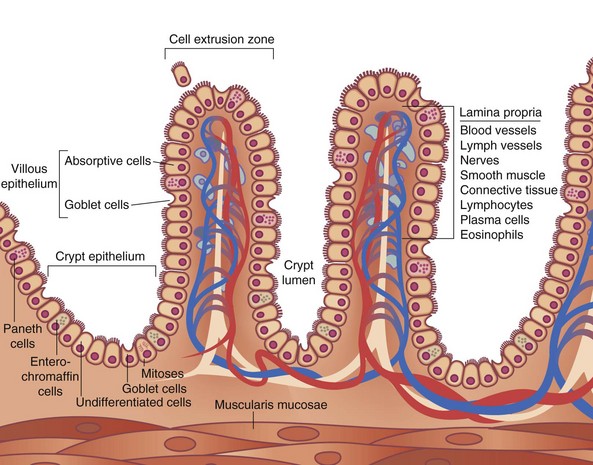
FIGURE 50-5 Schematic diagram of the histologic organization of the small intestinal mucosa.
(Adapted from Keljo DJ, Gariepy CE: Anatomy, histology, embryology, and developmental anomalies of the small and large intestine. In Feldman M, Scharschmidt BF, Sleisenger MH [eds]: Sleisenger & Fordtran’s gastrointestinal and liver disease: Pathology, diagnosis, management, Philadelphia, 2002, WB Saunders, p 1646.)
Physiology
Digestion and Absorption
The complex process of digestion and eventual absorption of nutrients, water, electrolytes, and minerals is the main role of the small intestine. Liters of water and hundreds of grams of food are delivered to the small intestine daily and, with remarkable efficiency, almost all food is absorbed, except for indigestible cellulose. The stomach initiates the process of digestion with the breakdown of solids to particles 1 mm or smaller, which are then delivered to the duodenum, where pancreatic enzymes, bile, and brush border enzymes continue the process of digestion and eventual absorption through the small intestinal wall.2 The small bowel is primarily responsible for the absorption of the dietary components (carbohydrates, proteins, and fats), as well as ions, vitamins, and water.
Carbohydrates
An adult consuming a normal Western diet will ingest 300 to 350 g of carbohydrates a day, with about 50% consumed as starch, 30% as sucrose, 6% as lactose, and the remainder as maltose, trehalose, glucose, fructose, sorbitol, cellulose, and pectins.2 Dietary starch is a polysaccharide consisting of long chains of glucose molecules (Fig. 50-6). Amylose makes up about 20% of starch in the diet and is broken down at the α-1,4 bonds by salivary (i.e., ptyalin) and pancreatic amylases that convert amylose to maltotriose and maltose. Amylopectin, making up about 80% of dietary starch, has branching points every 25 molecules along the straight glucose chains; the α-1,6 glucose linkages in amylopectin produce the end products of amylase digestion—maltose, maltotriose, and the residual branch saccharides, the dextrins. In general, the starches are almost totally converted into maltose and other small glucose polymers before they have passed beyond the duodenum or upper jejunum. The remainder of carbohydrate digestion occurs as a result of brush border enzymes of the luminal surface.
The brush border of the small intestine contains the enzymes lactase, maltase, sucrase-isomaltase, and trehalase, which split the disaccharides, as well as other small glucose polymers, into their constituent monosaccharides (Table 50-1). Lactase hydrolyzes lactose into glucose and galactose. Maltase hydrolyzes maltose to produce glucose monomers. Sucrase-isomaltase is a complex of two subunits; sucrase hydrolyzes sucrose to yield glucose and fructose, and isomaltase hydrolyzes the α-1,6 bonds in α-limit dextrins to yield glucose. Glucose represents more than 80% of the final products of carbohydrate digestion, with galactose and fructose usually representing no more than 10% of the products of carbohydrate digestion.
Table 50-1 Characteristics of Brush Border Membrane Carbohydrases
ENZYME | SUBSTRATE | PRODUCTS |
---|---|---|
Lactase | Lactose | Glucose |
Galactose | ||
Maltase (glucoamylase) | α-1,4-linked oligosaccharides, up to nine residues | Glucose |
Sucrase-isomaltase (sucrose-α -dextrinase) | ||
Sucrase | Sucrose | Glucose |
Fructose | ||
Isomaltase | α-Limit dextrin | Glucose |
Both enzymes | α-Limit dextrin | |
α-1,4-link at nonreducing end | Glucose | |
Trehalase | Trehalose | Glucose |
From Marsh MN, Riley SA: Digestion and absorption of nutrients and vitamins. In Feldman M, Sleisenger MH, Scharschmidt BF (eds): Sleisenger and Fordtran’s gastrointestinal and liver disease: Pathophysiology, diagnosis, management, vol 2, Philadelphia, 1998, WB Saunders, p 1480.
The carbohydrates are absorbed in the form of monosaccharides. Transport of the released hexoses (glucose, galactose, and fructose) is by specific mechanisms involved in active transport. The major routes of absorption are by three membrane carrier systems—sodium glucose transporter 1 (SGLT-1), glucose transporter 5 (GLUT-5), and glucose transporter 2 (GLUT-2)2 (Fig. 50-7). Glucose and galactose are absorbed by a carrier-mediated active transport mechanism, which involves the cotransport of Na+ (SGLT-1 transporter). As Na+ diffuses into the inside of the cell, it pulls the glucose or galactose along with it, thus providing the energy for transport of the monosaccharide. The exit of glucose from the cytosol into the intracellular space is predominantly by a Na+-independent carrier (GLUT-2 transporter) located at the basolateral membrane. Fructose, the other significant monosaccharide, is absorbed from the intestinal lumen through a process of facilitated diffusion. The carrier involved in fructose absorption is GLUT-5, which is located in the apical membrane of the enterocyte. This transport process does not depend on Na+ or energy. Fructose exits the basolateral membrane by another facilitated diffusion process involving the GLUT-2 transporter.
Protein
Protein digestion is initiated in the stomach, where gastric acid denatures proteins.2 Digestion is continued in the small intestine, where the protein comes into contact with pancreatic proteases. Pancreatic trypsinogen is secreted in the intestine by the pancreas in an inactive form but becomes activated by the enzyme enterokinase, a brush border enzyme in the duodenum. Activated trypsin then activates the other pancreatic proteolytic enzyme precursors. The endopeptidases, which include trypsin, chymotrypsin, and elastase, act on peptide bonds at the interior of the protein molecule, producing peptides that are substrates for the exopeptidases (carboxypeptidases), which serially remove a single amino acid from the carboxyl end of the peptide (Table 50-2). This results in splitting the complex proteins into dipeptides, tripeptides, and some larger proteins, which are absorbed from the intestinal lumen by an Na+-mediated active transport mechanism and digested further by enzymes in the brush border and in the cytoplasm of the enterocytes (Fig. 50-8). These peptidase enzymes include aminopeptidases and several dipeptidases, which split the remaining larger polypeptides into tripeptides and dipeptides and some amino acids. The amino acids, dipeptides, and tripeptides are easily transported through the microvilli into the epithelial cells where, in the cytosol, additional peptidases hydrolyze the dipeptides and tripeptides into single amino acids; these then pass through the epithelial cell membrane into the portal venous system. In normal humans, digestion and absorption of protein are usually 80% to 90% completed in the jejunum.
Table 50-2 Principal Pancreatic Proteases
ENZYME | PRIMARY ACTION |
---|---|
Endopeptidases | Hydrolyze interior peptide bonds of polypeptides and proteins |
Trypsin | Attacks peptide bonds involving basic amino acids; yields products with basic amino acids at carboxyl-terminal end |
Chymotrypsin | Attacks peptide bonds involving aromatic amino acids, leucine, glutamine, and methionine; yields peptide products with these amino acids at carboxyl-terminal end |
Elastase | Attacks peptide bonds involving neutral aliphatic amino acids; yields products with neutral amino acids at carboxyl-terminal end |
Exopeptidases | Hydrolyze external peptide bonds of polypeptides and protein |
Carboxypeptidase A | Attacks peptides with aromatic and neutral aliphatic amino acids at carboxyl-terminal end |
Carboxypeptidase B | Attacks peptides with basic amino acids at carboxyl-terminal end |
From Castro GA: Digestion and absorption. In Johnson LR (ed): Gastrointestinal physiology, St Louis, 1991, Mosby, pp 108-130.
Fats
Emulsification
Most adults in North America consume 60 to 100 g/day of fat. Triglycerides, the most abundant fats, are composed of a glycerol nucleus and three fatty acids; small quantities of phospholipids, cholesterol, and cholesterol esters also are found in the normal diet. Essentially all fat digestion occurs in the small intestine, where the first step is the breakdown of fat globules into smaller sizes to facilitate further breakdown by water-soluble digestive enzymes, a process termed emulsification.2 This process is facilitated by bile from the liver, which contains bile salts and the phospholipid lecithin. The polar parts of the bile salts and lecithin molecules are soluble in water, whereas the remaining portions are soluble in fat. Therefore, the fat-soluble portions dissolve in the surface layer of the fat globules and the polar portions, projecting outward, are soluble in the surrounding aqueous fluids. This arrangement renders the fat globules more accessible to fragmentation by agitation in the small intestine. Therefore, a major function of bile salts, and especially lecithin in the bile, is to allow the fat globules to be readily fragmented by agitation in the intestinal lumen. With the increase in surface area of the fat globules resulting from the action of the bile salts and lecithin, the fats can now be readily attacked by pancreatic lipase, the most crucial enzyme in the digestion of triglycerides, which splits triglycerides into free fatty acids and 2-monoglycerides.
Intracellular Processing
The monoglycerides and free fatty acids, which are dissolved in the central lipid portion of the bile acid micelles, are absorbed through the brush border because of their highly lipid-soluble nature and simply diffuse into the interior of the cell.2 After disaggregation of the micelle, bile salts remain within the intestinal lumen to enter into the formation of new micelles and act to carry more monoglycerides and fatty acids to the epithelial cells. The released fatty acids and monoglycerides in the cell re-form into new triglycerides. This re-formation of a triglyceride occurs in the cell through the interactions of intracellular enzymes that are associated with the endoplasmic reticulum.
Enterohepatic Circulation
The proximal intestine absorbs most of the dietary fat. Although the unconjugated bile acids are absorbed into the jejunum by passive diffusion, the conjugated bile acids that form micelles are absorbed in the ileum by active transport and are reabsorbed from the distal ileum. The bile acids then pass through the portal venous system to the liver for secretion as bile. The total bile salt pool in humans is 2 to 3 g; it recirculates about six times every 24 hours (the enterohepatic circulation of bile salts).2 Almost all the bile salts are absorbed, with only about 0.5 g lost in the stool every day; this is replaced by resynthesis from cholesterol.
Water, Electrolytes, and Vitamins
Eight to 10 liters of water/day enter the small intestine. Much of this is absorbed, with only approximately 500 mL or less leaving the ileum and entering the colon2 (Fig. 50-9). Water may be absorbed by the process of simple diffusion. In addition, water may be drawn in and out of the cell through a process of osmotic pressure, resulting from active transport of sodium, glucose, or amino acids into cells.
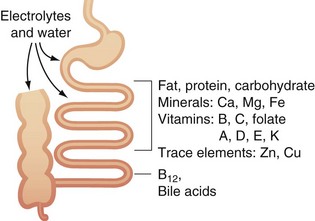
FIGURE 50-9 Absorption of water and electrolytes in the small bowel and colon.
(Adapted from Westergaard H: Short bowel syndrome. In Feldman M, Scharschmidt BF, Sleisenger MH [eds]: Sleisenger & Fordtran’s gastrointestinal and liver disease: Pathology, diagnosis, management, Philadelphia, 2002, WB Saunders, p 1549.)
Electrolytes can be absorbed in the small bowel by active transport or by coupling to organic solute.2 Na+ is absorbed by active transport through the basolateral membranes. Cl− is absorbed in the upper part of the small intestine by a process of passive diffusion. Large quantities of HCO3− must be reabsorbed, which is accomplished in an indirect fashion. As the Na+ is absorbed, H+ is secreted into the lumen of the intestine. It then combines with HCO3− to form carbonic acid, which then dissociates to form water and carbon dioxide. The water remains in the chyme, but the carbon dioxide is readily absorbed in the blood and is subsequently expired. Calcium is absorbed, particularly in the proximal intestine (duodenum and jejunum), by a process of active transport; absorption appears to be facilitated by an acid environment and is enhanced by vitamin D and parathyroid hormone. Iron is absorbed as a heme or nonheme component in the duodenum by an active process. Iron is then deposited within the cell as ferritin or transferred to the plasma bound to transferrin. The total absorption of iron is dependent on body stores of iron and the rate of erythropoiesis; any increase in erythropoiesis increases iron absorption. Potassium, magnesium, phosphate, and other ions also can be actively absorbed throughout the mucosa.
Vitamins are fat-soluble (e.g., vitamins A, D, E, and K) or water-soluble (e.g., ascorbic acid [vitamin C], biotin, nicotinic acid, folic acid, riboflavin, thiamine, pyridoxine [vitamin B6], and cobalamin [vitamin B12]).2 The fat-soluble vitamins are carried in mixed micelles and transported in chylomicrons of lymph to the thoracic duct and into the venous system. The absorption of water-soluble vitamins appears to be more complex than originally thought. Vitamin C is absorbed by an active transport process that incorporates a sodium-coupled mechanism as well as a specific carrier system. Vitamin B6 appears to be rapidly absorbed by simple diffusion into the proximal intestine. Thiamine (vitamin B1) is rapidly absorbed in the jejunum by an active process similar to the sodium-coupled transport system for vitamin C. Riboflavin (vitamin B2) is absorbed in the upper intestine by facilitated transport. The absorption of vitamin B12 occurs primarily in the terminal ileum. Vitamin B12 is derived from cobalamin, which is freed in the duodenum by pancreatic proteases. The cobalamin binds to intrinsic factor, which is secreted by the stomach, and is protected from proteolytic digestion. Specific receptors in the terminal ileum take up the cobalamin–intrinsic factor complex, probably by translocation. In the ileal enterocyte, free vitamin B12 is bound to an ileal pool of transcobalamin II, which transports it into the portal circulation.
Motility
Food particles are propelled through the small bowel by a complex series of muscular contractions.2 Peristalsis consists of intestinal contractions passing aborally at a rate of 1 to 2 cm/second. The major function of peristalsis is the movement of intestinal chyme through the intestine. Motility patterns in the small bowel vary greatly between the fed and fasted states. Pacesetter potentials, which are thought to originate in the duodenum, initiate a series of contractions in the fed state that propel food through the small bowel.
Endocrine Function
Gastrointestinal Hormones
The gastrointestinal hormones are distributed along the length of the small bowel in a spatially specific pattern. In fact, the small bowel is the largest endocrine organ in the body. Although often classified as hormones, these agents do not always function in a truly endocrine fashion (i.e., discharged into the bloodstream, where an action is produced at some distant site; Fig. 50-10). Sometimes, these peptides are discharged and act locally in a paracrine or autocrine manner. In addition, these peptides may serve as neurotransmitters (e.g., vasoactive intestinal peptide). The gastrointestinal hormones play a major role in pancreaticobiliary and intestinal secretion and motility. In addition, certain gastrointestinal hormones exert a trophic effect on normal and neoplastic intestinal mucosa and pancreas. The location, major stimulants of release, and primary effects of the more important gastrointestinal hormones are summarized in Table 50-3. In addition, the diagnostic and therapeutic uses of gastrointestinal hormones are listed in Table 50-4. Dockray3 and Gariepy and Dickinson4 have presented a more in-depth discussion of the structure, molecular biology, physiologic functions, and uses of these hormones.
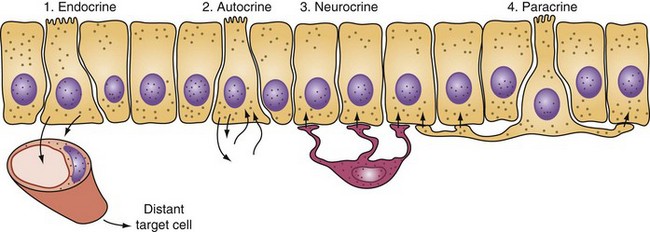
FIGURE 50-10 Actions of intestinal hormones may be via endocrine, autocrine, neurocrine, or paracrine effects.
(Adapted from Miller LJ: Gastrointestinal hormones and receptors. In Yamada T, Alpers DH, Laine L, et al [eds]: Textbook of gastroenterology, ed 3, vol 1, Philadelphia, 1999, Lippincott Williams & Wilkins, p 37.)
Table 50-4 Diagnostic and Therapeutic Uses of Gastrointestinal Hormones
HORMONE | DIAGNOSTIC AND THERAPEUTIC USES |
---|---|
Gastrin | Pentagastrin (gastrin analogue) used to measure maximal gastric acid secretion |
Cholecystokinin | Biliary imaging of gallbladder contraction |
Secretin | Provocative test for gastrinoma |
Measurement of maximal pancreatic secretion | |
Glucagon | Suppresses bowel motility for endocrine spasm |
Relieves sphincter of Oddi spasm | |
Provocative test for insulin, catecholamine, and growth hormone release | |
Somatostatin analogues | Treat carcinoid diarrhea and flushing |
Decrease secretion from pancreatic and intestinal fistulas | |
Ameliorate symptoms associated with hormone-overproducing endocrine tumors | |
Treat esophageal variceal bleeding |
Immune Function
Peyer patches are unencapsulated lymphoid nodules that constitute an afferent limb of the gut-associated lymphoid tissue, which recognizes antigens through the specialized sampling mechanism of the microfold (M) cells contained within the follicle-associated epithelium (Fig. 50-11). Antigens that gain access to the Peyer patches activate and prime B and T cells in that site. The M cells cover the lymphoid follicles in the gastrointestinal tract and provide a site for the selective sampling of intraluminal antigens. Activated lymphocytes from intestinal lymphoid follicles then leave the intestinal tract and migrate into afferent lymphatics that drain into mesenteric lymph nodes. Furthermore, these cells migrate into the lamina propria. The B lymphocytes become surface immunoglobulin A (IgA)–bearing lymphoblasts, which serve a critically important role in mucosal immunity.
Obstruction
Causes
The causes of a small bowel obstruction can be divided into three categories (Box 50-1):
Box 50-1 Adapted from Tito WA, Sarr MG: Intestinal obstruction. In Zuidema GD (ed): Surgery of the alimentary tract, Philadelphia, 1996, WB Saunders, pp 375–416.
Causes of Mechanical Small Intestinal Obstruction in Adults
The causes of small bowel obstruction have changed dramatically since the 1900s.5 At the turn of the 20th century, hernias accounted for more than 50% of mechanical intestinal obstructions. With the routine elective repair of hernias, this cause has dropped to the third most common cause of small bowel obstruction in industrialized countries. Adhesions secondary to previous surgery are now the most common cause of small bowel obstruction (Fig. 50-12).
Miscellaneous causes of bowel obstruction account for 2% to 3% of all cases but should be considered in the differential diagnosis. These include intussusception of the bowel, which in the adult is usually secondary to a pathologic lead point, such as a polyp or tumor (Fig. 50-13), gallstones, which can enter the intestinal lumen by a cholecystenteric fistula and cause obstruction, enteroliths originating from jejunal diverticula, foreign bodies, and phytobezoars.
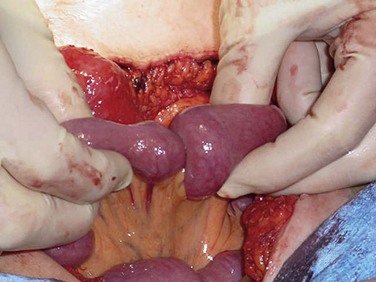
FIGURE 50-13 Jejunojenunal intussusception in an adult patient.
(Courtesy Dr. Steven Williams, Nampa, ID.)
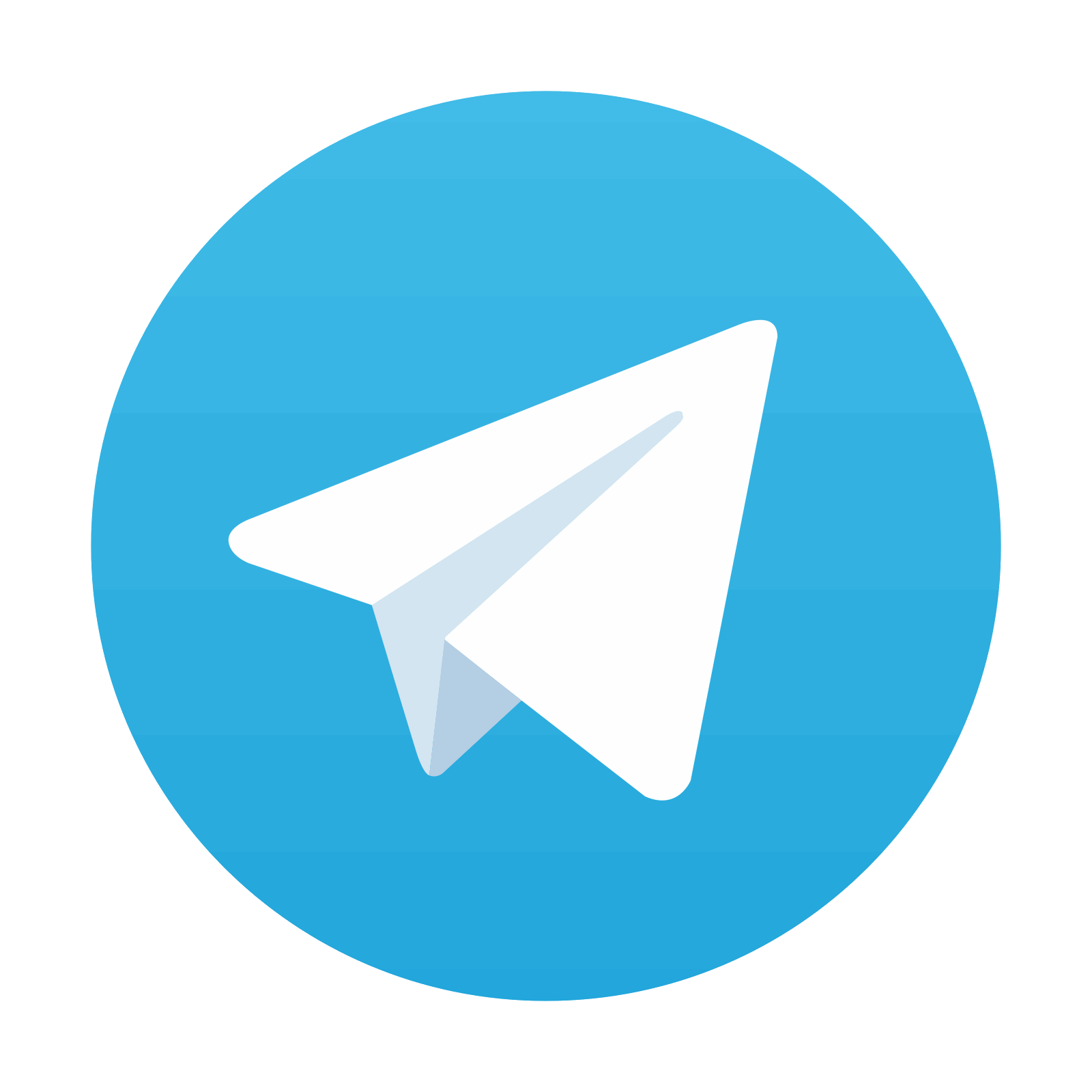
Stay updated, free articles. Join our Telegram channel

Full access? Get Clinical Tree
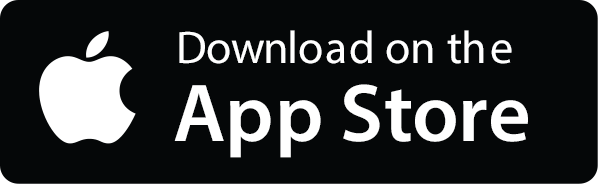
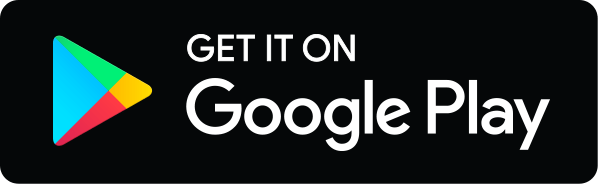