The relation between remodeling and left ventricular (LV) torsion has not yet been fully investigated. The aim of this study was to determine whether LV torsion assessed by speckle tracking imaging can predict progressive LV dilation after acute myocardial infarction (AMI). From January 2006 through June 2008, 91 patients with AMI who were successfully treated with primary coronary intervention underwent conventional and speckle tracking echocardiographies at initial presentation and 3 days and 6 months after first AMI. Patients were divided into 2 groups based on presence of LV remodeling (increase of LV end-diastolic volume >20%) at 6-month follow-up. LV remodeling developed in 23 patients. At initial presentation, LV end-diastolic volume was not significantly different between the no-remodeling and remodeling groups (91.27 ± 35.68 vs 85.74 ± 28.89 ml, p = 0.51), but LV torsion (2.23 ± 0.67 vs 1.70 ± 0.58°/cm, p <0.05) was significantly decreased in the remodeling group. At 6-month follow-up speckle tracking echocardiography, apical rotation and global torsion in patients with remodeling were 6.7 ± 2.6 (p <0.05) and 1.7 ± 0.7°/cm (p = 0.76 from baseline), respectively, and in patients without remodeling, 8.8 ± 3.4 (p <0.01) and 2.5 ± 0.7°/cm (p <0.01 from baseline), respectively. According to receiver operating characteristic analysis, LV torsion of 1.9°/cm (area under curve 0.79, sensitivity 75%, specificity 78%) at initial presentation was selected as a significant predictor of remodeling. In conclusion, decreased LV torsion assessed by speckle tracking echocardiography may predict late LV remodeling after reperfusion therapy after AMI.
After acute myocardial infarction (AMI), dysfunctional myocardium may exhibit changes in ventricular mass and chamber size and shape, known as “left ventricular (LV) remodeling”. Numerous studies have demonstrated that LV remodeling correlates with the incidence of heart failure and death, supporting a causative role for remodeling in heart failure progression. Several diagnostic techniques have been used to identify early prediction of LV remodeling. LV torsional deformation, rotation of the apex and base of the left ventricle in its longitudinal axis in opposite directions, results from subendocardial and subepicardial helixes. Measurement of such a fundamental determinant of cardiac motion might be a clinical index of contractility and could potentially become a sensitive marker of LV function. LV torsion and untwisting are known to play an important role in systolic and diastolic LV performance. The recently developed 2-dimensional speckle tracking imaging has been introduced as a noninvasive method for evaluating LV torsion. In a previous study Takeuchi et al reported that systolic torsion was decreased and diastolic untwisting prolonged and delayed in patients with anterior wall MI and abnormal LV systolic function using 2-dimensional speckle tracking imaging. However, the relation between LV remodeling and torsion has not yet been fully investigated. In addition, there has been no study that included serial evaluations of LV torsion before and after primary percutaneous coronary intervention (PCI) in patients with AMI. We hypothesized that assessment of LV torsion could provide additional and important information in the setting of AMI, allowing identification of patients at high risk for progressive LV dilation in the 6 months after AMI.
Methods
From January 2006 to June 2008, we studied 97 consecutive patients who were admitted to the coronary care unit with a first AMI (97 men, 61.1 ± 12.6 years old). Study inclusion criteria were (1) confirmed first AMI, (2) successful primary PCI (defined as Thrombolysis In Myocardial Infarction Trial grade 3 flow and residual stenosis <30%), and (3) AMI which occured within 6 hours of onset of symptoms or 6 to 24 hours if there was evidence of continuing ischemia. Exclusion criteria included clinical signs of heart failure or cardiogenic shock in the first week after AMI, atrial fibrillation, significant valvular heart disease, and hemodynamically unstable conditions, such as patients requiring mechanical ventilation or receiving inotropic agents or hemodynamic support with an intra-aortic balloon pump.
Diagnosis of AMI was based on documented transient increase of biochemical markers of myocardial necrosis (creatine kinase-MB and/or troponin I) and presence of typical symptoms and/or electrocardiographic signs of AMI.
Echocardiographic images were obtained with a 3.5-MHz transducer in the left lateral decubitus position using a commercially available system (Vivid 7, GE Vingmed, Horten, Norway). LV volumes and ejection fraction (EF) were obtained by modified Simpson biplane technique. The LV wall was divided into 16 segments and the wall motion of each segment was visually evaluated and scored (1 = normal; 2 = hypokinesis; 3 = akinesia; 4 = dyskinesis; 5 = aneurysmal). The average of scores of evaluated segments served as the wall motion score index (WMSI). Mitral early and late diastolic flow velocities were measured and a mitral early/late diastolic flow velocity ratio was calculated. Deceleration time of mitral E wave was measured and Doppler tissue imaging of the mitral annulus was obtained from the apical 4-chamber view. Systolic early and late diastolic mitral annulus velocities were measured. From these a systolic early/late diastolic mitral annulus velocity ratio was calculated.
Torsion measurements were performed off-line using dedicated software (EchoPac PC 6.1.3, GE Medical Systems, Horten, Norway). Three consecutive cardiac cycles were measured and averaged. Mean frame rate of the obtained images was 70 frames/s (range 50 to 90). Basal and apical short-axis views were used for measurement of rotation and torsion. The software then selected stable speckles within the myocardium and tracked these speckles frame by frame throughout the cardiac cycle. Counterclockwise rotation as viewed from the LV apex was denoted by a positive value, with clockwise rotation by a negative value. Co-ordinates for the rotation for each frame were exported to a spreadsheet program (Excel 2003, Microsoft Corp., Redmond, Washington). Instantaneous twist was then calculated by subtracting basal rotation from apical rotation at corresponding time points in the cardiac cycle. Twist was the difference between basal rotation and apical rotation and torsion was defined as twist divided by LV length (between LV apex and LV base). LV torsion was calculated as LV torsion (degrees per centimeter) = (apical LV rotation minus basal LV rotation)/LV diastolic longitudinal length (that was measured between locations of the base and apex of the left ventricle at the end of diastolic phase). From these traces peak global and regional LV systolic torsion values were recorded and used for subsequent analysis. For serial assessments of torsion, patients underwent conventional and speckle tracking echocardiographies at initial presentation and 3 days and 6 months after AMI. Patients were divided into 2 groups according to Doppler echocardiographic LV end-diastolic volume assessed 6 months after PCI; LV remodeling was defined as an increased LV end-diastolic volume >20% compared to initial presentation, and no remodeling was defined as an LV end-diastolic volume change <20%. At 6 months after PCI, all patients underwent follow-up coronary angiography to evaluate in-stent restenosis or de novo region.
Interobserver variabilities for LVEF, WMSI, and LV global torsion were measured by analysis of 10 random patients by 2 independent blinded observers. Intraobserver variabilities for LVEF, WMSI, and LV global torsion were measured by analysis of 10 patients by the same observer at 2 different time points. Intra- and interobserver variabilities were calculated and expressed as percent average value.
Analysis of data was performed using SPSS 17.0 (SPSS, Inc., Chicago, Illinois). A p value <0.05 was considered statistically significant. All numeric data are presented as mean ± SD. Variables were compared between the 2 groups using unpaired Student’s t test. To analyze change over time in LV volume, WMSI, EF, and global torsion, paired t tests were used. A Cox proportional hazards model was used for multivariate analysis (forward stepwise) to investigate which echocardiographic parameters identified using univariate analysis were significantly associated with LV remodeling. Diagnostic use of functional variables with significant differences between patients with and without remodeling was compared through receiver operating characteristic curves. Best cut-off value was defined as the point with the highest sum of sensitivity and specificity. Results were expressed as area under the curve or 95% confidence interval for this area.
Results
Of the 97 patients initially selected for the study, 1 patient (1.0%) was excluded because of cardiogenic shock with inotropic agents and ventilator care, 3 patients (3.1%) were excluded with moderate to severe mitral valve regurgitation during follow-up, and 2 patients (2.0%) with atrial fibrillation were excluded. Ninety-one patients with AMI were followed up at 6 months. During the mean follow-up duration of 26 ± 16 months, LV remodeling occurred in 25% (23 of 91 patients), including congestive heart failure in 2 patients. All patients had follow-up coronary angiography and no significant in-stent restenosis was detected in any patient. Table 1 lists baseline clinical characteristics of patients with and without LV remodeling. There was no significant difference between the 2 groups with respect to age, gender, blood pressure, Killip class, and frequency of coronary risk factors including previous ischemic heart disease. Administration of aspirin, clopidogrel, β blockers, angiotensin-converting enzyme inhibitors, angiotensin receptor blockers, and statins was not significantly different between the 2 groups during the 6-month follow-up period. Initial troponin I was significantly higher in the remodeling group (p <0.05), whereas peak creatine kinase-MB value was not significantly different between the no-remodeling and remodeling groups (p = 0.50). The proportion of left anterior descending coronary artery lesions in the no-remodeling group was smaller than in the remodeling group (p <0.05). With regard to echocardiographic parameters, LV end-diastolic volume, LV end-systolic volume, and deceleration time did not differ significantly between the 2 groups. However, WMSI was significantly higher and LVEF was lower in the remodeling group (p <0.05). At initial presentation LV apical rotation (p <0.05) and torsion (p <0.05) were significantly decreased in the remodeling group, but LV basal rotation (p = 0.16) was not significantly different between the 2 groups. Figure 1 and Table 2 present serial changes in regional and global LV functions and volumes. LV end-diastolic volume significantly increased in the remodeling group from baseline to 6-month follow-up (p <0.01), whereas LV end-diastolic volume in the no-remodeling group decreased from baseline to 6-month follow-up (p <0.01). A significant recovery in LVEF from baseline to 6-month follow-up was observed in the no-remodeling group (p <0.01), whereas no significant improvement was observed in the remodeling group (p = 0.56).
No Remodeling (n = 68) | Remodeling (n = 23) | p Value | |
---|---|---|---|
Age (years) | 63.7 ± 11.9 | 63.6 ± 11.3 | 0.74 |
Men (%) | 48 (70%) | 14 (61%) | 0.44 |
Body mass index (kg/m 2 ) | 24.8 ± 3.0 | 24.5 ± 3.1 | 0.62 |
Systolic blood pressure (mm Hg) | 131.5 ± 28.2 | 128.0 ± 20.3 | 0.47 |
Diastolic blood pressure (mm Hg) | 78.5 ± 14.3 | 78.9 ± 9.3 | 0.81 |
Heart rate (beat/min) | 77.3 ± 16.2 | 85.2 ± 20.3 | <0.05 |
Killip classes I and II/II and IV | 59 (87%)/9 (13%) | 18 (74%)/5 (26%) | 0.32 |
Symptom-to-balloon time (minutes) | 233.6 ± 100.5 | 237.3 ± 68.4 | 0.61 |
Door-to-balloon time (minutes) | 68.7 ± 23.0 | 66.7 ± 9.9 | 0.67 |
ST-segment elevation myocardial infarction/non–ST-segment elevation myocardial infarction | 25 (37%)/43 (63%) | 13 (56%)/10 (44%) | <0.01 |
Left anterior descending coronary artery lesion | 29 (43%) | 17 (74%) | <0.05 |
Risk factors | |||
Previous ischemic heart disease | 5 (7%) | 3 (13%) | 0.41 |
Hypertension | 38 (%) | 15 (%) | 0.47 |
Diabetes mellitus | 18 (26%) | 8 (35%) | 0.44 |
Dyslipidemia | 13 (%) | 6 (%) | 0.55 |
Current smoker/ex-smoker | 22 (32%)/11 (16%) | 7 (30%)/4 (17%) | 0.87 |
Medication | |||
Aspirin | 68 | 23 | |
Clopidogrel | 68 | 23 | |
β blocker | 44 (65%) | 12 (52%) | 0.33 |
Angiotension-converting enzyme inhibitor | 26 (38%) | 12 (52%) | 0.33 |
Angiotensin receptor blocker | 34 (50%) | 8 (35%) | 0.23 |
Statin | 57 (84%) | 17 (74%) | 0.35 |
Cardiac markers | |||
Initial troponin I (ng/ml) | 3.2 ± 8.9 | 11.4 ± 28.6 | <0.05 |
Initial creatine kinase-MB (ng/ml) | 22.8 ± 45.4 | 35.9 ± 69.4 | 0.30 |
Maximum creatine kinase-MB (ng/ml) | 112.2 ± 108.5 | 130.4 ± 123.7 | 0.50 |
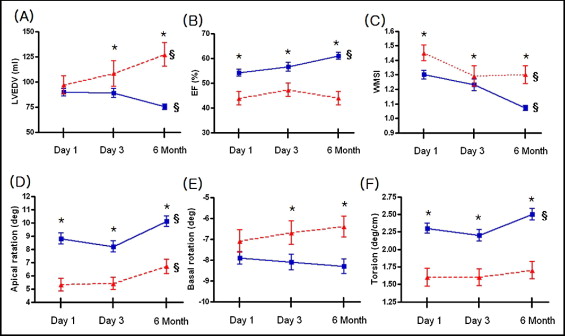
Day 1 | Day 3 | 6 Months | ||||
---|---|---|---|---|---|---|
No Remodeling | Remodeling | No Remodeling | Remodeling | No Remodeling | Remodeling | |
Left ventricular end-diastolic volume (ml) | 89.6 ± 31.8 | 96.8 ± 42.7 | 88.9 ± 26.3 | 108.2 ± 52.9 ⁎ | 75.3 ± 22.6 | 126.9 ± 56.3 ⁎ |
Left ventricular end-systolic volume (ml) | 43.5 ± 23.8 | 56.2 ± 35.5 | 38.9 ± 23.8 | 56.4 ± 44.9 ⁎ | 39.5 ± 12.1 | 70.7 ± 45.8 ⁎ |
Ejection fraction (%) | 54.2 ± 11.6 | 43.8 ± 13.1 ⁎ | 56.5 ± 10.3 | 47.2 ± 11.4 ⁎ | 60.9 ± 11.3 | 43.9 ± 12.8 ⁎ |
Wall motion score index | 1.30 ± 0.25 | 1.45 ± 0.26 ⁎ | 1.23 ± 0.23 | 1.29 ± 0.29 ⁎ | 1.07 ± 0.15 | 1.30 ± 0.30 ⁎ |
Deceleration time (ms) | 197.2 ± 51.7 | 206.4 ± 99.5 | 227.1 ± 76.7 | 210.6 ± 75.1 | 224.4 ± 61.2 | 175.2 ± 70.3 ⁎ |
Mitral early/late diastolic flow velocity ratio | 1.0 ± 0.3 | 0.9 ± 0.5 | 1.0 ± 0.4 | 1.1 ± 0.9 | 0.9 ± 0.4 | 1.3 ± 1.1 ⁎ |
Systolic early/late diastolic mitral annulus velocity ratio | 13.3 ± 4.8 | 15.6 ± 7.7 | 14.3 ± 6.4 | 16.5 ± 8.6 | 12.9 ± 6.2 | 17.0 ± 9.4 ⁎ |
Apical rotation (°) | 8.8 ± 3.4 | 5.3 ± 2.3 ⁎ | 8.2 ± 2.5 | 5.4 ± 1.9 ⁎ | 10.1 ± 3.3 | 6.7 ± 2.6 ⁎ |
Basal rotation (°) | −7.9 ± 2.5 | −7.1 ± 2.6 | −8.1 ± 2.2 | −6.7 ± 2.3 ⁎ | −8.3 ± 2.9 | −6.4 ± 2.4 ⁎ |
Twist (°) | 16.8 ± 4.1 | 12.4 ± 4.3 ⁎ | 16.2 ± 3.8 | 12.2 ± 3.7 ⁎ | 18.7 ± 4.6 | 13.1 ± 4.4 ⁎ |
Torsion (°/cm) | 2.3 ± 0.6 | 1.6 ± 0.6 ⁎ | 2.2 ± 0.5 | 1.6 ± 0.5 ⁎ | 2.5 ± 0.7 | 1.7 ± 0.6 ⁎ |
At 6-month follow-up, apical rotation had raised significantly (p <0.01 in the 2 groups), but basal rotation was not significantly increased (p = 0.37 in no-remodeling group, p = 0.10 in remodeling group) in either group from baseline. There was an amelioration in torsion in patients without remodeling from baseline (p <0.01), whereas torsion in the remodeling group was not improved (p = 0.76).
By univariate analysis using a Cox proportional hazards model, WMSI, EF, and global torsion at initial presentation were significantly associated with LV remodeling. We also analyzed postinterventional echocardiographic parameters to evaluate the effect of intervention. WMSI, EF, and global torsion at 3 days after PCI were also significantly associated with LV remodeling by univariate analysis. The hazard ratio of each variable is presented in Table 3 . Using multivariate Cox analysis, initial global torsion, global torsion 3 days after PCI, and EF 3 days after PCI were independent predictors of LV remodeling in patients with AMI ( Table 3 ).
Univariate Analysis | Multivariate Analysis | |||
---|---|---|---|---|
HR (95% CI) | p Value | HR (95% CI) | p Value | |
Left anterior descending coronary artery lesion | 3.81 (1.33–10.86) | <0.05 | ||
ST-segment elevation myocardial infarction | 1.76 (0.68–4.57) | 0.24 | ||
Initial presentation | ||||
Deceleration time | 1.00 (0.99–1.01) | 0.56 | ||
Mitral early/late diastolic flow velocity ratio | 0.76 (0.20–2.85) | 0.68 | ||
Systolic early/late diastolic mitral annulus velocity ratio | 1.07 (0.98–1.15) | 0.11 | ||
Left ventricular end-diastolic volume | 1.01 (0.98–1.02) | 0.39 | ||
Left ventricular end-systolic volume | 1.01 (0.99–1.03) | 0.07 | ||
Wall motion score index | 7.98 (1.31–48.75) | <0.05 | 0.40 (0.03–6.18) | 0.51 |
Ejection fraction | 0.93 (0.88–0.97) | <0.01 | 0.94 (0.88–1.01) | 0.09 |
Apical rotation | 0.64 (0.51–0.81) | <0.01 | ||
Basal rotation | 1.15 (0.95–1.39) | 0.16 | ||
Global torsion | 0.13 (0.04–0.38) | <0.01 | 0.13 (0.04–0.41) | <0.01 |
3 days after percutaneous coronary intervention | ||||
Deceleration time | 0.99 (0.98–1.01) | 0.37 | ||
Mitral early/late diastolic flow velocity ratio | 1.35 (0.61–2.98) | 0.46 | ||
Systolic early/late diastolic mitral annulus velocity ratio | 1.04 (0.98–1.11) | 0.21 | ||
Left ventricular end-diastolic volume | 1.01 (1.00–1.03) | <0.05 | 1.01 (0.97–1.04) | 0.66 |
Left ventricular end-systolic volume | 1.02 (1.00–1.04) | <0.05 | 0.98 (0.94–1.03) | 0.45 |
Wall motion score index | 4.07 (1.00–16.51) | <0.05 | 1.99 (0.12–31.68) | 0.63 |
Ejection fraction | 0.92 (0.88–0.97) | <0.01 | 0.93 (0.88–0.99) | <0.05 |
Apical rotation | 0.57 (0.43–0.75) | <0.01 | ||
Basal rotation | 1.31 (1.04–1.64) | <0.05 | ||
Global torsion | 0.12 (0.04–0.37) | <0.01 | 0.16 (0.04–0.58) | <0.01 |
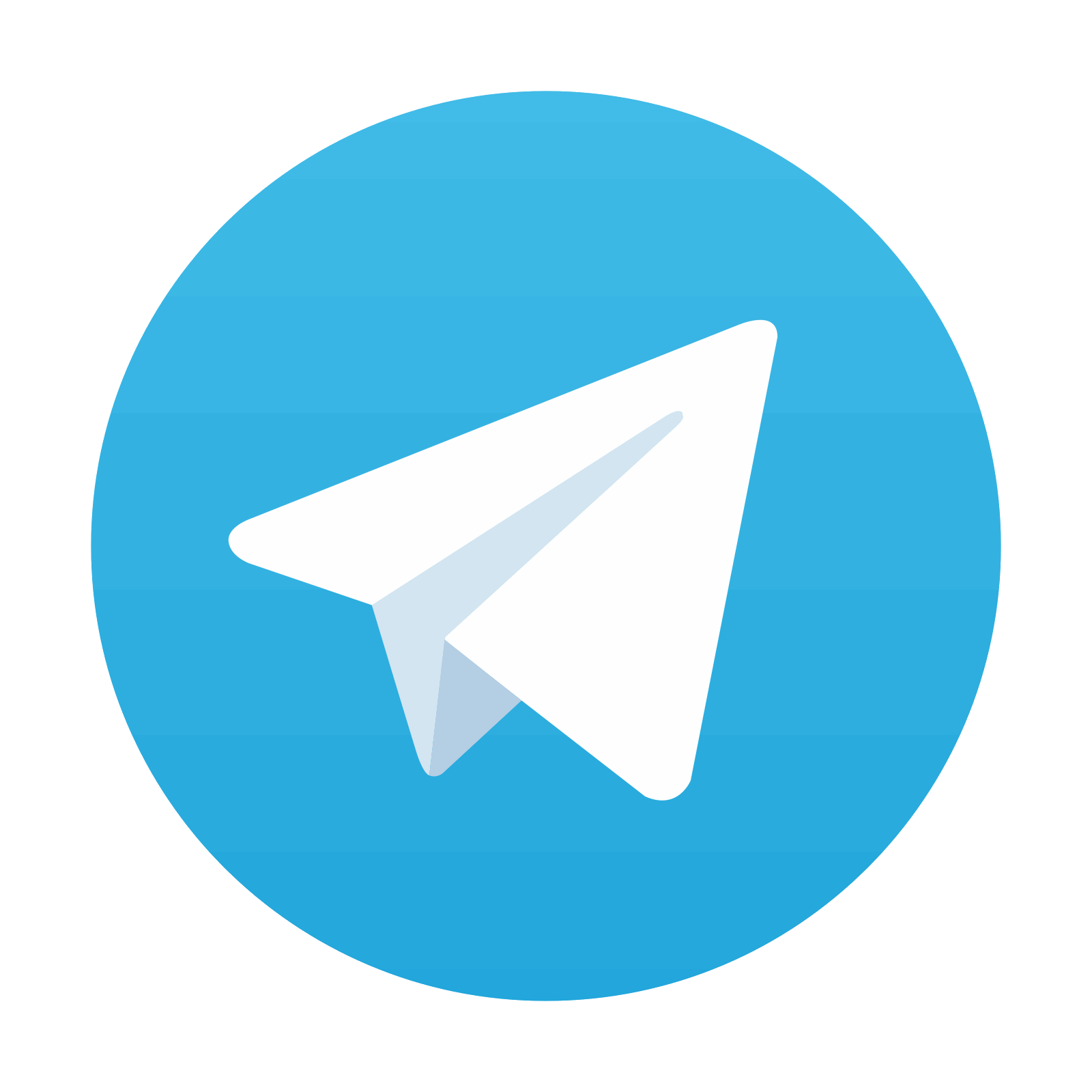
Stay updated, free articles. Join our Telegram channel

Full access? Get Clinical Tree
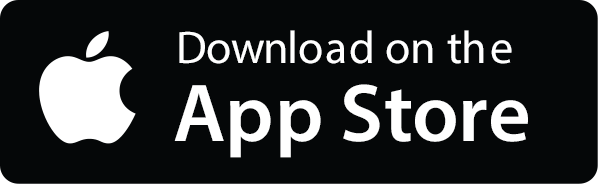
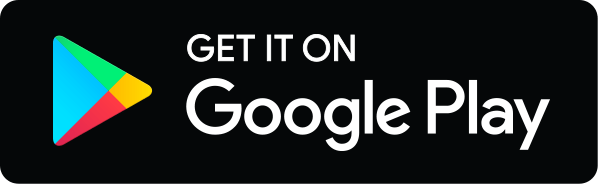
