Obstructive Sleep Apnea and Hypertension
Obstructive sleep apnea (OSA) and hypertension are common medical conditions which often coexist. Is there is a causal relationship between these two conditions? This question has been repeatedly raised in literature and has remained an area of interest for many years. The association between OSA and hypertension was initially reported by Tilkian et al in 1976 who demonstrated a substantial cyclical elevation in blood pressure with each apneic episode. Systemic hypertension was also noted in one-third of the patients with OSA.
In this chapter we will review the epidemiology of OSA and hypertension; mechanisms by which OSA leads to development and/or progression of hypertension; and the effect of treating OSA on hypertension.
Definition of Obstructive Sleep Apnea
OSA is defined by the occurrence of daytime sleepiness, loud snoring, witnessed breathing interruptions, or awakenings as a result of gasping or choking in the presence of at least five obstructive respiratory events (apneas, hypopneas, or respiratory effort–related arousals) per hour of sleep. The presence of 15 or more obstructive respiratory events per hour of sleep in the absence of sleep-related symptoms is also sufficient for the diagnosis of OSA because of a greater association of this severity of obstruction with important consequences such as increased cardiovascular disease risk.
Obstructive apneas or hypopneas occur when the upper airway dilator muscles fail to maintain the patency of the upper airway and airflow during sleep. Various factors that can increase the risk for developing OSA include altered facial structure, small upper airway lumen, poor upper airway muscle function, respiratory control instability, increased arousal response, small lung volumes, fluid retention, male sex, obesity, advancing age, genetic factors, menopause, and smoking.
Prevalence of Obstructive Sleep Apnea and Hypertension
Sleep apnea occurs in 3% to 7% of adult males and 2% to 5% of adult females in the general population. Peppard et al recently reported the prevalence of OSA of 26% among patients between 30 to 70 years of age. A population-based, age-stratified case control study done in Sweden assessed the prevalence of OSA in men. This study reported that the prevalence of OSA in primary hypertension was 35%. In the Wisconsin Sleep Cohort, the odds ratios for the presence of hypertension at 4-year follow-up was 2.89 with an apnea-hypopnea index (AHI) of 15 or more events per hour compared with those with an AHI of 0. This study demonstrated a dose-response relationship between the severity of OSA and presence of hypertension, independent of the confounding factors. The Sleep Heart Health Study showed similar findings with an increase in prevalence of hypertension with increasing AHI.
Rapid eye movement (REM) sleep is generally associated with a greater propensity for upper airway closure because of inhibition of muscle tone during this stage of sleep. As a result, the likelihood of OSA or worsening of OSA in REM sleep is increased. In addition, REM sleep is also associated with increased sympathetic activity which can contribute to a rise in blood pressure. The Wisconsin Sleep cohort reported a significant dose-relationships between REM AHI and prevalent hypertension. The higher relative odds of prevalent hypertension were most evident with REM AHI 15 or more. In individuals with a non-REM AHI 5 or less, a two-fold increase in REM AHI was associated with 24% higher odds ratio of hypertension. Longitudinal analysis also revealed a significant association between higher REM AHI and the development of hypertension ( p trend = 0.017).
A recent report with a 5-year follow-up from the Sleep Heart Health Study concluded that the association between hypertension and AHI was not significant after adjusting for body mass index (BMI). Similarly, the Vitoria Sleep Cohort also found no association between OSA and incidence of hypertension after adjustment for confounders. It is unclear why there are variations in the results of these studies but these could be accounted by differences in the populations sampled and techniques used to diagnose sleep apnea or AHI cutoff points may account for the variation related to the impact of OSA on prevalent and incident hypertension.
There is a striking association between drug-resistant hypertension and OSA. Prevalence of OSA in patients with drug-resistant hypertension has been reported to be approximately 64% to 83%. Pedrosa et al identified OSA as the most common secondary cause of hypertension in patients with drug-resistant hypertension ( Fig. 16.1 ).

Treatment of OSA with continuous positive airway pressure (CPAP) has been shown to lower blood pressure (BP) in this patient cohort. Similar impact of CPAP on BP was observed in a recent study including patients with resistant and nonresistant hypertension and OSA. These findings support the role of OSA in the pathogenesis of hypertension in these patients ( Fig. 16.2 ).

Mechanism of Obstructive Sleep Apnea
Anatomically, the upper airway has a limited bony support. There are multiple anatomic and physiologic factors which promote the collapse of this portion of the airway. These influences are offset by the factors which dilate the airway to maintain airway patency.
The two primary forces promoting airway collapse are intraluminal negative pressure generated by the diaphragm during inspiration and the extraluminal tissue pressure produced by tissues surrounding the airway. These influences are overcome by the action of pharyngeal dilator muscles, specifically genioglossus muscle along with the impact of tracheal traction on the airway from lung inflation. In addition, low lung volumes during nonrapid eye movement (NREM) sleep lead to less tension on the airway wall and are associated with increased airflow resistance and collapsibility.
OSA results from complete or partial collapse of the upper airway lumen during sleep. During state of wakefulness, upper airway patency is maintained by the activity of pharyngeal dilator muscles. This muscle activity tends to be reduced during sleep along with the decrease in lung volumes, increasing the likelihood of airway closure during sleep. Factors that affect the functioning of pharyngeal dilator muscles include fatigue, and neuropathic and myopathic injury also contribute to airway obstruction. Most patients with obstructive apnea have an anatomically small upper airway, either because of increased soft tissue surrounding the airway or a small bony structure surrounding the airway.
Stability of the respiratory control system may fluctuate during sleep leading to variation in activity of the upper airway dilator muscles. Low central respiratory drive leads to low upper airway dilator muscle activity, high airway resistance, and propensity to airway collapse.
Any disease state contributing to fluid retention and mobilization of fluid overnight from the legs to the neck may lead to narrowing of the airway lumen and increase the propensity to airway closure. This can be an important factor for OSA in conditions such as heart failure and end-stage renal disease ( Fig. 16.3 ).

Obesity and male gender are risk factors for OSA. Obesity promotes airway collapsibility by increasing extraluminal tissue pressure as a result of fat deposition. Men have greater total neck soft tissue volume and longer length of airway, independent of height, that may account for higher airway collapsibility.
Aging is a risk factor for OSA because of multiple reasons. Older individuals have an increased collapsibility of the airway caused by a loss of collagen and an increased deposition of parapharyngeal fat, an impairment of pharyngeal dilator muscles response to negative intraluminal pressure, a loss of elastic recoil of the lung leading to reduction in lung volumes, and a less tethering effect on the upper airways.
The ability to arouse from sleep can also be involved in pathogenesis of OSA. In general, after arousal, people hyperventilate for a short duration which may lead to drop in CO 2 concentration. If this drop is below the apnea threshold, central apnea occurs. As the upper airway dilator muscles receive respiratory input, hypocapnia reduces the activity of the upper airway dilator muscles and leads to collapse of the airway.
Other risk factors for OSA include genetic causes and ethnicity which affects craniofacial anatomy and may predispose to obesity. Menopause has also been identified as a risk factor for OSA because of central redistribution of body fat. Smoking has been associated with OSA and is likely as a result of increased upper airway inflammation and nasal stuffiness ( Fig. 16.4 ).

Cardiovascular Effects of Obstructive Sleep Apnea
Airway closure leads to a decrement or cessation of ventilation and development of hypoxia and hypercapnia. These changes lead to an arousal from sleep and increase in respiratory drive until the upper airway opens and blood gas abnormalities are reversed. Each episode of airway occlusion initiates a sequence of hemodynamic, autonomic, and chemical changes ( Fig. 16.5 ).

Each episode of OSA leads to a generation of negative intrathoracic pressure as an effort is made to breathe against a closed glottis. This leads to an increase in left ventricular (LV) transmural pressure, which in turn increases the LV afterload.
It also increases venous return which increases right ventricular (RV) preload. RV afterload also increases simultaneously as a result of hypoxic pulmonary vasoconstriction. These changes lead to RV distention and leftward displacement of the interventricular septum which impairs LV filling. The combination of increased LV afterload and diminished LV preload during obstructive apneas lead to a reduction in stroke volume and cardiac output. This increase in cardiac afterload raises the myocardial oxygen demand whereas OSA related hypoxia has reduced the oxygen supply. These changes can potentially precipitate myocardial ischemia in those with preexisting coronary artery disease, impair cardiac contractility, and diastolic relaxation. These repetitive episodes over time can induce LV hypertrophy ( Fig. 16.6 ).

Each episode of apnea leads to hypoxia and hypercapnia which are potent stimuli to chemoreceptors and lead to sympathetic stimulation. Apnea also induces sympathetic stimulation via elimination of inhibitory effects of pulmonary stretch receptors. Reductions in stroke volume during obstructive apneas reduce sympathetic inhibitory input from carotid sinus, in turn augmenting sympathetic outflow. Arousal from sleep at the end of apnea causes further adrenergic surge which leads to a rise in blood pressure and heart rate. Once the sleep ensues, the same cycle repeats itself ( Fig. 16.7 ).

The episodes of hypoxia followed by reoxygenation lead to generation of free radicals and induces inflammation. Induction of inflammation leads to vascular endothelial injury and atherogenesis. Patients with OSA have a reduction in nitric oxide levels, which in turn impairs endothelially mediated vasodilation and can lead to hypertension. Treating OSA with CPAP has been shown to reduce oxidative stress and improve nitrate levels ( Fig. 16.8 ).

A randomized trial has also reported a reduction in carotid intima-media thickness, pulse-wave velocity, C-reactive protein, and catecholamine levels in patients treated with CPAP.
Mechanism of Obstructive Sleep Apnea in a Selected Population
Hypertension
OSA is associated with surges in sympathetic outflow which in turn raises the blood pressure and heart rate. Somers et al reported that patients with OSA have high sympathetic activity when awake with further increases in blood pressure and sympathetic activity during sleep, especially stage II and REM sleep. Peak sympathetic activity was noted at the end of each episode of apnea and was followed by a surge in blood pressures ( Fig. 16.9 ). Interestingly, peak mean blood pressures remained higher than wakefulness during all stages of sleep (116 ± 5 mm Hg during stage II and 127 ± 7 mm Hg during REM both ( p < 0.0001). This study also showed that treatment with CPAP attenuates sympathetic activity and blood pressure during sleep ( p < 0.03).

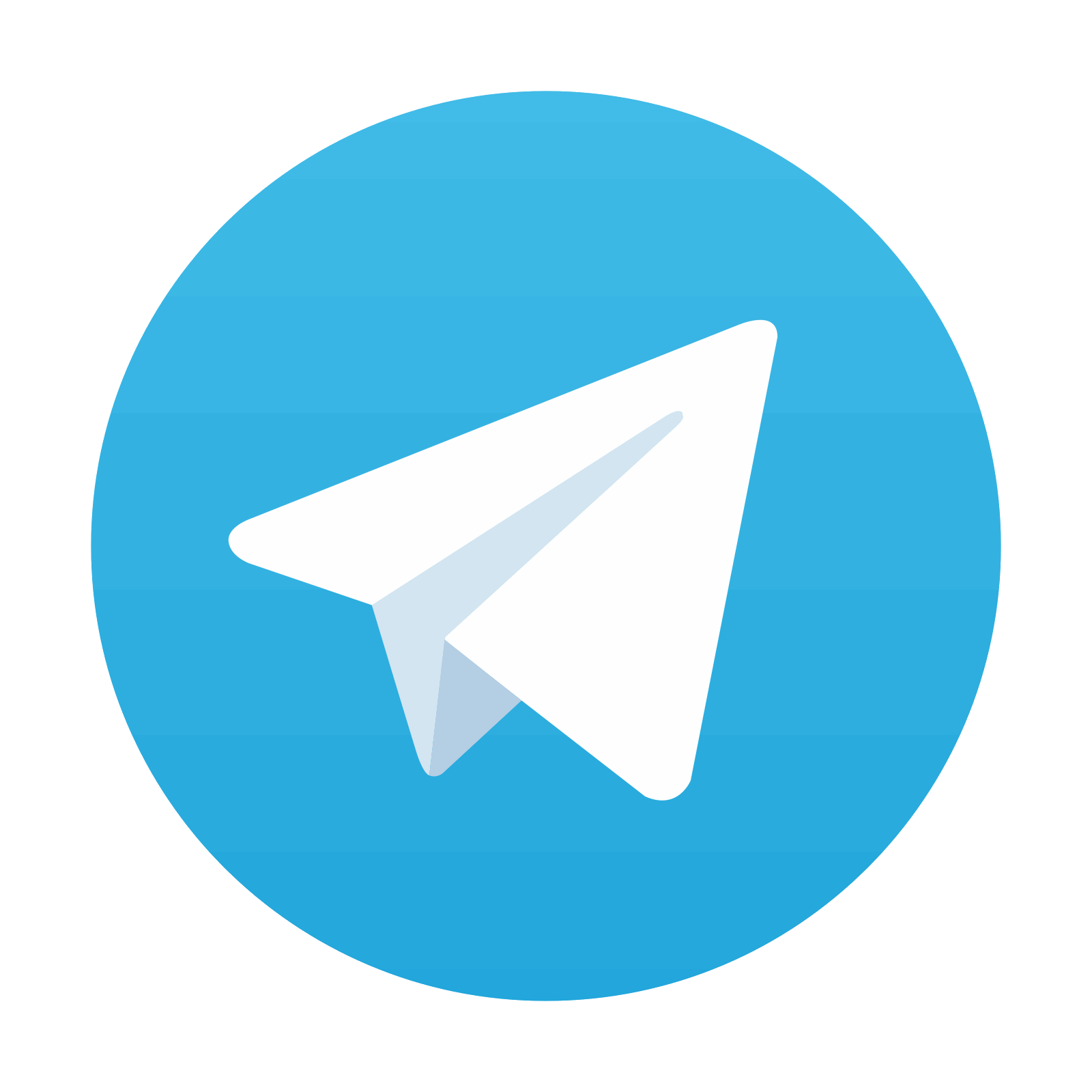
Stay updated, free articles. Join our Telegram channel

Full access? Get Clinical Tree
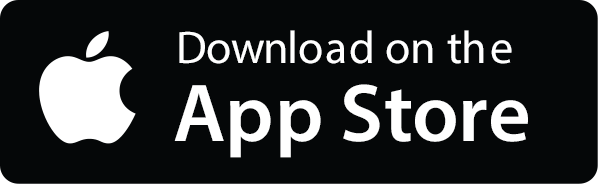
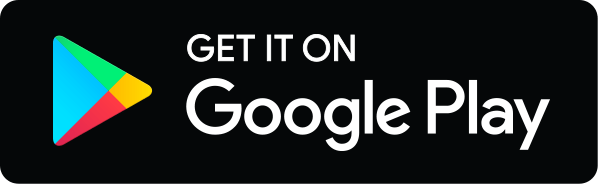
