Background
The distinction between right ventricular (RV) dysfunction due to an acute etiology (pulmonary embolism [PE]) or chronic afterload (pulmonary arterial hypertension [PAH]) has important therapeutic implications. The aim of this study was to test the hypothesis that RV remodeling would alter RV free wall strain (RVFWS) and differentiate chronic from acute RV afterload.
Methods
In this retrospective study, patients with PE ( n = 45) who underwent echocardiography within 48 hours of computed tomographic pulmonary angiography were matched 1:1 for age, gender, and pulmonary artery systolic pressure with patients with PAH ( n = 45) and a larger unmatched PAH control group ( n = 116). RV function was evaluated with end-diastolic area, fractional area change (FAC), and RVFWS by two-dimensional speckle-tracking. The ability of RVFWS to distinguish acute from chronic RV dysfunction was assessed using receiver operating characteristic curves, and its incremental value was sought with stepwise models.
Results
RV end-diastolic area, FAC, and RVFWS were significantly impaired in patients with PE ( P < .001), with no significant differences in other clinical variables. In matched patients, receiver operating characteristic curve analysis revealed that RVFWS had significantly better discriminative power than the McConnell sign ( P = .02), with a cutoff of −17.9%, sensitivity of 87.5%, specificity of 62.5%, and an area under the curve of 0.76. Sequential logistic regression demonstrated an incremental and independent benefit of using RVFWS to predict acute PE versus chronic PAH ( P = .01). Observer concordance was superior for RVFWS compared with FAC ( P < .01).
Conclusions
RVFWS is more predictive than RV end-diastolic area and less variable than FAC in distinguishing acute from chronic RV pressure overload. RVFWS adds incremental and independent information to standard measures of RV function in assessing the acuity of pulmonary hypertension.
The distinction between pulmonary embolism (PE) and chronic pulmonary arterial hypertension (PAH) may have important therapeutic implications. Although right ventricular (RV) function and size are important prognostic markers in both patients with PE and those with chronic PAH, RV function may differ between these entities. In chronic pressure overload, the myocardium is able to adapt and remodel to compensate and maintain cardiac output, as evidenced in previous experimental models using pulmonary artery banding. In contrast, the RV myocardium has limited time to increase contractility in acute pressure overload, resulting in RV dilation and impaired function.
The distinction between these causes might be facilitated by a technique that is able to quantify RV dysfunction to a degree that has not been feasible with standard imaging. This information is not readily gathered from computed tomographic pulmonary angiography. Pulmonary artery systolic pressure (PASP) will increase only if >30% to 50% of the pulmonary artery bed is occluded, and generally, echocardiography has limited value in submassive PE (negative predictive value, 40%–50%). Historically, subjective markers (such as the McConnell sign) have been used to diagnose acute PE, but this is limited by sensitivity of 70% and specificity of 33%. Strain imaging using speckle-tracking of the RV free wall is an emerging technique to measure myocardial deformation, which has been validated using sonomicrometry and is now readily available, including in the acute setting. Previous work has shown the ability of RV free wall strain (RVFWS) to identify RV dysfunction in patients with PAH. However, the load dependency of functional measurements can complicate the evaluation of systolic dysfunction. We sought to determine whether RV global and regional strain could be used in a clinical context to differentiate acute versus chronic increases in RV afterload.
Methods
Study Design
In this cross-sectional study of RV function, patients with acute PE were compared with control patients with PHT. Patients with acute PE ( n = 45) were retrospectively identified from administrative records and were eligible for study if echocardiography was performed within 48 hours of computed tomographic pulmonary angiography. These patients were anticoagulated, and none underwent thrombolysis. Most were hemodynamically stable, although two had documented systolic blood pressure < 90 mm Hg. Patients with documented chronic thromboembolic pulmonary hypertension were excluded. Control patients were obtained from a chronic PAH registry cohort from the Royal Hobart Hospital and Launceston General Hospital between 2005 and 2013. These latter had PAH diagnosed by right-heart catheterization and were treated with an endothelin receptor antagonist, a phosphodiesterase-5 inhibitor, intravenous or inhaled prostacyclin analogs, or their combination, in accordance with current guidelines. The primary control group comprised patients with PAH matched for age, gender, and PASP (as derived from transthoracic echocardiography) ( n = 45). Because matching is rarely perfect, an unmatched group from the same patient cohort ( n = 116) was included in a sensitivity analysis.
Standard Echocardiography
Studies were performed on commercially available GE (Vivid E9, Vivid 7, and Vivid I; GE Medical Systems, Milwaukee, WI) and Philips (iE33 and EPIQ; Philips Medical Systems, Andover, MA) equipment. The acquisition followed a standard protocol, including RV-focused views and measurement of pulsed-wave Doppler of the RV outflow tract for estimation of pulmonary vascular resistance (PVR).
All measurements were performed by a single reader. Standard RV and left ventricular (LV) measurements were performed per current American Society of Echocardiography guidelines. LV ejection fraction was measured using Simpson’s biplane method from the apical four- and two-chamber views. RV end-diastolic area (RVEDA) (square centimeters) and RV end-systolic area (square centimeters) were calculated from the apical RV-focused view. Fractional area change (FAC) was calculated as the percentage change between the RVEDA and RV end-systolic area. Peak velocities of the early (E) and late (A) diastolic filling were derived from the transmitral inflow pattern. Tissue Doppler imaging was used to determine the peak diastolic early velocity (e′) of the lateral and septal mitral annulus from the apical four-chamber view. PASP was calculated from the tricuspid regurgitation maximal signal, using the modified Bernoulli equation. Right atrial pressure was assessed via inferior vena cava collapsibility. To calculate PVR, tricuspid regurgitation maximum velocity was divided by the RV outflow time-velocity integral. The severity of tricuspid jet regurgitation was assessed using standard guidelines, on the basis of jet density, hepatic vein flow reversal, and tricuspid valve morphology.
Speckle Tracking Strain Analysis
RVFWS was assessed from an apical RV-focused view, using speckle-tracking (Image Arena; Tomtec, Unterschleissheim, Germany). The RV end-diastolic endocardial border was manually traced along the RV septal and RV free wall. These borders were then tracked automatically frame by frame throughout the cardiac cycle. Manual adjustments were performed when necessary, and regions were excluded if excessive noise or inadequate tracking was present. Peak systolic strain was obtained from the average of the three separate basal, mid and septal RVFWS traces, RV septal wall measurements were not used for analysis.
Statistical Analysis
Data were assessed for normality using the Shapiro-Wilk W test and transformed if necessary. For PVR values, the “linear trend at point” method was used for missing data. Patients were placed into equal tertiles for PVR because of an inability to normalize. Comparison of patients and control subjects was performed by matching 1:1 for age, gender, and PASP, as well as the inclusion of all control subjects into statistical models. Acuity of afterload increase was assessed as a binary variable (PAH vs PE). Associations of RV systolic function were determined using univariate and multivariate logistic regression. Anticipated associations were placed into the model (age, gender, and PASP). Incremental benefit was assessed by comparing the global χ 2 value for each model. Receiver operating characteristic curves were created to determine the diagnostic ability of RV functional measurements to distinguish those with and without acute increases in RV afterload, with determination of the area under the curve (AUC). Cutoff values were obtained using the Youden index. Interoperator variability was assessed in both the chronic pulmonary hypertension ( n = 30) and PE ( n = 25) studies. Variability studies were randomly selected, with the second reader not aware of reader 1’s results or which cardiac cycle and image window was measured.
Bland-Altman analysis was used to assess the mean difference and limits of agreement, and the intraclass correlation coefficient (ICC) was calculated.
Results
Patient Characteristics
Patient characteristics are presented in Table 1 . Patients in the PAH group were divided into 81 (50%) with idiopathic PAH, 68 (42%) with scleroderma, seven (3%) with congenital heart disease, four (2.5%) with chronic obstructive pulmonary disease and one (0.6%) with portopulmonary hypertension. The main clinical differences between PE and the PHT control subjects were in body mass index, heart rate, systolic blood pressure, and renal function. Atrial fibrillation was present in four members of the PE cohort (9%) and five members of the PAH group (3%). The severity of tricuspid regurgitation in patients with PAH was mild in 119 (74%), moderate in 27 (17%), and severe in 15 (9%).
Variable | Matched | P | |
---|---|---|---|
PE ( n = 45) | PAH ( n = 45) | ||
Age (y) | 64 ± 15 | 62 ± 13 | .57 |
Women | 69% | 69% | |
BMI (kg/m 2 ) | 34.5 ± 8.3 | 28.5 ± 9.1 | .01 |
SBP (mm Hg) | 115 ± 32 | 126 ± 18 | .09 |
DBP (mm Hg) | 69 ± 19 | 69 ± 8 | .90 |
HR (beats/min) | 92 ± 16 | 71 ± 12 | <.001 |
eGFR (mL/min/1.73 m 2 ) | 58 ± 17 | 66 ± 15 | .07 |
BUN (mmol/L) | 7.7 ± 3.6 | 6.7 ± 2.9 | .24 |
Creatinine (μmol/L) | 97 ± 36 | 83 ± 27 | .06 |
PASP (mm Hg) | 48.2 ± 18.2 | 46.3 ± 17.1 | .61 |
PVR (Wood units) | 4.1 ± 1.7 | 2.9 ± .90 | .001 |
EF (%) | 59 ± 10 | 64 ± 7 | .03 |
E/e′ ratio | 10 ± 4 | 11 ± 5 | .29 |
Basal RVFWS (%) | −17.4 ± 8.6 | −22.8 ± 7.6 | .002 |
Mid RVFWS (%) | −13.1 ± 8.4 | −19.6 ± 4.7 | <.001 |
Apical RVFWS (%) | −10.3 ± 5.4 | −14.4 ± 8.3 | <.007 |
RVFWS (%) | −13.6 ± 5.7 | −19.6 ± 4.7 | <.001 |
FAC (%) | 25.6 ± 11.8 | 37.7 ± 11.8 | <.001 |
RVEDA (cm 2 ) | 24.8 ± 6.1 | 21.02 ± 7.1 | .01 |
Echocardiography
Comparison between patients with PE and control subjects showed differences in PVR (higher in patients with PE) and all RV functional indices (reduced in patients with PE) ( Table 1 ). RV size (RVEDA) was increased in patients with PE. Figure 1 shows the pattern of RV regional strain.
LV ejection fraction showed a significant difference with the matched group (59 ± 10% vs 64 ± 7%, P = .03). Diastolic function between groups was similar.
McConnell Sign
Both the PE and PAH groups were evaluated for the presence of the McConnell sign. This sign was identified in 41 patients, 18 (40%) with PE and 23 (14%) with PAH ( Table 2 ). Generally, those presenting with the McConnell sign had higher heart rates, higher PVR, lower RVFWS, higher RVEDA, and lower FAC. Figure 2 shows the regional variation in strain between patients with and those without the McConnell sign. We created an RV free wall basal-mid/apical free wall ratio (RV apex/[average basal + mid] × 100). Patients who demonstrated the McConnell sign showed similar RV apical strain compared with those without this sign (59 ± 54% vs 52 ± 36%, P = .27), with the same finding when restricted to patients with PE (64 ± 78% vs 47 ± 25%, P = .33). RV strain showed the same pattern of reduction from base to apex, irrespective of afterload.
Variable | McConnell sign | P | |
---|---|---|---|
Present | Absent | ||
HR (beats/min) | 89 ± 19.5 | 76 ± 13.2 | <.001 |
PASP (mm Hg) | 53.9 ± 21 | 46.8 ± 22.5 | .07 |
EF (%) | 61 ± 8.8 | 65 ± 9.6 | .07 |
e′ | 6.0 ± 1.8 | 6.8 ± 2.3 | .13 |
E/e′ (%) | 12.5 ± 7.2 | 11.6 ± 4.8 | .45 |
PVR (Wood units) | 4.3 ± 2.3 | 3.2 ± 1.4 | .007 |
Basal RVFWS (%) | −17.3 ± 8.3 | −22.3 ± 8.4 | .001 |
Mid RVFWS (%) | −15.3 ± 9.9 | −19.8 ± 7.4 | .009 |
Apical RVFWS (%) | −12.9 ± 8.4 | −14.8 ± 8.2 | .18 |
RVFWS (%) | −15.2 ± 7.5 | −19.0 ± 5.5 | .004 |
RVEDA (cm 2 ) | 24.2 ± 7.0 | 20.0 ± 7.1± | .001 |
FAC (%) | 27.4 ± 10.9 | 36.2 ± 11.2 | <.001 |
RV apical-/mid+base ratio | 59.4 ± 54.2 | 51.6 ± 36.4 | .27 |
RV Longitudinal Function
RVFWS was significantly decreased in the PE group compared with the unmatched ( P < .001) and matched ( P < .001) groups. This pattern was noted in all segments of the RV free wall, with highly significant differences among the basal, mid, and apical segments ( P < .001) ( Figure 1 ).
The associations of RV dysfunction are shown in Table 3 . Univariate analysis showed that age, sex, PASP, acuity of afterload, RVEDA, and PVR were significantly associated with RVFWS. In multivariate models, even when taking into account PASP, acuity of afterload was still significantly associated with RV strain (odds ratio [OR], −5.7; 95% CI, −7.4 to 4.0; P < .001). When PASP was replaced by PVR, this association still held true (OR, −4.4; 95% CI, −6.0 to 2.1; P < .001). Acuity retained a significant association with global longitudinal strain when RVEDA was taken into account (OR, −3.9; 95% CI, −5.7 to 2.2; P < .001).
Variable | Univariate | PASP model | PVR model | RVEDA | ||||
---|---|---|---|---|---|---|---|---|
OR (95% CI) | P | OR (95% CI) | P | OR (95% CI) | P | OR (95% CI) | P | |
Age | −0.06 (−0.12 to −0.003) | .04 | −0.06 (−0.11 to −0.01) | .01 | −0.05 (−0.10 to −0.002) | .06 | −0.06 (−0.11 to −0.01) | .01 |
Sex | 2.10 (0.21 to 3.99) | .030 | 1.50 (−0.06 to 3.07) | .06 | 1.85 (0.27 to −3.44) | .02 | 0.37 (−1.27 to 2.01) | .66 |
PASP | 0.12 (0.08 to 0.15) | <.001 | 0.11 (0.08 to 0.15) | <.001 | ||||
PE (vs PHT) | −5.7 (−7.61 to −0.38) | <.001 | −5.7 (−7.4 to −4.0) | <.001 | −4.4 (−6.1 to −2.1) | <.001 | −3.9 (−5.7 to −2.15) | <.001 |
RVEDA | 0.45 (0.35 to 0.56) | <.001 | 0.38 (0.28 to 0.49) | <.001 | ||||
PVR | 1.81 (1.35 to 2.27) | <.001 | 1.49 (1.04 to 1.93) | <.001 |
The incremental value of each factor for discrimination of acuity using sequential logistic regression is shown in a series of models in Table 4 . Using standard clinical predictors, heart rate, PASP, and RVEDA were significantly associated with acuity (model χ 2 = 60.5, P < .001). The addition of PVR did not significantly increase the association (χ 2 = 63.0, P = .12), and PVR itself was not significant in the model (OR, 0.80; 95% CI, 0.60–1.06; P = .12). However, the addition of RVFWS was significant ( P < .001) and significantly increased the strength of the model (χ 2 = 70, P = .008).
Model 1 | Model 2 (plus PVR) | Model 3 (plus RV GLS) | P | |||
---|---|---|---|---|---|---|
Global χ 2 = 60.5 ( P < .001) | Global χ 2 = 63.0 ( P < .001) | Global χ 2 = 70.0 ( P < .001) | ||||
Increment P = .12 | Increment P = .008 | |||||
OR | P | OR | P | OR | ||
HR | 0.92 (0.89–0.95) | <.001 | 0.92 (0.89–0.95) | <.001 | 0.93 (0.90–0.97) | <.001 |
Age | 0.99 (0.95–1.01) | .159 | 0.97 (0.94–1.01) | .12 | 0.97 (0.93–1.00) | .05 |
Sex | 1.74 (0.67–4.52) | .252 | 1.69 (0.64–4.45) | .29 | 1.87 (0.70–5.05) | .214 |
PASP | 1.04 (1.02–1.07) | .002 | 1.05 (1.02–1.08) | .001 | 1.05 (1.02–1.09) | .001 |
RVEDA | 0.88 (0.81–0.94) | <.001 | 0.88 (0.81–0.94) | <.001 | 0.90 (0.83–0.97) | .01 |
PVR | 0.80 (0.60–1.06) | .12 | 0.86 (0.64–1.15) | .29 | ||
RV GLS | 0.89 (0.81–0.97) | .01 |
Validity of RV Functional Markers over a Spectrum of Measurements
The diagnostic strength of different RV systolic functional parameters may depend on the magnitude of RV failure. Receiver operating characteristic curve analysis was performed, with groups divided on the basis of quantification of RV systolic function; group 1 contained those with mildly reduced or normal FAC values (>35%), whereas group 2 contained those with moderately or severely reduced FAC. If FAC was normal, discriminative power between variables was poor ( Table 5 ). When RV systolic function was at least moderately reduced, RVFWS retained its predictive ability (AUC = 0.77). A second analysis was performed, with groups created on the basis of RVFWS values (normal or mildly reduced, <−20%, or moderate or severe RVFWS). Similar results were seen, with RVFWS the strongest predictor (AUC = 0.69) ( Table 5 ), although some diagnostic strength was lost.
Variable | AUC | 95% CI | P | AUC | 95% CI | P |
---|---|---|---|---|---|---|
FAC normal/mild | FAC abnormal | |||||
FAC | 0.54 | 0.37–0.71 | .65 | 0.43 | 0.28–0.57 | .29 |
RVFWS | 0.49 | 0.31–0.67 | .89 | 0.76 | 0.62–0.90 | <.001 |
RVEDA | 0.40 | 0.23–0.57 | .25 | 0.77 | 0.69–0.85 | <.001 |
RVFWS normal (<−20%) | RVFWS abnormal (>−20%) | |||||
FAC | 0.70 | 0.26–1.0 | .25 | 0.28 | 0.18–0.37 | <.001 |
RVFWS | 0.20 | 0.03–0.36 | .076 | 0.69 | 0.59–0.78 | .001 |
RVEDA | 0.81 | 0.68–0.94 | .07 | 0.60 | 0.50–0.70 | .06 |
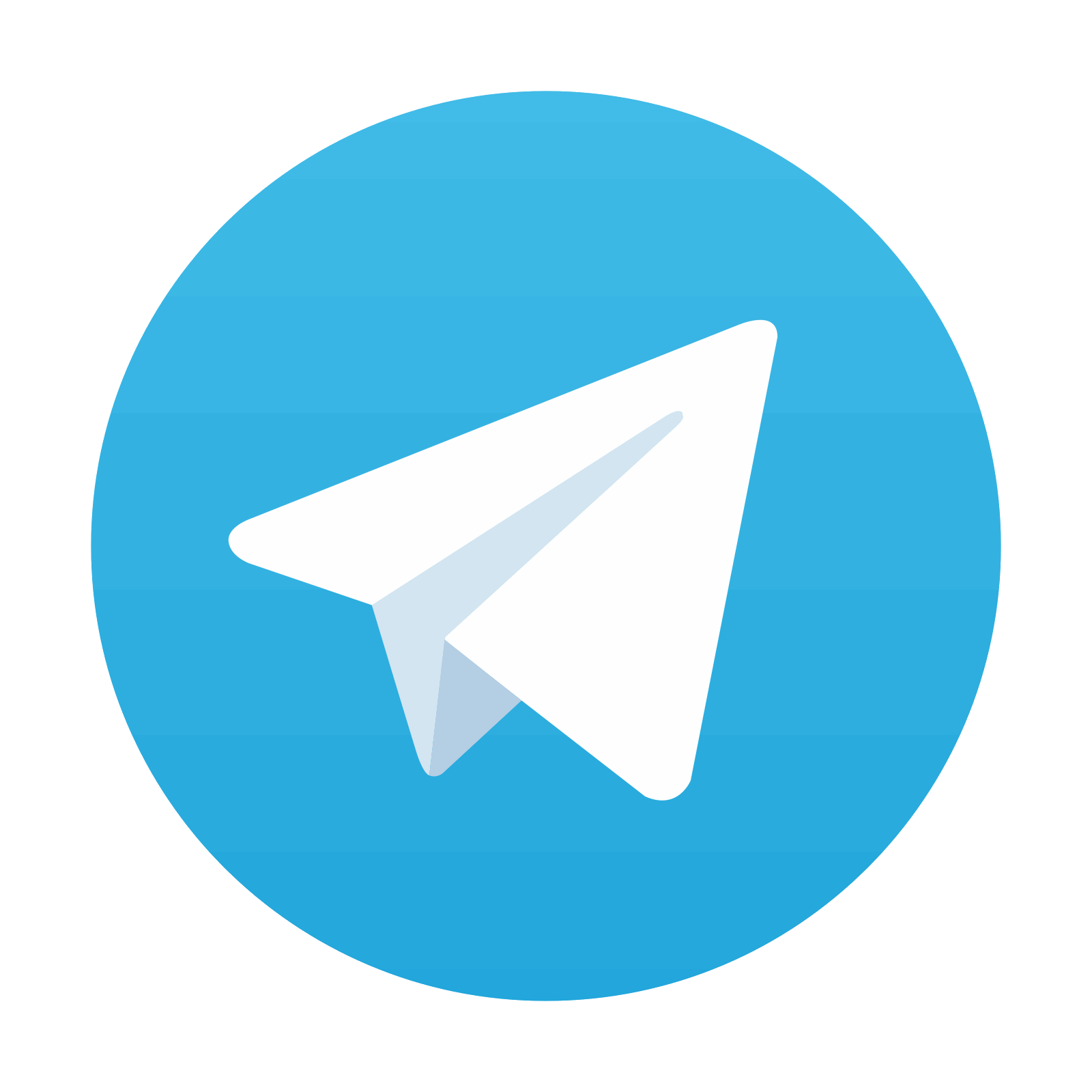
Stay updated, free articles. Join our Telegram channel

Full access? Get Clinical Tree
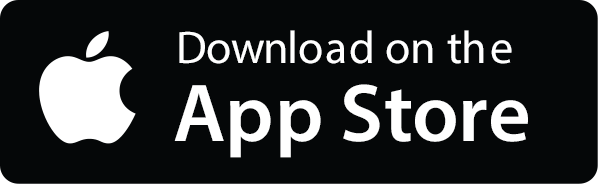
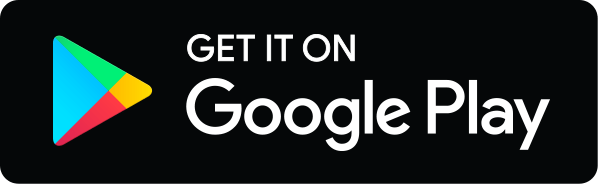
