Background
Pediatric dilated cardiomyopathy (DCM) is associated with death or transplantation and is typically considered a homogeneous process affecting global left ventricular (LV) function. However, assessment of regional abnormalities that contribute to pumping inefficiencies is lacking. The aim of this study was to define regional strain patterns of mechanical inefficiency in the broader context of LV discoordination (dysfunction and timing abnormalities) and to evaluate their associations with LV function and clinical outcomes.
Methods
Multiplanar apical LV echocardiographic images from patients with pediatric DCM ( n = 56) and control subjects ( n = 20) were analyzed by two-dimensional longitudinal speckle-tracking strain analysis to identify segmental strain patterns and global contraction groups. Clinical outcome (death or transplantation vs transplantation-free survival) and echocardiographic data were evaluated. Outcome groups were compared using the Fisher exact test, the χ 2 test, or analysis of variance (with P values ≤ .05 considered to indicate statistical significance).
Results
Of 56 patients with DCM, 29 (52%) progressed to death or transplantation. Five segmental strain patterns were observed that were used to define seven contraction groups by regional contractility and/or timing discoordination. The group(s) with the most discoordination had the most LV dysfunction ( P = .0004) and a trend toward the highest frequency of death or transplantation ( P = .069). Interreader reproducibility of segmental strain patterns agreed in 165 or 180 (92%) segments tested (κ = 0.90). Control subjects had normal strain patterns.
Conclusions
A heterogeneous mixture of abnormalities in the regional wall mechanics that lead to inefficient pump mechanics through functional and timing abnormalities were seen in this cohort and were categorized into natural subgroups. More severe LV discoordination was associated with increased LV dysfunction and a trend toward death or transplantation.
Children with dilated cardiomyopathy (DCM) have variable outcomes, with many demonstrating progression toward ventricular dilation and dysfunction leading to death or cardiac transplantation. Traditional echocardiographic measures of function and left ventricular (LV) size can help predict which patients will progressively decline and which will remain stable or undergo natural reverse remodeling. DCM is typically considered a process of global LV dysfunction, although LV regional wall motion abnormalities may also be present, leading to further contractile inefficiency. Of the few studies assessing the quantitative degree of discoordination in pediatric DCM, none have yet combined an analysis of segmental timing and functional abnormalities. In an overlapping pediatric DCM population, we previously reported on the frequency, characteristics, and QRS morphology of patients with a classic strain pattern of mechanical dyssynchrony caused by an underlying activation delay that may be responsive to cardiac resynchronization therapy (CRT). Although not reported in that study, frequent regional wall motion abnormalities that did not fit the classic pattern of electromechanical dyssynchrony were noted. In this study, we remove focus from electrical-mechanical interactions and instead evaluate the broader context of mechanical discoordination by describing the mechanical timing and functional heterogeneities that can contribute to inefficient contraction patterns.
Speckle-tracking strain echocardiography permits the analysis of regional function and timing of ventricular motion, with multiple studies demonstrating the utility of this analysis in pediatric and congenital heart disease. However, the majority of these studies have not included the identification of regional strain patterns in their analyses, instead concentrating on the simpler peak segmental or global strain or the dispersion of the time to peak regional strain. Conversely, pattern analysis defines segmental patterns of deformation on the basis of the shape of each strain curve rather than the absolute x axis (timing) or y axis (function) value, thereby defining the contractile behavior of specific cardiac regions throughout the cardiac cycle and providing important regional information that becomes a fundamental strength of strain imaging. We hypothesized that discrete patterns of regional wall motion can characterize contraction inefficiencies that may be associated with ventricular dysfunction and clinical outcomes. Accordingly, the goals of this study were to describe observed patterns of regional wall motion in children with DCM and to relate these to LV function and the clinical outcomes of transplant or death.
Methods
Study Subjects
This was a retrospective study of pediatric subjects with DCM with echocardiograms obtained using a standardized functional GE protocol, with apical imaging optimized for speckle-tracking strain analysis. Studies were obtained between September 2005 and October 2013 at the Hospital for Sick Children (Toronto, ON, Canada). Starting in 2006, functional-protocol echocardiograms were obtained in all children with DCM. These functional echocardiograms were usually obtained within 1 month of the initial diagnostic echocardiographic study (39 of 56 subjects [70%]). The median time from diagnostic to functional echocardiography in the other 17 subjects was 4 months (range, 1-125 months). Subjects with longer delays were diagnosed before 2005. We previously reported on the prevalence of a specific classic pattern of electromechanical dyssynchrony in an overlapping cohort of pediatric subjects with DCM. Inclusion criteria for subjects with DCM were increased LV end-diastolic diameter (LVEDD) Z score ≥ 2 and LV ejection fraction (LVEF) < 40% by two-dimensional (2D) echocardiography.
Functional echocardiograms from 20 control pediatric subjects, ordered for clinical indications of ventricular function or murmur, were also analyzed to define normal contraction patterns and for comparison with subjects with DCM. Control inclusion criteria were good imaging windows with normal cardiac anatomy and normal ventricular size and function (LVEF ≥ 55% and LVEDD Z score < 2).
Exclusion criteria for both the DCM and control groups were cardiac pacing, congenital anatomic heart disease, mitral valve surgery, atrial dysrhythmias, and frequent ectopy precluding the evaluation of consecutive sinus beats. Subjects were also excluded if they did not have four-chamber LV apical echocardiographic images of adequate quality. Demographics and clinical status were obtained from the medical record. This study was approved by the Hospital for Sick Children Research Ethics Board.
Two-Dimensional Echocardiography
Echocardiograms were obtained for clinical indications during outpatient clinic visits or inpatient hospitalization using a functional protocol with grayscale images optimized for longitudinal speckle-tracking strain analysis (median, 70 frames/sec; range, 50-130 frames/sec) using the GE Vivid E9 ultrasound scanner (GE Vingmed Ultrasound AS, Horten, Norway). The myocardium and endocardial border definition were optimized while maximizing frame rate with depth and sector arc adjustments. Because of patient age, breath holding was not consistently feasible. LV size was assessed by LVEDD Z score from the M-mode measurements using the Hospital for Sick Children’s institutional Z scores. The biplane Simpson method was used to measure LVEF. LV size and LVEF were recorded from the official echocardiographic report.
Echocardiographic Tissue Doppler Analysis
Using GE EchoPAC PC version BT11, in the DCM population, offline tissue Doppler analysis was performed on the three apical views (median, 124 frames/sec; range, 79-236 frames/sec). Mid and basal segment time to peak velocity was measured, and the 12-segment SD of the time to peak velocity (TsSD) was determined according to previously reported methodology as an index of contractile discoordination. Tissue Doppler images was not available for the control subjects.
Echocardiographic Speckle-Tracking Strain Analysis
The LV four-, three-, and two-chamber apical views were analyzed offline for 2D speckle-tracking strain in GE EchoPAC PC version BT11 after direct image file transfer from the ultrasound machine to EchoPAC at acquired frame rates. The LV endocardial border was traced in end-systole from the medial to the lateral mitral valve annulus for longitudinal speckle-tracking and the full-thickness region of interest adjusted to exclude the pericardium and papillary-chordal structures over one cardiac cycle. EchoPAC automatically analyzed the integrity of the speckle-tracking of each of the six segments, and the reader visually confirmed the adequacy of strain tracking. In case of poor tracking, the region-of-interest line or thickness was changed until it tracked adequately or was excluded for persistently inadequate tracking. The strain analysis for a single view was included if four or more segments were adequately tracked, including at least three mid and basal segments in each view. The QRS reference point was placed at the onset of the QRS to ensure consistency and inclusion of early pre-ejection events. Aortic valve closure timing was defined on spectral Doppler relative to QRS onset in an image with a similar heart rate. The software divided each view into six segments for an overall 18-segment global model that includes the three LV views. Global longitudinal peak systolic strain (PS) was reported as a mean average of all adequately tracked segments in all views.
Regional Pattern Identification of Segmental Strain Curves
For the regional pattern strain analysis, the morphology or shape of each segmental strain curve was examined. No preconceived criteria were used; rather, the patterns were described as observed. Overall, five patterns emerged from the data, and one of these patterns was identified for each segmental curve on the basis of the closest fit to that pattern’s criteria. The five regional patterns are detailed in the “Results” section ( Figure 1 , left , and Figure 2 ).


Categorization of Global Contraction Groups
A bull’s-eye model was created for each left ventricle using a color-coding system based on the five regional patterns and the regional PS. Using these measures, seven global contraction groups were identified with similar mechanical characteristics. Each left ventricle was then placed in one of these groups, giving priority to the distribution of regional patterns. Regional peak strain was used when a left ventricle could be considered in two different groups as additional information to guide best fit. The seven global contraction groups are detailed in the results section ( Figure 1 , right ).
Definitions
In this text, strain refers to LV longitudinal speckle-tracking systolic strain. Because longitudinal LV PS is a negative value (shortening in systole), the nomenclature in this report refers to greater strain as a larger negative number (e.g., peak strain of −13% has greater strain than −6%, or peak strain −13% > −6%).
For the purpose of this report, discoordination refers to any type of inefficient pumping mechanism or any ventricle that has regional wall motion abnormalities. The focus of this report is the various types of inefficient contraction physiology that result from different patterns of mechanical discoordination in this population. When we refer to electromechanical dyssynchrony, it is in the context of an underlying electrical activation delay that leads to mechanical dyssynchrony, which was the focus of our previous work.
Statistical Analysis
Continuous variables are reported as mean ± SD. Categorical variables are reported as number (percentage). Outcome groups were compared using the Fisher exact test, the χ 2 test, or analysis of variance as appropriate. P values < .05 were considered to indicate statistical significance. Statistical analyses were performed using SAS version 9.4 (SAS Institute Inc, Cary, NC). An interobserver comparison was performed on the segmental pattern analysis for 15 randomly selected subjects with DCM representing echocardiograms. After an initial discussion between the two observers to define the characteristics of each pattern, they independently categorized each segment as pattern A, B, C, D, or E in a blinded fashion. Interobserver reproducibility was assessed by agreement and the κ statistic between the two readers. Each observer performed independent strain tracking, analysis, and interpretation.
Results
DCM Study Population
Demographics are provided for 56 subjects with DCM in Table 1 . Three subjects with DCM were excluded (initially 59 subjects in the DCM population) because of inadequate four-chamber LV apical echocardiographic image quality. Idiopathic and familial DCM diagnoses represented the large majority of the subjects with DCM. Of the 56 subjects with DCM, 29 (52%) progressed to death or transplantation, with 23 transplantations (41%) and six deaths (11%). Across the entire cohort, the average length of follow-up from the initial echocardiogram analyzed for speckle-tracking strain was 27 months (range, 1–90 months). Older age at echocardiography was the only demographic variable to associate with death or transplantation (transplantation-free survivors, 3.1 ± 4.7 years; death or transplantation group, 6.3 ± 6.6 years; P = .045).
Variable | Value |
---|---|
Age (y) | 4.7 ± 5.9 (0–18) |
Infants | 30 (54%) |
Children | 17 (30%) |
Adolescents | 9 (16%) |
Female | 27 (48%) |
≥2 heart failure medications | 46 (82%) |
DCM diagnosis | |
Idiopathic | 34 (60%) |
Familial | 15 (27%) |
Postchemotherapy | 1 (2%) |
Postmyocarditis | 6 (11%) |
Traditional Echocardiographic Analysis
Table 2 displays the traditional echocardiographic data and strain data in the subjects with DCM. The majority of subjects with DCM had moderate to severe disease on the basis of LVEDD Z score and LVEF.
Variable | Value |
---|---|
LVEDD Z score | 6.6 ± 2.4 |
LVEF (%) | 23.0 ± 8.3 |
Global PS (%) | −7.5 ± 4.1 |
TsSD (msec) | 33.8 ± 12.8 |
Segmental strain patterns | |
(A) Normal timing | 231 (31%) |
(B) Holosystolic stretch | 94 (13%) |
(C) Inert (PS < −3%) | 60 (8%) |
(D) Early stretch–late contraction | 309 (42%) |
(E) Early terminated contraction | 44 (6%) |
Global contraction categories | |
(1) Homogenous | 3 (5%) |
(2) Septal hypokinesis | 3 (5%) |
(3) LV wall hypokinesis | 8 (14%) |
(4) Apical sparing | 14 (25%) |
(5) Chaotic | 12 (22%) |
(6) Paradoxical timing dispersion | 7 (13%) |
(7) Late timing dispersion | 9 (16%) |
Regional Strain Pattern Analysis
For all subjects, each of the 18 LV segments that tracked adequately was analyzed for segmental PS and defined as one of five different segmental strain patterns on the basis of the observed strain curve morphology. Illustrated (drawn) strain curves representing all five segmental patterns are shown in Figure 1 ( left ). Examples of actual strain curves from study subjects demonstrating each segmental pattern are shown in Figure 2 . The segmental pattern color-coding system established in Figure 1 ( left ) is carried through all other figures. To our knowledge, this type of individual segmental pattern analysis was first reported by Carasso et al . However, we did not use any predefined patterns. Instead, patterns were identified on the basis of evaluation of the strain curve shape and morphology. This allowed a more natural identification of the observed patterns that emerged from the data rather than attempting to “force” the curves into predefined categories. Each pattern defines specific timing and/or contractile abnormalities that allow insight into the pathophysiology of the various regions.
The normal timing pattern (pattern A) was defined by a discrete peak of the strain curve occurring near aortic valve closure. This pattern had a wide range of peak strain but demonstrated some degree of preserved contractility (PS > −3%) and no distinct early stretch. Some strain curves demonstrated early minor or small peaks on the systolic downslope of the main peak. These first peaks were not used to define the pattern if there was a second, higher amplitude peak and if the slope of the line going into the first, minor peak was similar to the slope coming out of that peak. The holosystolic stretch pattern (B) remained above the baseline throughout ≥90% of systole, with no distinct early or late active contraction identified. The inert pattern (C) remained below the baseline for the majority of systole but had no distinct peaks, and PS was < −3%. Like pattern B, this pattern did not have a distinct peak, although it did remain predominantly below the baseline. The early stretch–late contraction pattern (D) was defined by the peak of the strain curve occurring significantly after aortic valve closure, with early stretch noted. As with pattern A, the PS amplitude varied considerably in this category but was ≥ −3%. The early terminated contraction pattern (E) demonstrated a distinct peak of early shortening that terminated before 70% of systole, followed by stretching or a smaller second peak. To be classified as pattern E, the second peak (if present) had to be lower in amplitude than the first, and the slope of the strain curve going into the first peak had to be substantially different from the slope after the first peak.
The most common patterns were the early stretch–late contraction (42%) and normal timing (31%) patterns ( Table 2 ). The least common was early terminated pattern (6%). Segmental strain pattern frequency and peak strain were plotted on a bull’s-eye plot ( Figure 3 ) to demonstrate the pattern distribution by region. Overall, peak strain was most preserved in the apical segments. The apical segments also had the lowest frequency of holosystolic stretch and inert patterns.

Global Contraction Categories
Segmental strain patterns were graphically displayed as an 18-segment bull’s-eye plot for each subject with DCM and combined with segmental peak strain to identify and define global ventricular contraction categories ( Figure 1 , right ). We identified seven contraction categories representing different types of LV pump inefficiencies, which arose naturally from observation of the data. All 56 bull’s-eye plots were organized by their overall ventricular contraction category, as shown in Figure 4 . The frequency of each group ( Table 2 ), association with outcome ( Table 3 ), and association with LV function and quantitative discoordination ( Table 4 ) are reported.

Characteristic | DCM transplantation-free survivors ( n = 27) | DCM death or transplantation ( n = 29) | P |
---|---|---|---|
Echocardiographic data | |||
LVEDD Z score | 6.3 ± 2.3 | 7.0 ± 2.5 | .31 |
LVEF (%) | 26.6 ± 7.8 | 19.6 ± 7.3 | .0012 |
Global LV PS (%) | −8.6 ± 4.6 | −6.4 ± 3.2 | .049 |
TsSD (msec) | 33.9 ± 13.1 | 33.8 ± 12.8 | .97 |
Global contraction category | |||
(1) Homogenous | 1 (33%) | 2 (67%) | .60 |
(2) Septal hypokinesis | 2 (67%) | 1 (33%) | .51 |
(3) LV wall hypokinesis | 5 (62%) | 3 (38%) | .46 |
(4) Apical sparing | 8 (57%) | 6 (43%) | .44 |
(5) Chaotic | 3 (25%) | 9 (75%) | .069 |
(6) Paradoxical timing dispersion | 4 (57%) | 3 (43%) | .81 |
(7) Late timing dispersion | 4 (44%) | 5 (56%) | .61 |
Global contraction category | n | Global LV PS (%) | TsSD (msec) | LVEF (%) |
---|---|---|---|---|
(1) Homogenous | 3 | −11.0 ± 3.9 | 25.1 ± 1.2 | 33.7 ± 6.7 ∗ |
(2) Septal hypokinesis | 3 | −8.2 ± 2.9 | 29.3 ± 12.8 | 20.7 ± 4.7 |
(3) LV wall hypokinesis | 8 | −7.3 ± 2.9 | 29.8 ± 8.2 | 24.5 ± 7.2 |
(4) Apical sparing | 14 | −4.9 ± 3.0 ∗ | 34.6 ± 7.3 | 19.6 ± 6.3 † |
(5) Chaotic | 12 | −5.0 ± 3.8 ∗ | 39.6 ± 14.8 † | 16.8 ± 6.0 ∗ |
(6) Paradoxical timing | 9 | −9.9 ± 3.6 ∗ | 37.5 ± 7.5 | 28.1 ± 8.1 ∗ |
(7) Late timing | 7 | −11.5 ± 2.6 ∗ | 28.6 ± 24.9 | 28.4 ± 8.0 † |
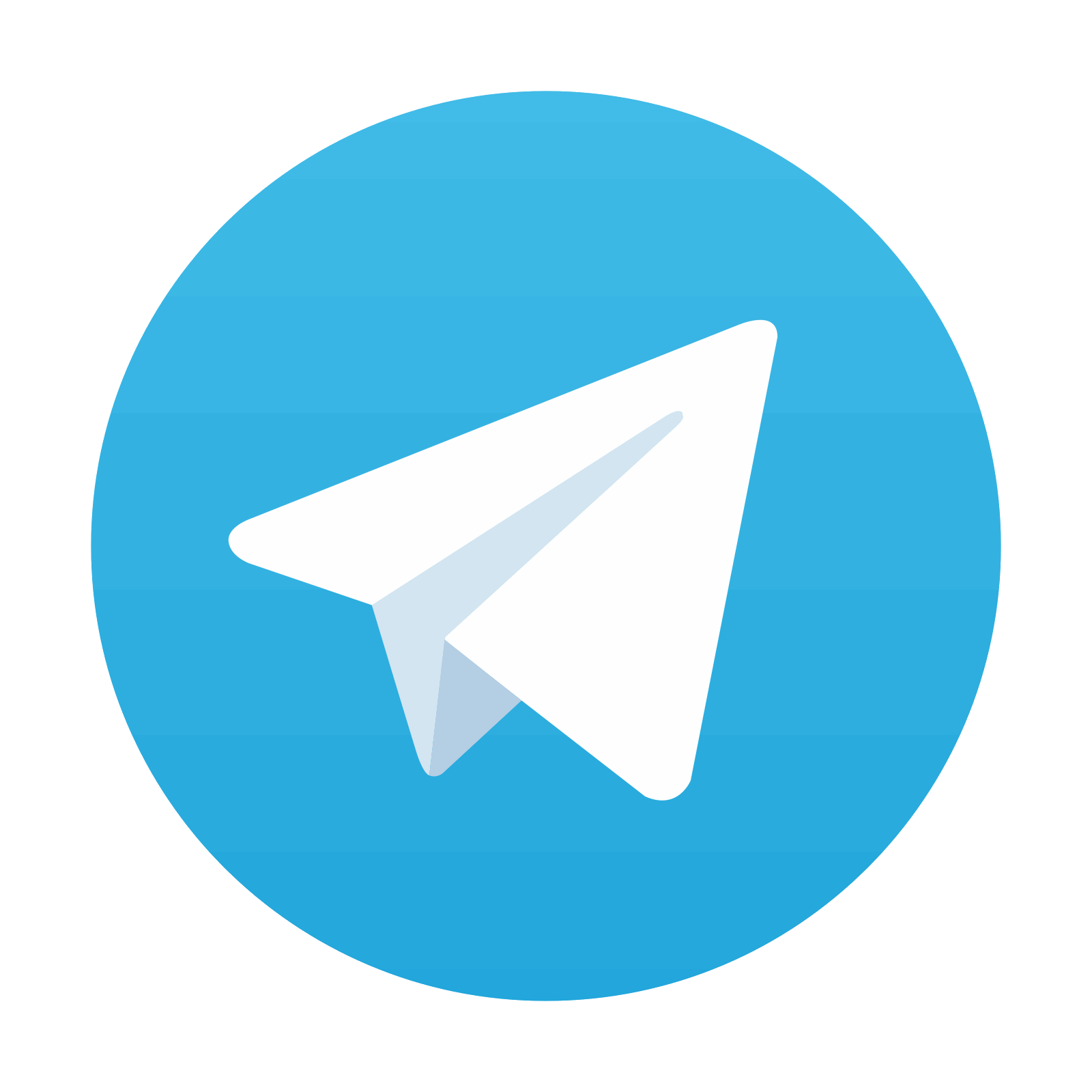
Stay updated, free articles. Join our Telegram channel

Full access? Get Clinical Tree
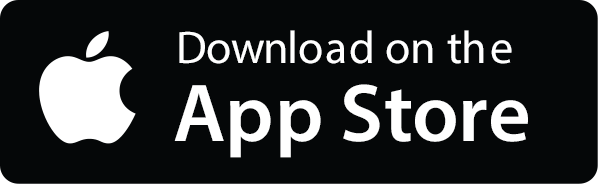
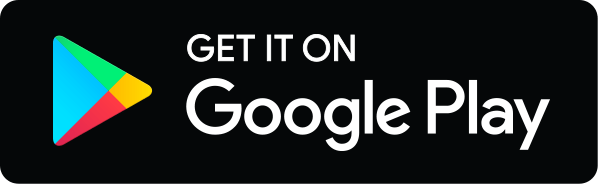
