Background
The optimal right ventricular pacing site remains controversial. The aim of this study was to assess how acute right ventricular outflow tract (RVOT) pacing affects global left ventricular function and intraventricular dyssynchrony of the left ventricle.
Methods
Thirty-six patients with sick sinus syndrome and intact intrinsic atrioventricular conduction were enrolled. All patients underwent dual-chamber permanent pacemaker implantation, with the atrial lead placed in the right atrial appendage and the right ventricle lead positioned at the septal site of the RVOT. Chamber size, dyssynchrony index, myocardial performance index, and global left ventricular ejection fraction were determined using transthoracic two-dimensional echocardiography, tissue Doppler echocardiography, and real-time three-dimensional echocardiography.
Results
RVOT pacing increased the myocardial performance index (0.42 ± 0.21 with RVOT pacing vs 0.35 ± 0.21 without RVOT pacing, P = .002) and decreased the global left ventricular ejection fraction on real-time 3-dimensional echocardiography (51.4 ± 6.2% with RVOT pacing vs 55.9 ± 7.1% without RVOT pacing, P = .001). Intraventricular dyssynchrony of the left ventricle induced by RVOT pacing was determined by increased septal-to-posterior wall motion delay (69.7 ± 54.0 ms with RVOT pacing vs 22.8 ± 22.3 ms without RVOT pacing, P < .0001), increased systolic and diastolic dyssynchrony by tissue Doppler echocardiography, and increased systolic dyssynchrony index when assessed using real-time three-dimensional echocardiography (5.56 ± 1.74% with RVOT pacing vs 4.05 ± 1.61% without RVOT pacing, P < .0001).
Conclusion
Acute RVOT pacing adversely affects left ventricular function and increases intraventricular dyssynchrony in patients with sick sinus syndrome.
Right ventricular (RV) apical pacing may induce left atrial dilation and increase heart failure and atrial fibrillation. The Mode Selection Trial (MOST) identified a strong correlation between the cumulative percentage of RV apical pacing and the incidence of hospitalization for congestive heart failure in patients with sick sinus syndrome (SSS) who had dual-chamber pacemakers. RV outflow tract (RVOT) pacing, compared with RV apical pacing, can reduce left ventricular activation time and the incidence of myocardial perfusion defects and may preserve left ventricular function. Thus, RVOT pacing was initially proposed as a favorable alternative pacing site for patients undergoing permanent pacemaker implantation. However, to our knowledge, global left ventricular mechanical dyssynchrony induced by acute RVOT pacing has never been investigated using real-time three-dimensional (3D) echocardiographic (RT3DE) imaging.
Notably, RT3DE imaging has proven to be an effective tool for assessing global left ventricular mechanical dyssynchrony. The aim of this study was to investigate the impact of acute RVOT pacing on left ventricular mechanical dyssynchrony and global left ventricular function by RT3DE in patients with SSS who underwent dual-chamber pacemaker implantation. To compare RVOT pacing with intrinsic conduction in the same patient and for adequate atrial rate control, we enrolled only those patients with SSS with intact intrinsic atrioventricular conduction.
Methods
Patient Population
We enrolled 36 patients with SSS with implanted dual-chamber permanent pacemakers who had normal atrioventricular and intraventricular conduction. All atrial leads were placed in the right atrial appendage, and all ventricular leads were located on the septal site of the RVOT. Lead position was confirmed using standard criteria. The optimal RVOT lead position was confirmed by biplane x-ray and 12-lead electrocardiography. Patients with histories of myocardial infarction, left bundle branch block, persistent atrial fibrillation, valvular heart disease, or congestive heart failure were excluded. The mean age of the 36 patients (7 [19.4%] men, 29 [80.6%] women) was 76 ± 10 years (range, 54-95 years). In total, 24 patients (66.7%) had hypertension, 9 patients (25.0%) had chronic kidney disease, 8 patients (22.2%) had dyslipidemia, 5 patients (13.9%) had diabetes mellitus, and 4 patients (11.1%) had coronary artery disease. Fifteen patients took calcium channel blockers, and 16 patients took angiotensin-converting enzyme inhibitors or type 1 angiotensin II receptor blockers. Normal controls were 16 healthy volunteers in normal sinus rhythm. Informed consent was obtained from all study subjects. The institutional review committee on human research at our institution approved this study.
Study Protocol
All patients had a programmed constant pacing rate of 70 beats/min at the time of echocardiography to ensure continuous right atrial pacing. All echocardiographic measurements were obtained initially during right atrial pacing with intrinsic atrioventricular conduction. Five minutes later, these tests were followed by atrial and RVOT pacing (with an atrioventricular delay of 120 ms to ensure full ventricular capture).
Echocardiographic Techniques
Transthoracic two-dimensional (2D) echocardiography and Doppler tissue imaging were performed using a Sonos 7500 (Live 3D Echo; Philips Medical Systems, Andover, MA) with an S3 transducer, and echocardiographic data were obtained soon after pacemaker implantation in patients with SSS (range, 1-18 days). Left atrial and ventricular dimensions and septal-to-posterior wall motion delay were determined using conventional M-mode echocardiography. Pulsed-wave Doppler measured the myocardial performance index, an index combining systolic and diastolic myocardial performance, and the mitral and aortic time-velocity integrals.
Doppler tissue imaging used 3 standard apical views for long-axis motion of the ventricle, as previously described. At a minimum, 3 consecutive beats were stored with digital loops for offline analysis using QLAB version 2.0 (Philips Medical Systems). Myocardial pulse Doppler velocity profile signals were reconstructed offline from Doppler tissue color images showing regional myocardial velocity curves. The color-coded area and settings of the echocardiographic equipment were adjusted to acquire the highest possible frame rate (minimum frame rate, 90 Hz) to optimize Doppler tissue color images. Times to peak myocardial sustained systolic velocity (Ts) and early diastolic velocity (Te) were determined for each of the 12 nonapical segments. Synchronicity was assessed by calculating the standard deviation of Ts (Ts-SD) and Te (Te-SD) of all 12 left ventricular segments. The averages of ≥3 consecutive beats were used for comparison.
The RT3DE approach obtained a pyramidal volume in real time using an X4 matrix transducer. This transducer has 3000 active elements sending and receiving simultaneously, resulting in a real-time 3D volume. For acquisition of a full-volume data set, 4 smaller real-time volumes (ie, 4 live 3D volumes) acquired from alternate cardiac cycles were combined to provide a relatively larger pyramidal volume (up to 90° × 90°). Full-volume acquisition was performed while subjects held their breath and required a relatively stable R-R interval to minimize translational artifacts between the 4 acquired subvolumes. Apical full-volume acquisitions of the left ventricle were acquired for all patients. To optimize the acquisition frame rate, depth was minimized to include only the mitral and aortic valves, the positions of which were required to determine spatial orientation in subsequent analyses. In patients with markedly dilated left ventricles, the scan-line density was reduced to increase the pyramidal volume acquired. Quantitative analysis by four-dimensional left ventricular analysis software (Research-Arena 4D LV-Analysis; TomTec Imaging Systems, Munich, Germany) involved defining several 2D slices through the voxel-based 3D data set. The endocardial border in each slice was traced using a semiautomated detection process, and a “cast” of the left ventricular cavity was generated as a mathematical model, providing time-volume data for an entire cardiac cycle. By dividing this volume into pyramidal subvolumes based around a nonfixed central point, this study estimated time-volume data for each of the 17 standard myocardial segments defined by the American Heart Association. The RT3DE data sets were used for time-volume analysis for the determination of global and segmental left ventricular volumes ( Figure 1 ). The dyssynchrony indices by RT3DE imaging were derived by calculating the time taken to reach the minimum regional volume for each segment as a percentage of the cardiac cycle. The systolic dyssynchrony index was defined as the standard deviation of these time periods. A high systolic dyssynchrony index indicated increased intraventricular dyssynchrony. Additionally, regional wall motion assessment in the longitudinal planes of the left ventricle by RT3DE with and without RVOT pacing was also performed at the anterior (segments 1, 7, and 13 of the left ventricle), septal (segments 2, 3, 8, 9, and 14 of the left ventricle), posterior (segments 4, 10, and 15 of the left ventricle) and lateral (segments 5, 6, 11, 12, and 16 of the left ventricle) walls of the left ventricle. Furthermore, radial analysis of the left ventricle by RT3DE imaging with and without RVOT pacing was conducted at the basal (segments 1-6), mid (segments 7-12), and apical (segments 13-16) levels of the left ventricle. Likewise, the systolic dyssynchrony index in longitudinal and radial analyses was defined as the standard deviation of time needed to reach the minimum regional volume for each segment at each wall or each ventricular level as a percentage of a cardiac cycle.

For intraobserver analysis of the RT3DE data, 26 random measurements were reanalyzed, with a period of 4 to 5 weeks between the first and second analysis. Intraclass correlation coefficients for intraobserver agreement were 0.914 and 0.911 for the systolic dyssynchrony index and 3D left ventricular ejection fraction, respectively. For interobserver analysis of the RT3DE data, 12 random measurements were analyzed. The intraclass correlation coefficients for interobserver agreement were 0.873 and 0.891 for the systolic dyssynchrony index and 3D left ventricular ejection fraction, respectively.
Statistical Analysis
Data are expressed as mean ± SD or as percentages. Categorical variables between patients with SSS and healthy volunteers were compared using Fisher’s exact test (2 tailed). Continuous variables between patients with SSS and healthy volunteers were compared using the Mann-Whitney U test. Continuous variables within the same group were compared using Wilcoxon’s signed-rank test for paired samples. Correlations between the systolic dyssynchrony index derived by RT3DE imaging and other echocardiographic parameters in patients with SSS with RVOT pacing were determined using Spearman’s rank correlation test. Statistical analyses were performed using SPSS version 12.0 (SPSS, Inc, Chicago, IL). All P values were 2 tailed, and P values < .05 were considered statistically significant.
Results
Patient Characteristics
Table 1 lists the clinical characteristics of the study patients. The patients with SSS and healthy volunteers did not differ significantly in age, sex, body mass index, or prevalence of diabetes mellitus, hypertension, or coronary artery disease and were balanced in terms of use of drugs such as calcium channel blockers and β-blockers. However, patients with SSS had longer baseline sinus cycle lengths than healthy volunteers.
Variable | Study group (n = 36) | Control group (n = 16) | P |
---|---|---|---|
Age (y) | 76 ± 10 | 69 ± 15 | .279 |
Men | 7 (19.4%) | 5 (31.3%) | .478 |
Body mass index (kg/m 2 ) | 24.4 ± 3.5 | 23.6 ± 3.2 | .465 |
Diabetes mellitus | 5 (13.9%) | 0 (0.0%) | .308 |
Hypertension | 24 (66.7%) | 7 (43.8%) | .120 |
Dyslipidemia | 8 (22.2%) | 5 (33.3%) | .506 |
Coronary artery disease | 4 (11.1%) | 0 (0.0%) | .299 |
Sinus cycle length (ms) | 1102.6 ± 386.9 | 856.0 ± 163.3 | .012 |
QRS duration (ms) | 91.5 ± 18.7 | 91.3 ± 9.8 | .437 |
PR interval (ms) | 184.3 ± 56.0 | 162.9 ± 24.7 | .359 |
Medications | |||
β-blockers | 6 (16.7%) | 5 (31.3%) | .281 |
Calcium channel blockers | 15 (41.7%) | 5 (31.3%) | .476 |
Table 2 lists differences in echocardiographic parameters between patients with SSS during atrial pacing and healthy volunteers. The patients with SSS during atrial pacing and healthy volunteers did not differ significantly in left ventricular end-diastolic diameter, global left ventricular ejection fraction, myocardial performance index, and mechanical dyssynchrony indices, such as septal-to-posterior wall motion delay by M-mode echocardiography, Ts-SD and Te-SD by Doppler tissue imaging, and systolic dyssynchrony index by 3D echocardiography.
Variable | Study group (n = 36) | Control group (n = 16) | P |
---|---|---|---|
2D echocardiography | |||
LA size (mm) | 31.7 ± 7.0 | 28.6 ± 3.8 | .242 |
LVEDD (mm) | 45.8 ± 6.2 | 47.4 ± 3.2 | .410 |
SPWMD (ms) | 22.78 ± 22.25 | 16.25 ± 22.47 | .111 |
MPI | 0.35 ± 0.21 | 0.33 ± 0.09 | .766 |
TVI at mitral valve (cm) | 20.85 ± 4.69 | 23.03 ± 6.61 | .312 |
TVI at LVOT (cm) | 21.96 ± 4.41 | 24.35 ± 4.56 | .06 |
3D echocardiography | |||
SDI (%) | 4.05 ± 1.61 | 4.67 ± 1.53 | .178 |
3D LVEDV (mL) | 80.22 ± 21.78 | 84.7 ± 25.59 | .648 |
Global LVEF by RT3DE imaging (%) | 56.0 ± 7.1 | 58.3 ± 6.89 | .242 |
Tissue Doppler echocardiography | |||
Ts-SD (ms) | 24.88 ± 16.96 | 32.37± 19.13 | .160 |
Te-SD (ms) | 21.40 ± 13.33 | 13.27 ± 10.36 | .101 |
Acute Effects of RVOT Pacing on Echocardiographic Parameters of Patients With SSS
QRS duration was significantly longer with RVOT pacing than without RVOT pacing (151.2 ± 13.1 vs 92.2 ± 16.8 ms, P < .0001). Table 3 lists the differences in echocardiographic parameters between patients with SSS with and without RVOT pacing. No significant change existed in left atrial dimension, left ventricular dimension, and left ventricular volume determined by RT3DE imaging. The myocardial performance index was significantly higher ( P = .002) and global left ventricular ejection fraction derived by RT3DE imaging significantly lower ( P = .001) with than without RVOT pacing, indicating that RVOT pacing decreased left ventricular function. Thus, RVOT pacing induced a 8.1% decrease in the left ventricular ejection fraction derived by RT3DE imaging relative to the baseline left ventricular ejection fraction without RVOT pacing. A significant difference existed between intraventricular dyssynchrony with and without RVOT pacing when assessed by septal-to-posterior wall motion delay at the midventricular level ( P < .0001). Ts-SD and Te-SD with RVOT pacing were significantly prolonged compared with those without RVOT pacing ( P < .0001). Global intraventricular contraction dyssynchrony was significantly longer with RVOT pacing than without RVOT pacing when assessed by the RT3DE systolic dyssynchrony index ( P < .0001; Figure 2 ). Thus, RVOT pacing induced a 37.3% increase in the global systolic dyssynchrony index from the baseline systolic dyssynchrony index without RVOT pacing, and this change should be clinically relevant. Additionally, RVOT pacing induced a significant 57.8% increase in the longitudinal systolic dyssynchrony index in the septal wall of the left ventricle compared with no RVOT pacing ( P = .022; Figure 3 , Table 4 ). Furthermore, on radial level analysis, RVOT pacing induced a significant 49.0% increase in the radial systolic dyssynchrony index at the midventricular level of the left ventricle ( P < .0001; Figure 4 ) and a significant 51.8% increase in the radial systolic dyssynchrony index at the apical level of the left ventricle ( P = .004; Figure 5 ) compared with those without RVOT pacing ( Table 5 ). Moderately strong correlations existed between the systolic dyssynchrony index derived by RT3DE imaging and global left ventricular ejection fraction ( r = −0.483, P = .003) and between the systolic dyssynchrony index derived by RT3DE imaging and the myocardial performance index ( r = 0.419, P = .012) in patients with SSS with RVOT pacing ( Table 6 ).
Variable | Without RVOT pacing | With RVOT pacing | P |
---|---|---|---|
2D echocardiography | |||
LA size (mm) | 31.7 ± 7.0 | 32.1 ± 6.6 | .567 |
LVEDD (mm) | 45.8 ± 6.2 | 45.6 ± 7.6 | .860 |
MPI | 0.35 ± 0.21 | 0.42 ± 0.21 | .002 |
TVI at mitral valve (cm) | 20.85 ± 4.69 | 20.21 ± 4.66 | .413 |
TVI at LVOT (cm) | 21.96 ± 4.41 | 20.99 ± 4.14 | .091 |
3D echocardiography | |||
LVEDV (mL) | 80.22 ± 21.78 | 77.48 ± 23.99 | .251 |
Global LVEF (%) | 56.0 ± 7.1 | 51.4 ± 6.2 | .001 |
Dyssynchrony | |||
SPWMD (ms) | 22.78 ± 22.25 | 69.72 ± 54.01 | <.0001 |
SDI (%) | 4.05 ± 1.61 | 5.56 ± 1.74 | <.0001 |
Tissue Doppler echocardiography | |||
Ts-SD (ms) | 24.88 ± 16.96 | 47.17 ± 19.59 | <.0001 |
Te-SD (ms) | 21.40 ± 13.33 | 43.74 ± 29.63 | <.0001 |
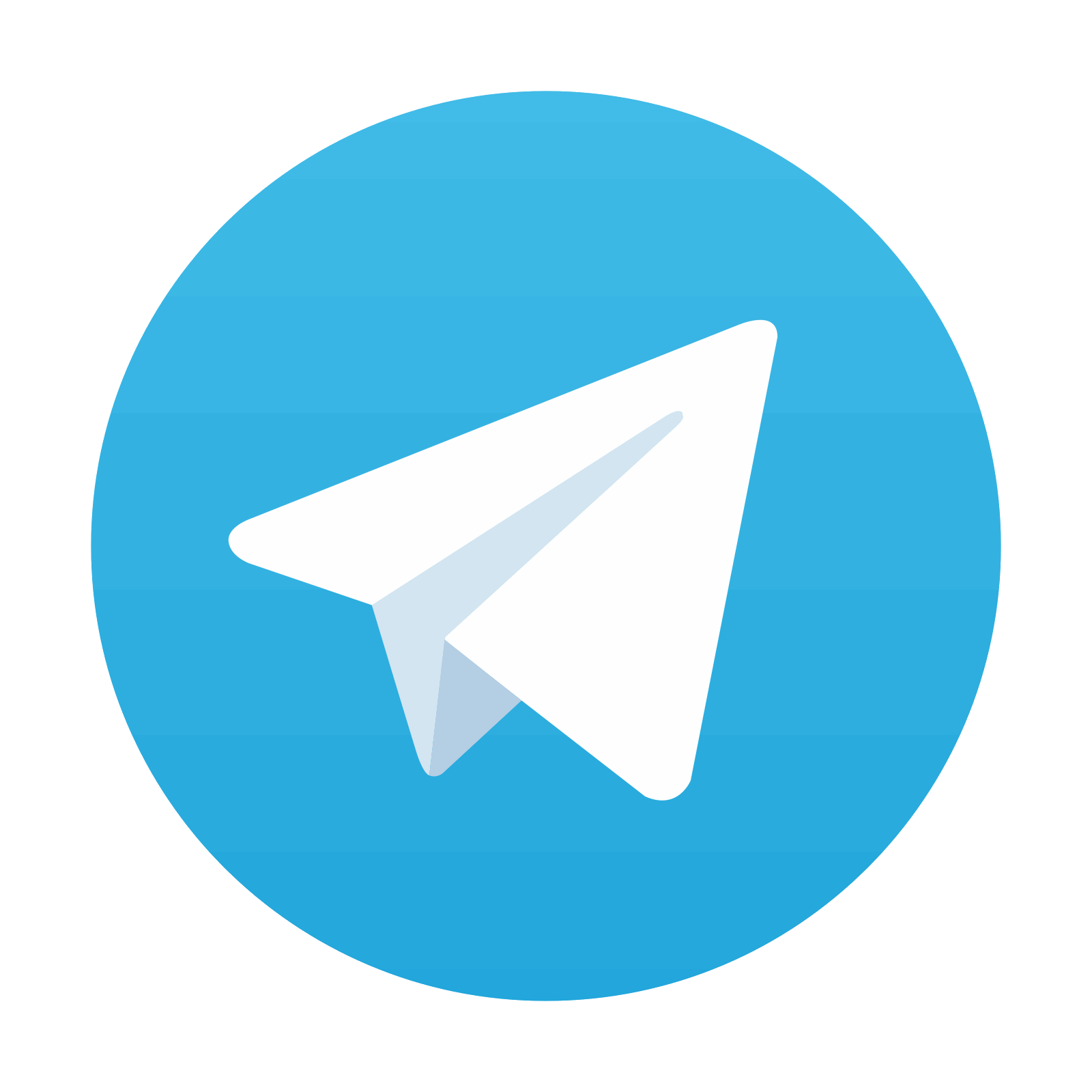
Stay updated, free articles. Join our Telegram channel

Full access? Get Clinical Tree
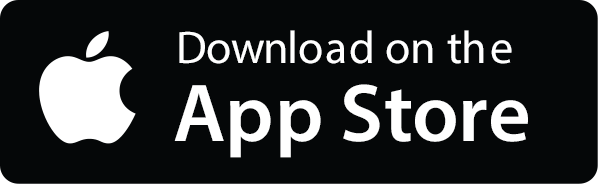
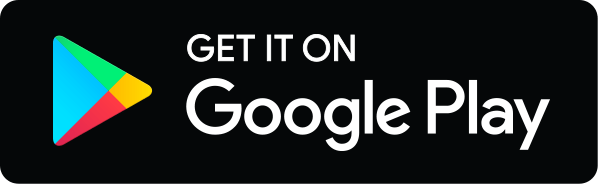
