Background
The mechanism of sudden death in hypertrophic cardiomyopathy (HCM) is ventricular tachyarrhythmia emanating from myocyte disarray, fibrosis, and inhomogeneity in intramyocardial activation. Tissue synchronization imaging (TSI) allows the measurement of regional delay, while two-dimensional strain can be used to identify myocardial fibrosis. The aim of this study was to assess the relationship between new ultrasonically derived parameters and nonsustained ventricular tachycardia (NSVT) in patients with HCM.
Methods
Ninety-three patients with HCM (mean age, 36 ± 16 years) and 30 patients with hypertension with secondary left ventricular (LV) hypertrophy (mean age, 42 ± 10 years; 65% men) were studied. All underwent standard echocardiographic, TSI, and two-dimensional strain examinations. Patients were followed every 3 months for 2 years. Holter monitoring was performed every 3 months. The primary endpoint was the occurrence of NSVT.
Results
Twenty-four patients (26%) had ≥1 episode of NSVT. Patients with NSVT had a higher value of maximal LV thickness (22 ± 6 vs 19 ± 5 mm, P = .04). There were no significant associations between NSVT on Holter monitoring and LV outflow gradient, New York Heart Association class, syncope, and medical therapy. N-terminal pro–brain natriuretic peptide values were significantly ( P = .01) higher in patients with NSTV (1034 ± 1088 vs 561 ± 593 pg/mL). Patients with HCM and NSVT had (1) similar values on TSI-studied parameters to patients without NSVT, (2) significant reductions in basal and mid septal strain and in basal anterior-septal strain, and (3) more frequently peak systolic strain ≥ −10% ( P < .0001). In multivariate analysis, the presence of >3 LV segments with longitudinal two-dimensional strain ≥ −10% (sensitivity, 81%; specificity, 97.1%; area under the curve, 0.944; P < .0001) was an independent predictor of NSVT.
Conclusions
Using a simple, inexpensive, easily available, and bedside-usable tool, it was possible to recognize with good sensitivity and specificity patients with HCM at higher risk for NSVT.
Hypertrophic cardiomyopathy (HCM) is the most common cause of sudden cardiac death in the young. The mechanism of sudden death in HCM is ventricular tachyarrhythmia emanating from myocyte disarray, fibrosis, and inhomogeneity in intramyocardial activation.
Obtained from Doppler tissue imaging, tissue synchronization imaging (TSI) is a new echocardiographic modality able to portray regional asynchrony on two-dimensional (2D) echocardiography. TSI transforms the timing of regional peak velocity into color codes, which allows the quantitative measurement of regional delay.
Several studies have demonstrated the ability of strain echocardiography in identifying myocardial dysfunction corresponding to areas of fibrosis in different structural heart disease.
Initially obtained from Doppler-derived velocity measurements, strain has been proposed as a sensitive tool to detect early systolic functional abnormalities in patients with HCM. Recently, a novel method to measure strain from standard 2D images has been developed, overcoming limitations of the Doppler-derived strain.
Therefore, the objective of the present study was to assess the value of these new ultrasonically derived techniques in predicting nonsustained ventricular tachycardia (NSVT) evident on 24-hour ambulatory Holter electrocardiographic monitoring in patients with HCM.
Methods
Patients
Between May 2006 and December 2009, we prospectively examined patients with HCM, who are regularly followed at our outpatient unit. The study was approved by the local ethics committee. Patients were enrolled in the study after their written informed consent was obtained.
Asymmetrical HCM was diagnosed with a septal thickness >15 mm and a septal/posterior wall thickness ratio >1.3 and concentric HCM with septal and posterior wall thickness >13 mm without any other cardiac or systemic disease capable of producing the magnitude of hypertrophy evident.
Apical HCM was defined in presence of asymmetric myocardial hypertrophy of the apex of the left ventricle (apex/posterior wall > 1.5).
In pediatric patients, HCM was diagnosed in the presence of a left ventricular (LV) maximal wall thickness at end-diastole > 2 SDs above the normal mean for body surface area.
Eight patients with implantable cardioverter-defibrillators were included in the study population, taking care during the echocardiographic acquisition not to visualize the device leads, to preserve blind postprocessing.
Exclusion criteria were diabetes mellitus, arterial hypertension, coronary artery disease, sinus tachycardia, atrial fibrillation on during the study evaluation, lung disease, New York Heart Association (NYHA) class ≥ III, prior surgical or catheter intervention, and inadequate echocardiograms.
Of the initial 150 patients with HCM, 57 were excluded from the study, 27 because they did not meet the inclusion criteria and 30 because not all required echocardiographic views were adequately obtained. As a result, in our selected final population of 93 patients with HCM, all required echocardiographic measurements were obtained.
In addition, 30 subjects (mean age, 42 ± 10 years) affected by essential arterial hypertension for ≥ 3 years were included in the echocardiographic evaluation (the LV hypertrophy [LVH] group). All patients with hypertension underwent antihypertensive therapy: 6 (20%) had triple combinations (2 [7%] with angiotensin-converting enzyme [ACE] inhibitors, β-blockers, and diuretics; 2 [7%] with angiotensin II antagonists, β-blockers, and diuretics; and 2 [7%] with ACE inhibitors, α-antagonists, and diuretics), 8 (27%) had double combinations (5 [17%] with ACE inhibitors and diuretics, 2 [7%] with angiotensin II antagonists and diuretics, and 1 [3%] with an angiotensin II antagonist and a β-blocker), and 16 (53%) received monotherapy (10 [33%] with ACE inhibitors, 4 [13%] with β-blockers, and 2 [6%] with calcium antagonists). The mean treatment period was 3.6 ± 1.6 years. Patients with diabetes mellitus, coronary artery disease, atrial fibrillation, NYHA class ≥ III, prior surgical or catheter coronary intervention, and inadequate echocardiograms were excluded.
Procedures
Standard Doppler echocardiography, TSI, and 2D strain were performed with the subjects in the partial left decubitus position using a Vivid 7 ultrasound system (GE Vingmed Ultrasound AS, Horten, Norway) equipped with 2D strain capabilities. A variable-frequency phased-array transducer (2.5, 3.5, and 4.0 MHz) was used for 2D, M-mode, and Doppler imaging. Doppler echocardiographic and 2D images were recorded on DVDs. All the measurements were analyzed by two experienced readers, taking the average of 3 cardiac cycles.
M-Mode and B-Mode
Two-dimensional measurements of septal and lateral wall thickness were obtained at end-diastole, in the parasternal short-axis view, at the mid cavity level by M-mode and integrated with parasternal long-axis and apical views. The measurement of LV wall maximal thickness was done using 2D echocardiography. LV ejection fraction was measured using a commercially available software program that applied Simpson’s rule on the 2-chamber and 4-chamber views.
LV mass was calculated using 2D echocardiography and indexed for body surface area. Relative wall thickness was defined as the ratio between the sum of the interventricular septum (IVS) and posterior wall thickness and the LV end-diastolic dimension.
Standard Doppler
Pulsed Doppler assessment of LV inflow was performed in apical 4-chamber view, with the sample volume placed at the level of the valve tips. The following measurements of global LV diastolic function were determined: peak velocities of the E and A waves (meters per second) and their ratios, the deceleration time of the E wave (milliseconds), and isovolumic relaxation time (milliseconds), measured as the time interval occurring between the end of systolic output flow and transmitral E-wave onset, by placing the pulsed Doppler sample volume between the outflow tract and the mitral valve. Transmitral inflow was analyzed also with the Valsalva maneuver to detect pseudonormal patterns. With pulsed tissue Doppler, peak early diastolic velocity on the septal part of the mitral annulus was measured (E′).
Two-Dimensional Strain Measurements
Using a dedicated software package (EchoPAC PC; GE Healthcare, Waukesha, WI), 2D strain was measured as previously described.
Two-dimensional strain is a novel, non-Doppler-based method to evaluate systolic strain from standard 2D acquisitions. By tracing the endocardial contour on an end-diastolic frame, the software automatically tracks the contour on subsequent frames. Adequate tracking can be verified in real time and corrected by adjusting the region of interest or manually correcting the contour to ensure optimal tracking. The sampling rate for 2D strain was 90 ± 15 frames/s.
For longitudinal strain, analysis was performed according to LV segments (6 basal, 6 mid, and 6 apical; Figure 1 ). For radial strain, analysis was performed according to LV segments from the basal, mid, and apical short-axis parasternal views.

Two-dimensional longitudinal and radial peak systolic strain were measured on 3 consecutive cycles by analyzing 2D acquisitions. Average values and standard deviations were calculated.
Reproducibility
The studies were analyzed offline by a second blinded observer for 30 patients, corresponding to 540 segments for longitudinal strain and 540 segments for radial strain.
Intraobserver variability was calculated by the average difference between the 30 measurements realized. Interobserver variability was calculated as the absolute difference divided by the average of the two observations for all parameters. Two-dimensional strain showed an intraobserver variability for peak systolic longitudinal strain of 3.6%, while interobserver variability was 5.2%.
TSI Study
Doppler tissue imaging was performed using apical views for the long-axis motion of the ventricles. Two-dimensional echocardiography with tissue Doppler color imaging views was optimized to reach the highest possible frame rate. At least 3 consecutive beats were stored, and the images were analyzed offline for TSI using a customized software package (EchoPAC PC). TSI is a parametric imaging tool derived from 2D Doppler tissue images. It automatically calculates and color codes the time to peak tissue velocity (Ts) in every position in the image with reference to the QRS signal ( Figure 1 ).
We studied the following parameters: the standard deviation of Ts of the 12 LV segments (Ts-SD-12-ejection) and the maximal difference in Ts between any 2 of the 6 basal LV segments (Ts-6-ejection). All the indices were automatically calculated by the dedicated software (EchoPAC PC) with the use of preset equations. The sampling rate for the TSI study was 100 ± 15 frames/s.
The reproducibility of TSI was calculated in 30 randomly selected data sets. The intraobserver and interobserver variability ranged from 7% to 9.5%.
Plasma N-Terminal Pro–Brain Natriuretic Peptide (NT-proBNP) Quantification
Immediately after their echocardiographic studies, patients with HCM had blood drawn. The samples were immersed in ice water and centrifuged within 1 hour for 15 minutes. Plasma was aspirated and placed in 1.5-mL Eppendorf tubes that were stored in a freezer. The tubes were sent in this condition to the laboratory, and blinded analyses were performed. NT-proBNP levels were measured by electrochemiluminescence sandwich immunoassay (Roche Diagnostics GmbH, Mannheim, Germany), and the results were reported as picograms per milliliter (detection interval, 5-70,000 pg/mL).
Ambulatory Holter Electrocardiography
Ambulatory Holter electrocardiographic recordings were obtained in a standard fashion using a portable tape recorder and modified V 1 and V 5 leads. Holter recordings were scanned on a DelMar Reynolds AccuPlus (model 403) Holter Analysis System (Del Mar Reynolds Medical, Irvine, CA). Arrhythmia frequency was normalized to 24 hours in recordings that did not include a complete 24-hour period of uninterrupted and interpretable rhythm because of noise or loss of signal. Three or more consecutive premature wide complexes with a heart rate > 100 beats/min were defined as NSVT.
Endpoints and Assessment
In the HCM outpatient clinic of Monaldi Hospital, ≥2 follow-up visit per year have been scheduled for each patient. Patients enrolled were asked to perform 24-hour Holter monitoring at 3-month intervals.
All the studied patients completed follow-up of 2 years. Patients were followed as outpatients at 3-month intervals, starting from the day of the echocardiographic evaluation. Follow-up data were obtained through reviews of patients’ hospital records, by periodic visits at our institution, or by phone interviews with the patients. Holter electrocardiography was performed every 3 months and when patients presented symptoms suggestive of arrhythmias. The primary endpoint was the occurrence of ventricular tachycardia. During the follow-up period, no patient of the studied cohort died.
Statistical Analyses
Data are expressed as mean ± SD. Clinical and demographic characteristics were compared using the Mann-Whitney U test for continuous variables and the χ 2 test for qualitative variables, expressed as proportions (or Fisher’s exact test for subgroups containing <5 observations). Predictors of NSVT were assessed using logistic regression analysis.
The ability of predictors to discriminate patients with NSVT on Holter monitoring was assessed using the area under the receiver operating characteristic curve (AUC) with 95% confidence interval. An AUC of 0.5 indicates that discrimination is no better than random, whereas an AUC of 1.0 indicates perfect discrimination. All comparisons were 2 tailed. A P value < .01 was considered statistically significant. StatView (SAS Institute Inc, Cary, NC) was used for all analyses.
Results
Patient Characteristics
Our study population consisted of 93 patients with HCM (mean age, 36 ± 17 years; mean body surface area, 1.8 ± 0.3 m 2 ; 71% men; Table 1 ). Twenty-four patients (26%) were in NYHA class II. Thirteen patients (14%) had histories of chest pain. Forty-eight patients (52%) had family histories of HCM, and 27 patients (29%) had family histories of sudden death. Of these, 11 were probands (index patients), and 11 were familial cases, with a rate of sudden death in index cases of 27.5%.
All patients with HCM | HCM without NSVT | HCM with NSVT | ||
---|---|---|---|---|
Variable | (n = 93) | (n = 69) | (n = 24) | P |
Age (y) | 36 ± 17 | 35 ± 16 | 39 ± 18 | NS |
Men (%) | 71 | 72 | 67 | NS |
Systolic blood pressure (mm Hg) | 119 ± 16 | 119 ± 16 | 119 ± 16 | NS |
Diastolic blood pressure (mm Hg) | 70 ± 12 | 70 ± 13 | 71 ± 12 | NS |
Body surface area (m 2 ) | 1.8 ± 0.3 | 1.8 ± 0.3 | 1.9 ± 0.2 | NS |
LV end-diastolic diameter (mm) | 46 ± 6 | 45 ± 6 | 47 ± 8 | NS |
LV ejection fraction (%) | 66 ± 8 | 64 ± 8 | 63 ± 6 | NS |
LV maximal wall thickness (mm) | 20 ± 5 | 19 ± 5 | 22 ± 6 | .03 |
LV mass index (g/m 2 ) | 128 ± 18 | 126 ± 16 | 125 ± 21 | NS |
Relative wall thickness | 0.78 ± 0.24 | 0.76 ± 0.26 | 0.79 ± 0.19 | NS |
E/A ratio | 1.2 ± 0.4 | 1.4 ± 0.5 | 1.6 ± 0.7 | NS |
Deceleration time (ms) | 207 ± 58 | 210 ± 62 | 197 ± 43 | NS |
E/Em ratio | 8.5 ± 2.5 | 8.6 ± 2.9 | 8.5 ± 2.9 | NS |
Obstructive HCM | 20% | 39% | 19% | NS |
NT-proBNP (pg/mL) | 669 ± 765 | 561 ± 593 | 1034 ± 1088 | .01 |
Risk factors for sudden death | ||||
1 | 30 | 20 | 10 | NS |
2 | 16 | 12 | 4 | NS |
≥3 | 8 | 5 | 3 | NS |
Seventy-five patients were under cardiac medical therapy at the time of the study. Of these, 48 patients were on β-blockers, 10 were on calcium channel blockers, 6 were on sotalol, 9 were on amiodarone, and 2 were on disopyramide. Prior implantable cardioverter-defibrillators were present in 8 patients.
Patients did not withdraw therapy before the echocardiographic evaluation, according to the rules of our institutional committee. Maximal LV wall thickness was 20 ± 5 mm (range, 13-40 mm), and 20 patients (22%) were defined as having obstructive HCM on the basis of the evidence of LV outflow tract gradients > 30 mm Hg under basal conditions or after the Valsalva maneuver (gradient > 30 mm Hg; range, 30-85 mm Hg; Table 1 ).
Myocardial hypertrophy involved the anterior IVS in 48 patients (52%), the anterior and posterior IVS in 22 patients (24%), the apex in 3 patients (3%), and both the IVS and the free wall in 20 patients (22%).
Ambulatory Holter Electrocardiography
Over 24 hours of Holter monitoring, 61 of the 93 study patients (66%) had ≥1 episode of ventricular arrhythmias, including 45 patients with premature ventricular contractions (range, 1-3600; mean, 160), 27 patients with couplets (range, 1 to 86; mean, 3.4), and 24 patients (26%) with runs of NSVT. The number of NSVT runs in 24 hours range from 1 to 8 (mean, 1.6), with 3 to 66 beats (in the longest burst) and at a mean ventricular rate of 136 ± 23 beats/min. Five patients had NSVT > 10 beats. Four patients with HCM had >1 Holter recording positive for NSVT.
Relation of Arrhythmias to Clinical and Standard Echocardiographic Characteristics
Patients with NSVT had higher values of maximal LV thickness than those without NSVT (22 ± 6 vs 19 ± 5 mm, P = .03).
The AUC, generated to assess the capability of maximal wall thickness (cutoff value > 20mm) to discriminate patients with and without NSVT, showed sensitivity of 57.1%, specificity of 65.7%, and an AUC of 0.634 ( Figure 2 ).
There were no statistically significant associations between NSVT on Holter electrocardiography and LV outflow gradient at rest or after the Valsalva maneuver, NYHA functional class, history of syncope, or the presence of medical therapy (19 of 24 patients vs 51 of 69 patients, P = .90). There was no significant relationship between NSVT and the presence of ST-segment depression. Family histories of sudden death were significantly more frequent in patients with HCM with NSVT (13 of 24) than in those without NSVT (14 of 69) ( P = .048).
NT-proBNP values were significantly ( P = .01) higher in patients with NSTV (1034 ± 1088 pg/ml) than in those without NSVT (561 ± 593 pg/ml). NT-proBNP showed sensitivity of 42.9% and specificity of 84.3%, with an AUC of 0.615 to predict NSVT in patients with HCM (cutoff value > 772; Figure 2 ). NT-proBNP was not significantly correlated with the number of risk factors for sudden death.
Relation of Arrhythmias to New Ultrasonic Parameters
The average analysis time per patient for the TSI indexes obtained from apical 4-chamber, 2-chamber, and 3-chamber views was 3 minutes. Patients with HCM and NSVT showed similar values of TSI parameters to those obtained in patients with HCM without NSVT (Ts-SD-12-ejection: patients with HCM and NSVT, 52 ± 26 vs 51 ± 19, P = .90; and Ts-6-ejection: patients with HCM and NSVT 48 ± 30 vs 70 ± 56 ms, P = .08; Figure 3 ). TSI indexes did not correlate with the number of risk factors for sudden death.
The average analysis time per patient for 2D strain was 5 minutes. Patients with HCM and NSVT showed similar average longitudinal strain than those without NSVT (−15.8 ± 2.4% vs −16 ± 3.5%, P = NS). However, in patients with HCM and NSVT, peak systolic strain was significantly reduced in the basal septum, the mid septum, and the basal anteroseptal wall ( Table 2 ).
HCM without NSVT | HCM with NSVT | ||
---|---|---|---|
Variable | (n = 69) | (n = 24) | P |
Longitudinal strain (%) | |||
Apical 4-chamber view | |||
Basal septum | −12 ± 4 | −10 ± 4 | .02 |
Mid septum | −14 ± 3 | −12 ± 4 | .01 |
Apical septum | −21 ± 5 | −20 ± 5 | NS |
Apical LW | −19 ± 7 | −20 ± 5 | NS |
Mid LW | −17 ± 5 | −15 ± 6 | NS |
Basal LW | −17 ± 6 | −16 ± 6 | NS |
Apical 3-chamber view | |||
Basal PLW | −16 ± 5 | −16 ± 5 | NS |
Mid PLW | −15 ± 4 | −15 ± 4 | NS |
Apical PLW | −20 ± 5 | −18 ± 5 | .09 |
Apical AS | −17 ± 6 | −17 ± 6 | NS |
Mid AS | −14 ± 3 | −14 ± 3 | NS |
Basal AS | −12 ± 4 | −11 ± 3 | NS |
Apical 2-chamber view | |||
Basal IW | −16 ± 5 | −15 ± 5 | NS |
Mid IW | −15 ± 4 | −14 ± 3 | NS |
Apical IW | −20 ± 5 | −18 ± 5 | .09 |
Apical AW | −18 ± 7 | −16 ± 5 | NS |
Mid AW | −17 ± 5 | −14 ± 5 | .07 |
Basal AW | −15 ± 5 | −15 ± 6 | NS |
TSI parameters | |||
Ts-SD-12-ejection | 51 ± 19 | 52 ± 26 | NS |
Ts-6-ejection | 70 ± 56 | 48 ± 30 | .08 |
The AUC, generated to assess the capability of longitudinal basal septal, mid septal, and basal anteroseptal wall strain, showed the best predictive value for mid septal strain (cutoff value > −15%; sensitivity, 81%; specificity, 54.4%; AUC, 0.680; Figure 4 ).
Patients with HCM and NSVT showed more LV segments with peak systolic strain ≥ −10% (3.9 ± 0.7 vs 2.2 ± 0.5, P < .0001; Figure 2 ). In particular, the presence of >3 segments with peak systolic strain ≥ −10% had sensitivity of 81%, specificity of 97.1%, and an AUC of 0.944 to detect patients with NSVT during follow-up ( Figure 5 ).
Average radial strain was similar between patients with HCM with and without NSVT. In those with NSVT, peak systolic radial strain was significantly reduced in the mid anterior, mid septal, and apical anteroseptal walls ( Table 3 ).
HCM without NSVT | HCM with NSVT | ||
---|---|---|---|
Variable | (n = 69) | (n = 24) | P |
Radial strain (%) | |||
Short-axis view, mitral valve level | |||
Anteroseptal wall | 27 ± 11 | 27 ± 10 | NS |
Anterior wall | 36 ± 14 | 34 ± 10 | NS |
Lateral wall | 40 ± 16 | 42 ± 13 | NS |
Posterior wall | 42 ± 16 | 45 ± 14 | NS |
Inferior wall | 36 ± 16 | 36 ± 15 | NS |
Septal wall | 29 ± 13 | 28 ± 14 | NS |
Short-axis view, papillary muscle level | |||
Anteroseptal wall | 36 ± 16 | 29 ± 15 | .07 |
Anterior wall | 40 ± 16 | 32 ± 14 | .04 |
Lateral wall | 40 ± 16 | 36 ± 13 | NS |
Posterior wall | 39 ± 17 | 36 ± 14 | NS |
Inferior wall | 38 ± 17 | 31 ± 14 | .08 |
Septal wall | 37 ± 17 | 26 ± 16 | .0097 |
Short-axis view, apical level | |||
Anteroseptal wall | 34 ± 16 | 26 ± 11 | .03 |
Anterior wall | 32 ± 16 | 27 ± 11 | NS |
Lateral wall | 31 ± 14 | 29 ± 12 | NS |
Posterior wall | 32 ± 14 | 31 ± 15 | NS |
Inferior wall | 37 ± 17 | 30 ± 14 | .09 |
Septal wall | 37 ± 20 | 28 ± 11 | .05 |
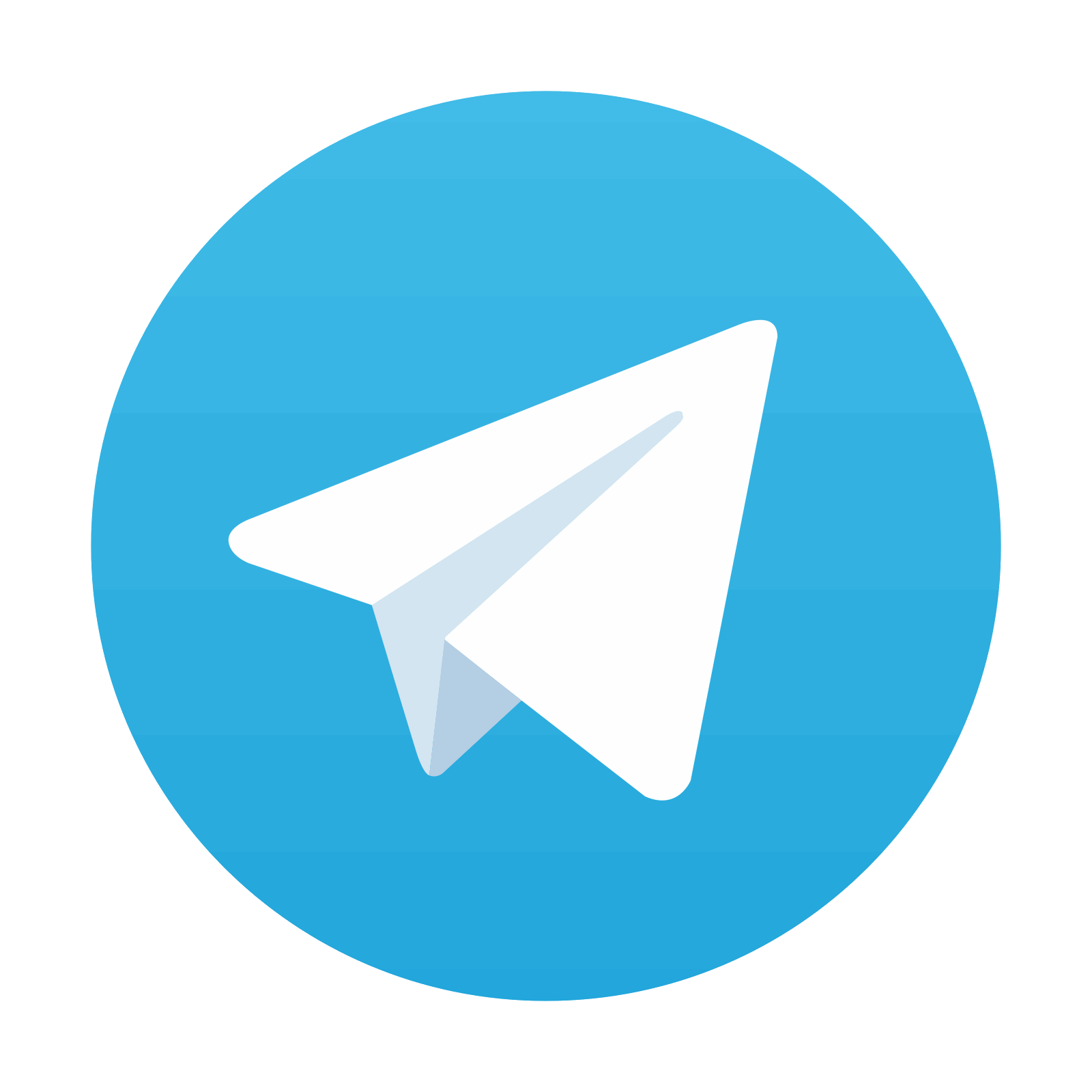
Stay updated, free articles. Join our Telegram channel

Full access? Get Clinical Tree
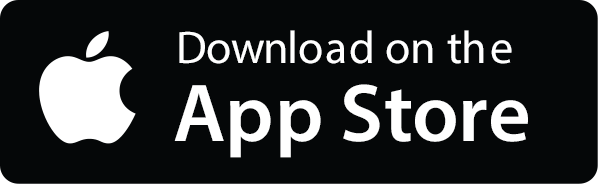
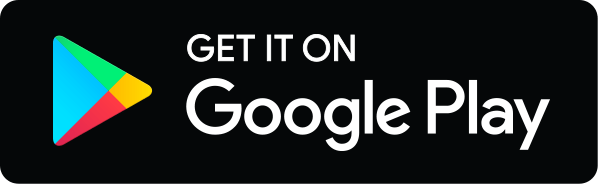
