Background
Right ventricular mechanics influence outcomes in patients with hypoplastic left heart syndrome (HLHS). The aim of this study was to determine whether differences in right ventricular performance have their origins in fetal life and if the architectural character of the hypoplastic left ventricle affects right ventricular mechanics.
Methods
The first complete fetal echocardiograms after 17 weeks’ gestation were reviewed in 84 fetuses with HLHS and in 115 gestational age-matched normal controls. Inflow, outflow, and myocardial tissue Doppler velocities were measured. E/A and E/e′ ratios and right ventricular myocardial performance index were calculated.
Results
In fetuses with HLHS, there were lower tricuspid E/A ratios (mean, 0.6 ± 0.1 vs 0.7 ± 0.1; P < .001), higher E/e′ ratios (mean, 8.1 ± 2.6 vs 7.0 ± 1.3; P = .006), and higher right ventricular myocardial performance indices (mean, 0.47 ± 0.14 vs 0.40 ± 0.10; P < .001) compared with controls. Among fetuses with HLHS grouped according to left ventricular architecture, those with left ventricular endocardial fibroelastosis had the most striking differences in right ventricular mechanics.
Conclusions
Right ventricular mechanics are different from normal in fetuses with HLHS and are influenced by the presence of left ventricular endocardial fibroelastosis. These differences precede the imposition of undue loading conditions as a consequence of surgical palliation and may offer clues to the development of later right ventricular failure.
Hypoplastic left heart syndrome (HLHS) includes cardiac defects with hypoplasia or atresia of the mitral valve and/or the aortic valve resulting in a hypoplastic left ventricle. HLHS variants include significant left ventricular (LV) hypoplasia in the setting of unbalanced atrioventricular canal defect and/or double-outlet right ventricle. All of these defects share a common consequence: the inability of the left ventricle to support the systemic circulation. Single-ventricle palliation is required, leaving the right ventricle as the systemic ventricle. Advances in surgical and medical management have led to improved survival of patients with HLHS. In the long-term, however, many of these children face a significant risk for heart failure. Abnormal right ventricular (RV) mechanics and myocardial dysfunction of the systemic right ventricle are important factors in the development of heart failure and influence long-term outcomes.
Abnormalities of RV mechanics in congenital heart disease in which the right ventricle is destined to be the systemic ventricle may present as early as fetal life. The fetal circulation provides a unique opportunity to study inherent, native ventricular mechanics before postnatal alterations in loading conditions as a result of the transition to the postnatal pulmonary circulation or surgical palliation. Thus far, the most common way to assess fetal RV function has been a qualitative evaluation of the degree of contraction. Ultrasound-derived Doppler tissue imaging (DTI) and myocardial performance index (MPI) can provide a quantitative assessment of RV systolic and diastolic performance, independent of morphology. These indices have been shown to be abnormal in the presence of some fetal abnormalities. However, little is known about RV mechanics in fetuses with HLHS and its variants.
The primary objective of this study was to determine whether, and to what degree, RV myocardial mechanics and performance are altered in fetuses with HLHS compared with fetuses with normal cardiac anatomy. The secondary objective was to determine if the architectural character of the abnormal left ventricle in HLHS influences RV myocardial mechanics and performance in the fetus.
Methods
This cross-sectional retrospective review used the echocardiographic imaging database of the Fetal Heart Program of the Cardiac Center at the Children’s Hospital of Philadelphia. Fetal echocardiograms from pregnant women referred for fetal echocardiography between January 2006 and December 2008 were reviewed. Echocardiograms demonstrating diagnoses of HLHS with severe mitral stenosis or atresia and/or severe aortic stenosis or atresia, double-outlet right ventricle, and unbalanced atrioventricular canal defect to the right ventricle were included. All fetuses had significant LV hypoplasia, left-to-right shunting at the atrial level, and retrograde perfusion of the transverse aorta from the ductus arteriosus. Common to all was the presence of a systemic right ventricle with varying degrees of LV nonviability. When serial echocardiograms from the same fetus were available, only the earliest, complete echocardiogram was included. Echocardiograms obtained when a fetus was <17 weeks or >41 weeks were excluded. To exclude confounding variables that may influence RV function, echocardiograms that demonstrated clear evidence of qualitatively decreased RV shortening on two-dimensional imaging, restriction across the atrial septum as indicated by dilated pulmonary veins and/or significant retrograde flow in the pulmonary veins on pulse Doppler tracing, greater than mild atrioventricular valve regurgitation, signs of fetal hydrops, or rhythm other than sinus were excluded. Echocardiograms with poor-quality images and those without the required inflow and outflow pulse Doppler tracings or DTI were also excluded.
A preexisting database of pulse and tissue Doppler measurements derived from normal fetal echocardiograms obtained at the Fetal Heart Program of Children’s Hospital of Philadelphia was used for the control group. Normal data for this database were obtained from fetal echocardiograms obtained for a family history of congenital heart disease. All fetuses had normal cardiovascular anatomy, normal biventricular size and qualitatively normal shortening, no extracardiac abnormalities, and normal uteroplacental function as defined by normal umbilical and uterine artery pulsatility indices for gestational age. All fetal echocardiograms from our normal database obtained at similar gestational ages as those from the subjects with HLHS were included. The measurements for both the study and control echocardiograms were collected offline by the same operators (M.M., D.S., and Z.T.). The institutional review board at The Children’s Hospital of Philadelphia approved this study (#2008-3-5836).
Echocardiographic Evaluation
A complete fetal echocardiographic study was performed using a Siemens Acuson Sequoia 256 system (Siemens Medical Solutions USA, Inc., Mountain View, CA) coupled with a 6C2 transducer. Pregnancy duration was estimated from the last menstrual period and confirmed by ultrasound measurement. Multiple two-dimensional views of the heart were obtained to evaluate two-dimensional fetal cardiac anatomy and qualitatively evaluate systolic shortening as previously described. All images were recorded digitally as clips or still frames and stored on our digital archiving system (KinetDx; Siemens Medical Solutions USA, Inc.).
Doppler interrogation of blood flow signals was performed at the tips of the tricuspid valve from an apical four-chamber view in diastole and at the distal RV outflow tract just below the level of the outflow valve in systole, as previously described. All Doppler recordings were obtained at an insonation angle <10° to flow. Angle correction was not used.
DTI signals were collected from the pulsed Doppler mode, as previously described. The Nyquist limit was decreased to detect the low myocardial tissue velocities. Filters were set to exclude high-frequency signals, and gains were minimized to allow a clear tissue signal with minimal background noise. The DTI signal was obtained from the apical four-chamber view. This sample was placed at the cardiac base just beneath the tricuspid valve annulus and signal obtained from the RV free wall using a 1-mm to 2-mm sample volume at a sweep speed of 100 mm/sec.
Doppler-Derived Flow Parameters
Measurements were performed offline using KinetDx cardiovascular imaging software. All measurements were recorded as averages of three cardiac cycles.
To assess diastolic function, inflow velocities and tissue velocities were measured and relevant ratios calculated. Tricuspid valve inflow peak E-wave (early diastole) and peak A-wave (late diastole) velocities were measured. The E/A ratio was calculated. RV free wall DTI peak s′ (systole), peak e′ (early diastole), and peak a′ (late diastole) velocities were measured. The s′ velocity is a measure of systolic performance. The E/e′ ratio was calculated; a higher E/e′ ratio corresponds to higher filling pressure.
The RV MPI, or Tei index, is a global measure of combined RV systolic and diastolic performance and is defined as the sum of the isovolumic contraction and relaxation times (isovolumetric time) divided by the ejection time, as previously described. The MPI was calculated using blood pool pulse Doppler signals, not tissue Doppler signals.
To evaluate interobserver variability of E/e′ and MPI, two independent observers (S.N. and A.S.) analyzed 20 randomly selected patients at different times. Each observer was blinded to the other’s measurements. To evaluate intraobserver variability, one observer (S.N.) remeasured 10 of the 20 randomly selected patients 4 weeks after the initial measurement for interobserver variability.
Statistical Analysis
Preliminary statistical analysis with Q-Q plots showed that the data were normally distributed. Therefore, data were analyzed using parametric analyses. Fetal gestational age, E/e′ ratio, and MPI were normally distributed for fetuses with HLHS and control subjects. Linear regression analysis was used to determine if there were any significant changes in the variables over gestational age. Because we found no significant changes in Doppler indices for either group over the course of gestation, these data are expressed as mean ± SD, and unpaired Student’s t tests were used for comparisons of means. All values were considered significantly different at P < .05. Interclass correlation coefficients were used to evaluate interobserver and intraobserver variability of the echocardiographic measurements. Interclass correlation coefficients of 0.7 to 0.8 indicate good agreement and coefficients > 0.8 strong agreement between measurements.
To determine if the architectural character of the abnormal left ventricle in HLHS influences RV myocardial mechanics and performance in the fetus, a subgroup analysis was performed. Echocardiograms in the HLHS group were stratified into the following groups: no discernible LV cavity (group 0), small LV cavity without endocardial fibroelastosis (EFE; group 1), and small LV cavity with EFE (group 2). Echocardiographically derived EFE is defined as the appearance of smooth-walled endocardium with eggshell-like echo brightness in the left ventricle compared with the signal intensity within the RV myocardium. The left ventricle may also have a spherical shape. Pulsed-wave Doppler and DTI ratios as well as RV MPI were compared among the three HLHS subgroups and the normal control group using analysis of variance with Bonferroni’s correction. Statistical analyses were performed using SPSS for Windows (SPSS, Inc., Chicago, IL).
Results
One hundred two consecutive fetuses with HLHS and HLHS variants presenting for fetal echocardiography between January 2006 and December 2008 were reviewed. Seven of these fetuses had more than mild atrioventricular valve regurgitation, six had restriction across the atrial septum, three had complete heart block, one had hydrops fetalis, and one did not have adequate imaging. All of these fetuses were excluded from analysis. Eighty-four fetuses fulfilled our inclusion criteria, and the first complete echocardiogram after 17 weeks’ gestation was included in the analysis. One hundred fifteen normal echocardiograms constituted the control group. There were no significant differences in the gestational ages of the study group compared with the control group ( Table 1 ). By linear regression analysis, blood pool E (HLHS, R 2 = 0.21, P < .001; normal, R 2 = 0.32, P < .001) and A (HLHS, R 2 = 0.14, P < .05; normal, R 2 = 0.13, P < .001) velocities and tissue Doppler s′ (HLHS, R 2 = 0.17, P < .05; normal, R 2 = 0.39, P < .001), e′ (HLHS, R 2 = 0.13, P < .05; normal, R 2 = 0.56, P < .001), and a′ (HLHS, R 2 = 0.13, P < .005; normal, R 2 = 0.14, P < .001) velocities increased over gestational age for both the HLHS and control subjects. There were no significant changes over gestational age for RV E/e′ ratio or RV MPI in both the study and control groups ( Figure 1 ).
Variable | HLHS group | Control group | P |
---|---|---|---|
Gestational age (wk) | 26.8 ± 5.2 (84) | 25.8 ± 4.6 (115) | .13 |
Tricuspid valve | |||
E (cm/sec) | 38.1 ± 10.0 (72) | 38.0 ± 7.2 (115) | .90 |
A (cm/sec) | 60.7 ± 12.8 (80) | 55.6 ± 8.8 (115) | .002 ∗ |
E/A ratio | 0.6 ± 0.1 (64) | 0.7 ± 0.1 (115) | <.001 ∗ |
RV free wall DTI | |||
s′ (cm/sec) | 4.6 ± 1.1 (61) | 5.3 ± 0.8 (115) | <.001 ∗ |
e′ (cm/sec) | 5.1± 1.6 (58) | 5.5 ± 1.0 (115) | .02 ∗ |
a′ (cm/sec) | 9.2 ± 2.2 (61) | 9.0 ± 1.7 (115) | .50 |
RV E/e′ ratio | 8.1 ± 2.6 (52) | 7.0 ± 1.3 (115) | .006 ∗ |
RV MPI | 0.47 ± 0.14 (81) | 0.40 ± 0.10 (111) | <.001 ∗ |
Echocardiographic measurements for both groups are noted in Table 1 . For the pulsed-wave Doppler blood pool velocities across the tricuspid valve, the peak A-wave velocity was significantly higher and the E/A ratio was significantly lower in the HLHS group compared with the control group. For the DTI tissue velocities of the RV free wall, the peak s′ and e′ velocities were significantly lower in the HLHS group compared with the control group. Both the E/e′ ratio and RV MPI were significantly higher in the HLHS group compared with the control group.
Subgroup analysis was performed to compare measures of RV mechanics among fetuses with variable LV architecture ( Table 2 , Figure 2 ). RV E/e′ ratio and MPI were higher in each of the HLHS subgroups compared with normal control subjects. Compared to normal controls, the subgroup with small LV cavities and EFE (group 2) distinguished itself as having significantly higher tricuspid valve A velocities, lower tricuspid valve E/A ratios, lower RV e′ velocities, lower s′ velocities, higher RV E/e′ ratios, and higher RV MPIs. Group 2 had significantly higher E/e′ ratios than group 1.
Variable | Group 0 ( n = 29) | Group 1 ( n = 21) | Group 2 ( n = 34) | Normal controls ( n = 115) | P |
---|---|---|---|---|---|
Tricuspid valve | |||||
A (cm/sec) | 59.4 ± 12.0 (26) | 56.6 ± 15.6 (21) | 64.3 ± 10.6 (33) ∗ | 55.6 ± 8.8 (115) ∗ | <.001 |
E/A ratio | 0.64 ± 0.1 (20) | 0.66 ± 0.1 (18) | 0.61 ± 0.1 (26) ∗ | 0.68 ± 0.1 (115) ∗ | <.001 |
RV free wall DTI | |||||
s′ (cm/sec) | 4.7 ± 1.2 (21) | 4.8 ± 1.0 (15) | 4.4 ± 1.0 (25) ∗ | 5.3 ± 0.8 (115) ∗ | <.001 |
e′ (cm/sec) | 5.2 ± 1.9 (19) | 5.5 ± 1.3 (15) | 4.7 ± 1.4 (24) ∗ | 5.5 ± 1.0 (115) ∗ | .02 |
RV E/e′ ratio | 7.76 ± 2.5 (16) | 7.21 ± 2.6 (14) † | 8.87 ± 2.6 (22) ∗† | 6.99 ± 1.3 (115) ∗ | <.001 |
RV MPI | 0.46 ± 0.15 (28) | 0.44 ± 0.13 (20) | 0.50 ± 0.13 (33) ∗ | 0.40 ± 0.10 (111) ∗ | <.001 |
∗ P < .05, group 2 versus normal controls.
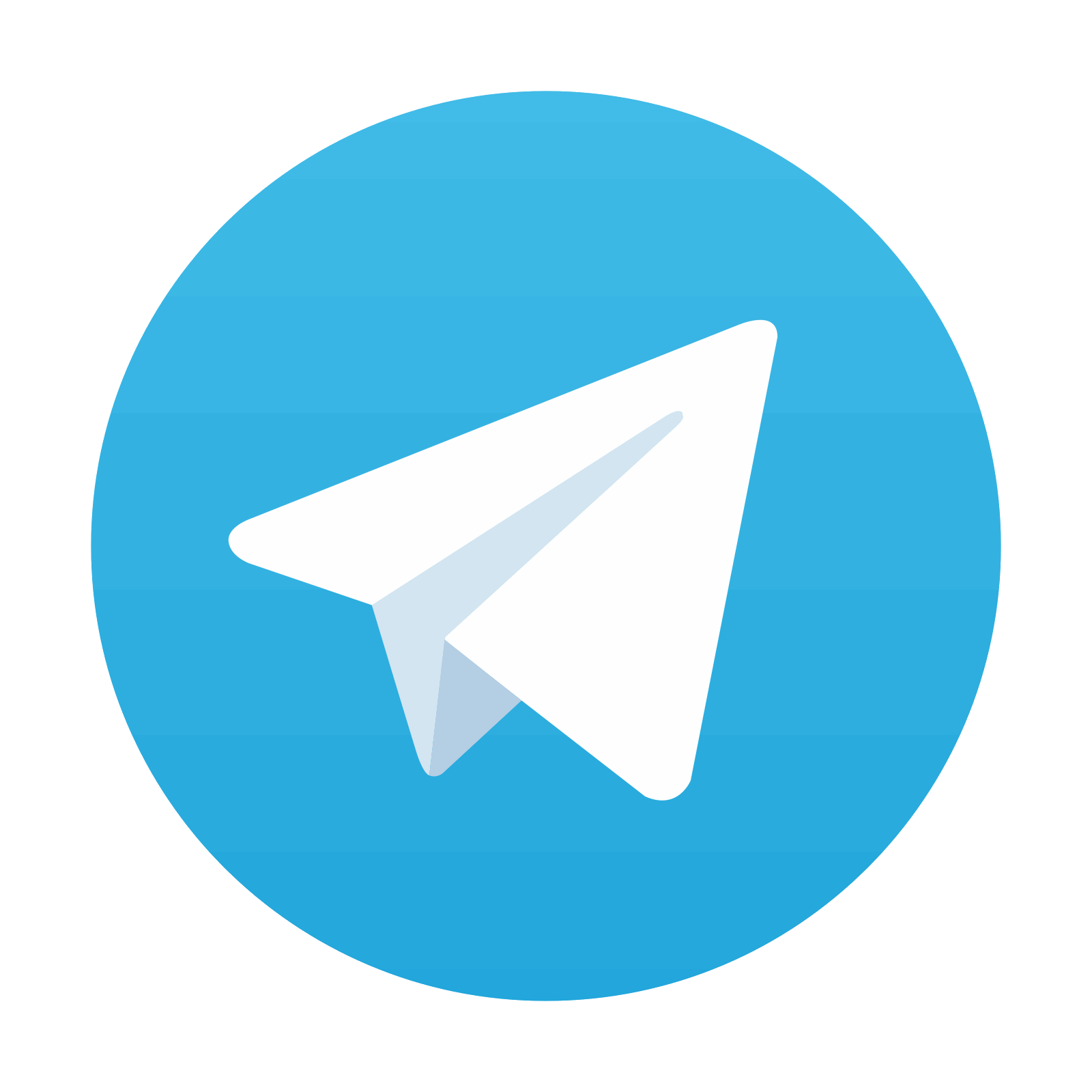
Stay updated, free articles. Join our Telegram channel

Full access? Get Clinical Tree
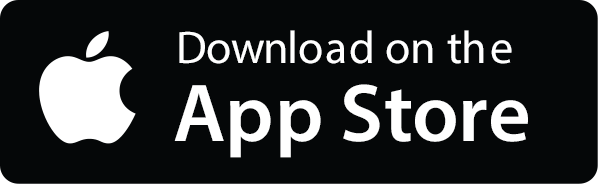
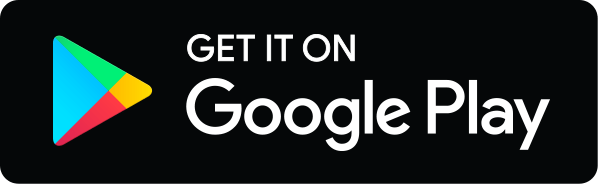