Restrictive Cardiomyopathy
Susan W. Denfield
Cardiomyopathies are defined as diseases of the myocardium associated with cardiac dysfunction (1). As per the World Health Organization (WHO), restrictive cardiomyopathy (RCM) is characterized by restrictive filling and reduced diastolic volume of either or both ventricles with normal or near-normal systolic function and wall thicknesses. Increased interstitial fibrosis may be present. It may be idiopathic or associated with another disease.
“Contemporary Definitions and Classification of the Cardiomyopathies” have been proposed by a consensus panel organized by the American Heart Association (AHA) to draw attention to the rapid advancement of molecular genetics in cardiology (2). The AHA consensus statement defined primary RCM as a rare form of heart disease characterized by “normal or decreased volume of both ventricles associated with biatrial enlargement, normal left ventricular wall thickness and atrioventricular valves, impaired ventricular filling with restrictive physiology, and normal (or near-normal) systolic function” (2). At that time the panel placed RCM in a “mixed” cardiomyopathy category because the causes of the diseases resulting in RCM were believed to be predominantly nongenetic.
This chapter reviews the epidemiology, etiologies including known genes and associated syndromes, pathology, pathophysiology, clinical presentation, diagnostic evaluation, differential diagnosis, outcome and management of pediatric patients with RCM.
Epidemiology
Of the four types of cardiomyopathy categorized by the WHO, dilated, hypertrophic, restrictive, and arrhythmogenic right ventricular cardiomyopathy, RCM is the least common (1). In children, RCM accounts for 2.5% to 5% of the diagnosed cardiomyopathies (3,4,5,6,7). In the Australian based study by Nugent et al., RCM accounted for 2.5% of the cardiomyopathies diagnosed in children less than 10 years of age (6). This is similar to the two region United States based study by Lipshultz et al., who reported that RCM or other specified types (not dilated or hypertrophic) accounted for 3% of the cardiomyopathies in children less than 18 years of age (7). Three different single institution studies reported 5%, which may have been due to greater referral bias (3,4,5).
Overall the incidence of cardiomyopathies is higher in children less than 1 year of age when all types of cardiomyopathy are considered (6,7). Although, RCM may be an exception. In the Nugent et al. study, the incidence of all types of cardiomyopathy except RCM declined rapidly after infancy to their maximum study age of 10 years (6). In the studies of RCM in children the average age at the time of diagnosis was 6 years with median of 5 years and a range from 0.1 to 19 years (3,4,5,8,9,10,11,12,13,14,15,16,17,18,19,20,21,22,23,24,25,26,27,28,29,30,31,32,33). Only two of the patients were 19 at the time of diagnosis with the remainder less than 18 years old. At the time of diagnosis 8% were 1 year of age or less, with 12% between the ages of 12 and 19 years. Whether this apparent difference in usual age of onset is due to case ascertainment or true differences in the disease onset is unknown.
Gender differences have also been reported in cardiomyopathies. Beyond infancy boys had a higher incidence of cardiomyopathy than girls in the Lipshultz et al. study (7). Only hypertrophic and unspecified cardiomyopathies occurred with higher frequency in boys in the Nugent et al. study with RCM occurring with equal frequency in boys and girls (31). Of the 205 subjects with RCM in whom gender was reported 54% were female (3,4,5,6,7,8,9,10,11,12,13,14,15,16,17,18,19,20,21,22,23,24,25,26,27,28,29,30,31,32,33,34,35,36,37).
Genetics
Mutations in at least 10 genes have been associated with the RCM phenotype and include sarcomeric and nonsarcomeric gene defects (Table 56.1) (38,39,40,41,42,43,44,45,46,47,48,49,50,51,52,53). Modes of inheritance include autosomal dominant, autosomal recessive, and X-linked. De novo mutations also occur.
Sarcomeric Protein Gene Defects
Troponin I mutations have been identified in patients with RCM and hypertrophic cardiomyopathy (HCM) (39). Both the RCM phenotype and the HCM phenotype can be expressed in the same family. This indicates that idiopathic RCM can be part of the clinical expression of sarcomeric contractile protein disease. The diversity of the phenotypic expression of troponin mutations in families suggests that additional genetic or environmental factors or both play a role in disease expression. De novo mutations and autosomal dominant transmission have been documented in RCM patients and their families (39).
Kaski et al. reported a small series of 12 pediatric patients with RCM; 4 had a positive family history of cardiomyopathy, but the phenotypes varied and included restrictive, dilated, and left ventricular noncompaction forms (44). Gene mutations were found in
troponin I (TNNI3), troponin T (TNNT2), and alpha cardiac actin (ACTC). Peddy et al. reported an infant who presented with malignant arrhythmias and RCM and had a de novo mutation of cardiac troponin T (45).
troponin I (TNNI3), troponin T (TNNT2), and alpha cardiac actin (ACTC). Peddy et al. reported an infant who presented with malignant arrhythmias and RCM and had a de novo mutation of cardiac troponin T (45).
TABLE 56.1 Gene Mutations Reported in Association with RCM | ||||||||||||||||
---|---|---|---|---|---|---|---|---|---|---|---|---|---|---|---|---|
|
Ware et al. described a mutation in a beta-myosin heavy chain gene in an infant with RCM (46). Mutations in the beta-myosin heavy chain account for approximately 40% of mutations found in HCM in adults (47). The overlapping genotypic–phenotypic correlations are also evident in a report by Olson et al. (48). The proband was a child with a myosin light chain mutation resulting in a cardiomyopathy with midcavitary hypertrophy and restrictive physiology that was inherited in an autosomal recessive pattern. His two older brothers had cardiomyopathy with dilated atria, both of whom died related to thrombotic complications. Clinically unaffected family members were either heterozygotes or lacked the mutant allele.
Titin is a giant protein that plays a fundamental role in sarcomere structure and regulation of resting tension. Peled et al., reported a titin mutation in a family with RCM found in two generations (52). Additional gene mutations are likely to be found with ongoing genetic studies.
Nonsarcomeric Protein Gene Defects
Desmin, BAG3, and RSK2 mutations have been reported in children with RCM, while lamin A/C and transthyretin mutations have been reported to cause an RCM phenotype in adults. A Danish kindred has been documented in whom children have been identified as carriers of the familial transthyretin methionine 111 mutation, but none of the kindred has manifested amyloid-associated RCM in childhood (49), although adults in the family have developed RCM. The transthyretin ile 122 mutation resulting in RCM with amyloidosis has only been reported to manifest RCM in adulthood, as well (40).
Desmin is a myofibrillar protein that is the chief intermediate filament of skeletal and cardiac muscle (41). It maintains the structural and functional integrity of the myofibrils and functions as a cytoskeletal protein-linking Z bands to the plasma membrane. Mutations in the desmin gene may cause RCM with or without skeletal myopathy and with or without conduction system disease (41,42,50,51). The inheritance pattern is usually autosomal dominant. Sporadic mutations have also been identified (41).
BAG3 is a Z-disc–associated protein with antiapoptotic properties. Mutations in this gene are associated with progressive limb and axial muscle weakness, cardiomyopathy including restrictive, severe respiratory insufficiency, rigid spines, and peripheral neuropathy (53).
Coffin–Lowry syndrome is an X-linked disorder caused by mutations in the RSK2 gene on chromosome Xp22.2 (43). It is characterized by developmental delays, short stature, facial dysmorphisms, and progressive skeletal deformities. Cardiac anomalies are reported in approximately 14% of affected males with cardiomyopathy being one of the rare but reported cardiac abnormalities, including one patient who had a restrictive phenotype (33).
Emery–Dreifuss muscular dystrophy was first described as an X-linked disorder caused by mutations in the gene encoding for emerin on chromosome Xq28 (38). However, it can also be an autosomal disorder caused by mutations in the gene encoding for lamin A and lamin C (LMNA) on chromosome 1q21.2-q21.3 (38). Both variants of the disease can cause cardiac abnormalities including dilated cardiomyopathy, atrial and ventricular arrhythmias, conduction abnormalities, and sudden death. Sanna et al., reported on the cardiac features of Emery–Dreifuss caused by lamin A/C gene mutations, including one adult patient with a restrictive phenotype (38). To date the RCM phenotype has not been reported in children with Emery–Dreifuss.
Alström syndrome is characterized by cone–rod dystrophy resulting in progressive visual impairment, photophobia and nystagmus, obesity, progressive sensorineural hearing impairment, dilated or restrictive cardiomyopathy, insulin resistance, and multiple organ failure (54). ALMS1 is the only gene currently known to be associated with the syndrome and is estimated to be detected in 25% to 40% of individuals.
Mulibrey nanism is an autosomal recessive disease caused by mutations in the TRIM37 gene on chromosome 17q22-q23 encoding the peroxisomal TRIM37 protein of unknown function (55). Congestive heart failure and pericardial constriction were diagnosed during infancy in 12% and 6% of the patients respectively in a report by Karlberg et al. (55). Characteristic craniofacial features included scaphocephaly, facial triangularity, high and broad forehead, and low nasal bridge in over 90% of the patients. Other findings included a peculiar high-pitched voice (96%), yellowish dots in ocular fundi (79%), cutaneous naevi flammei (65%), hepatomegaly (45%), and fibrous dysplasia of long bones (25%). Mild muscular hypotonicity (68%) was the only neurologic abnormality. Although more typically associated with constrictive pericarditis (CP), a mixed constrictive/restrictive phenotype has been described as not all patients have improved with pericardiectomy and myocardial hypertrophy and fibrosis have been reported (56,57).
Etiologies
RCM has multiple causes and may result from myocardial diseases including noninfiltrative or infiltrative processes, storage diseases, endomyocardial diseases, myocarditis, and following cardiac transplantation (58) (Table 56.2). The pathology and histology vary with the underlying disease process. The most common etiology of RCM worldwide is secondary to endomyocardial fibrosis (EMF). EMF is estimated to affect 10 million people worldwide, occurring most often in children and adolescents (59,60). In adults, outside the tropics, amyloidosis is the most common cause of RCM (61). One case of cardiac amyloidosis has been reported in a pediatric patient (5). In the pediatric population, outside the tropics, idiopathic RCM is probably the most common cause of RCM based on reported cases in the literature (3,4,5,6,7,8,9,10,11,12,13,14,15,16,17,18,19,20,21,22,23,24,25,26,27,28,29,30,31,32,33,34,35,36,37).
Endomyocardial Disease
EMF was first described by Davies in 1948 (62). It occurs most frequently in tropical and subtropical Africa, particularly Uganda and Nigeria. However, it is found in tropical and subtropical regions throughout the world. It is occasionally seen in temperate climates, usually in individuals who previously lived in tropical areas. The etiology or etiologies remain unknown. Hypereosinophilia, likely related to parasitic infections, has occurred in some patients. However, there does not appear to be an increased prevalence of parasitic infections in patients with EMF. Autoimmunity, genetic, dietary, and environmental chemical factors have all been implicated in the development of EMF (60).
The disease occurs most often in children to young adults (59,60,63). Familial occurrence, and in some countries a high incidence in some ethnic groups, suggests a possible genetic predisposition (59,60). In a rural area of Mozambique, the overall prevalence of EMF was 20% in the population, but increased to 28% when two family members were affected and to 39% when three or more family members were affected (59).
The disease is most commonly biventricular, followed by pure left ventricular involvement approximately 40% of the time and purely right ventricular in 10% (64). The symptom complex varies with the site or sites of involvement. Symptoms from pulmonary venous congestion result from left-sided disease, while right-sided
disease results in signs and symptoms from systemic venous congestion. Involvement of the mitral or tricuspid valve apparatus can result in significant valvular regurgitation. Medical and surgical therapy is palliative.
disease results in signs and symptoms from systemic venous congestion. Involvement of the mitral or tricuspid valve apparatus can result in significant valvular regurgitation. Medical and surgical therapy is palliative.
TABLE 56.2 Causes of Restrictive Cardiomyopathies in Children and/or Adults | ||||||||||||||||||||||||||||||||||||||
---|---|---|---|---|---|---|---|---|---|---|---|---|---|---|---|---|---|---|---|---|---|---|---|---|---|---|---|---|---|---|---|---|---|---|---|---|---|---|
|
The histology of EMF is characterized by fibrosis of the endocardium of variable thickness. Histologic changes occur in predominantly three areas: the left ventricular apex, the mitral valve apparatus, and the right ventricular apex which may extend to the supporting structures of the tricuspid valve. In severe cases the process may extend to the outflow tracts. Small patches of fibroelastosis may occur in the outflow tracts, but the elastin component is thought to be secondary and not a primary part of the process. Cellular infiltrates are not prominent. Eosinophilia is not typically a prominent feature in contrast to Löffler endocarditis.
Hypereosinophilic Syndrome/Löffler Endocarditis
Löffler endocarditis or the hypereosinophilic syndrome (HES) is similar to EMF in many respects. There is continued debate whether they are variants of the same disease. Although there are pathologic and clinical similarities, there are important contrasts. HES is typically seen in temperate climates. It is more common in adult males. Hypereosinophilia is present. HES includes persistent eosinophilia with 1,500 eosinophils/mm3 for at least 6 months or until death with evidence of organ involvement. Usually, in HES, other organs besides the heart are involved and may include the lungs, bone marrow, and brain (65). The cause of the eosinophilia is usually unknown, but may be leukemic or secondary to parasitic, allergic, granulomatous, hypersensitivity, or neoplastic disorders (66).
Cardiac histologic findings include variable degrees of eosinophilic myocarditis (not characteristically seen in EMF), inflammatory reaction in the small intramural coronary vessels with thrombosis and fibrinoid change, and endocardial mural thrombosis, and fibrotic thickening (64).
The clinical picture may include weight loss, fever, cough, rash, and heart failure. Systemic embolism is frequent. Death is usually secondary to the cardiac manifestations of the disease. Therapy for the hypereosinophilia may include corticosteroids, hydroxyurea, or vincristine, but this area of therapy is usually not directed by the cardiologist. Cardiac therapy has included digoxin, diuretics, afterload reduction, and anticoagulation. Surgical approaches have included mitral and/or tricuspid valve repair or replacement and excision of fibrotic endocardium and may be useful for symptom palliation of intractable heart failure. However, pediatric case reports and case series of HES and EMF patients report a relatively high reoperation rate secondary to dysfunction of the mechanical or bioprosthetic valves with recurrent thrombosis, fibrosis, or tearing despite anticoagulation (67,68). Significant cardiac symptoms may persist (class II to IV) (68).
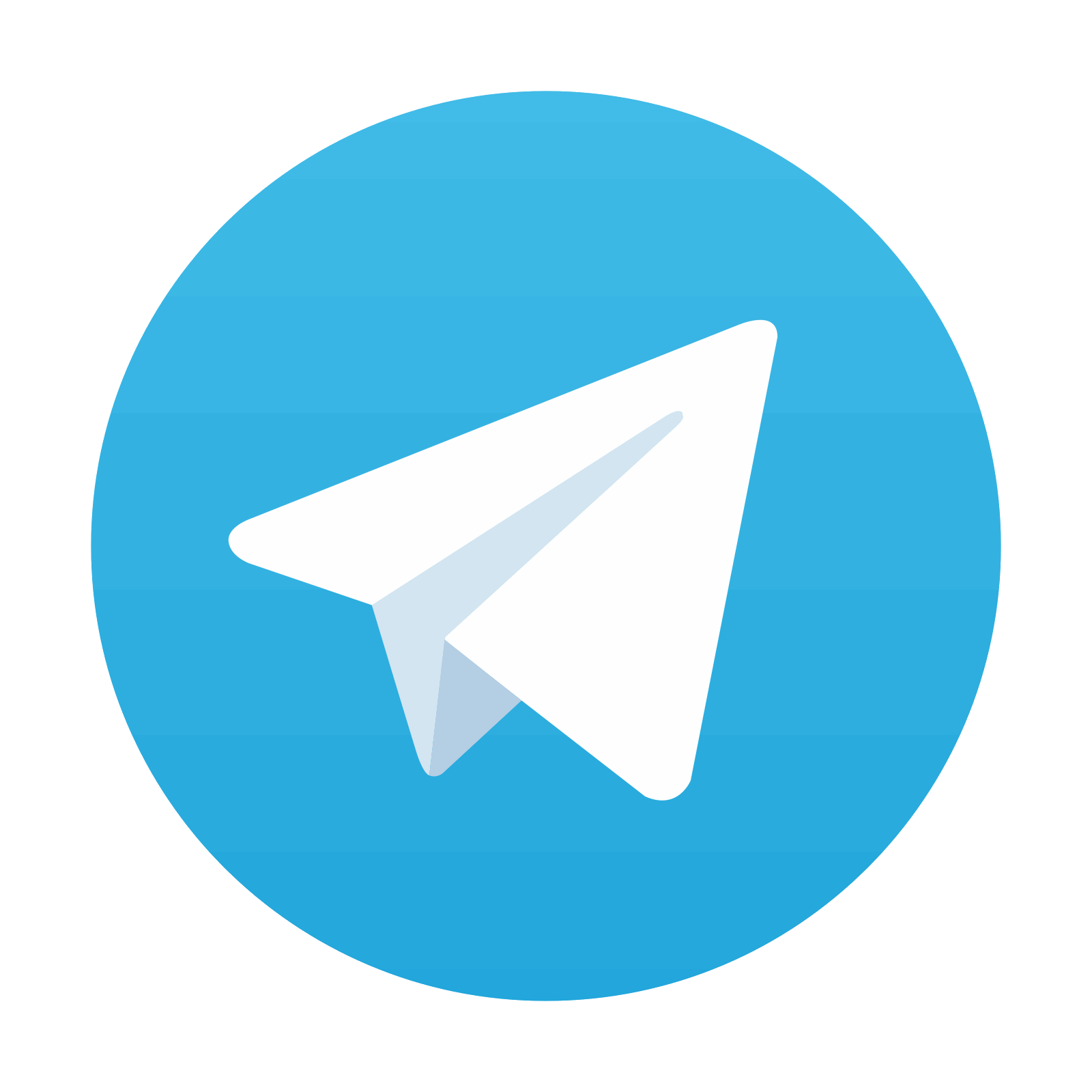
Stay updated, free articles. Join our Telegram channel

Full access? Get Clinical Tree
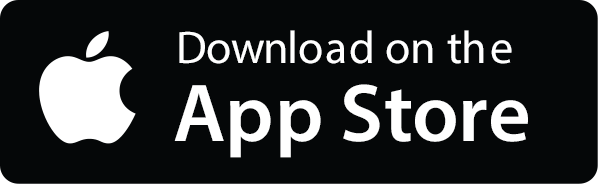
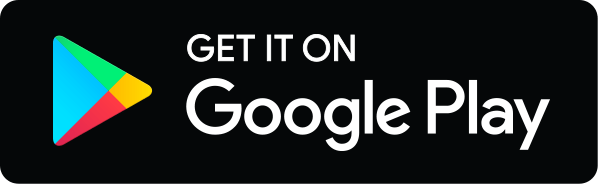