Background
Three-dimensional echocardiographic (3DE) analysis provides better measurements of left ventricular (LV) volumes, ejection fraction, myocardial deformation, and dyssynchrony. Many studies have shown that this technique has high intrainstitutional reproducibility. However, interinstitutional reproducibility is low, limiting its adoption. The aim of this study was to determine if standardization of training could reduce the interinstitutional variability in 3DE data analysis.
Methods
In total, 50 full-volume, transthoracic 3DE data sets of the left ventricle were analyzed by two readers. Measurements obtained included LV volumes, ejection fraction, global longitudinal strain, and two dyssynchrony indices. The cases represented a wide spectrum of ejection fraction. After initial analysis of 21 studies, readers formally met to standardize their analytic approach on six additional cases. Five months after the intervention, 23 new cases were analyzed. Paired t tests were performed to identify systematic institutional differences in measurements. Interinstitutional variability was quantified using intraclass correlation coefficients and variability.
Results
Before the intervention, there was a systematic bias in LV volumes, which was eliminated after intervention. Intraclass correlation coefficients showed that the intervention improved agreement in measurements of LV volumes, strain, and dyssynchrony between the two centers and decreased variability.
Conclusions
A simple intervention to standardize analysis can reduce interinstitutional variability of measurements obtained from 3DE analysis. This intervention is needed before the use of 3DE measurement in multicenter trials and to increase the reproducibility of such measurements in routine clinical practice.
Many studies have demonstrated that the use of three-dimensional (3D) echocardiographic (3DE) imaging for the assessment of left ventricular (LV) volumes, ejection fraction (EF), myocardial deformation, and dyssynchrony is superior to measurements obtained from two-dimensional echocardiographic (2DE) imaging. For LV volumes and EF, this improvement is due to circumventing the geometric assumptions and image foreshortening that occur with 2DE imaging. Likewise, for LV myocardial deformation measured using speckle-tracking (ST), the use of 3DE imaging improves measurements because speckles can be tracked frame to frame, as long as they remain within the acquisition pyramid. This is in contrast to 2DE ST, in which assessment is limited once the tracked speckles move out of the imaging plane. Similarly, assessment of LV dyssynchrony is improved with 3DE data because all of the walls of the left ventricle can be assessed at the same time, and as such, the LV segments that contract late (dyssynchronous) relative to the other LV segments can be identified.
Yet despite these advantages, widespread adoption of these methodologies into the clinical realm has not occurred. One reason for this discrepancy is that although reproducibility with 3DE imaging is superior 2DE analysis, overall interinstitutional reproducibility of 3DE measurements is poor. Most studies supporting the use of 3DE imaging in LV assessment were single-center studies in which data were acquired and measured by highly trained personnel. The use of trained personnel is crucial, because experience has been demonstrated to have an important role in achieving accurate, reproducible 3DE measurements, allowing better reproducibility than 2DE imaging. In contrast, meta-analyses and multicenter trials have demonstrated poor interinstitutional reproducibility and lower accuracy due to differences in acquisition and analysis techniques. The aim of this study was to perform a study to examine interinstitutional variability in the measurements of LV EF and volumes, LV mechanics, and LV dyssynchrony before and after the implementation of a strategy to increase reproducibility.
Methods
Study Design
In total, we studied 3DE LV data sets from 50 patients with a wide range of LV systolic function as determined by 2DE and 3DE image quality that was visually judged as adequate for ST analysis. In total, King’s College Hospital provided 24 3DE data sets, and the University of Chicago Medical Center provided 26. Three-dimensional echocardiographic images were defined as adequate if no more than two LV segments in a 16-segment LV model demonstrated poor endocardial definition. First, 21 data sets were independently analyzed by two readers (one from each institution) as per their institutional conventions. After analysis of these preintervention results, an in-person meeting was arranged, at which standardization of 3DE ST analysis with respect to placement of the endocardial contours, reference frame choice, and image loop choice occurred. As well, six new cases were analyzed during this meeting until both readers achieved similar results. Five months after this meeting, the two readers independently analyzed 23 new cases. The institutional review boards of both institutions approved the acquisition and analysis of 3DE data sets for this study.
Imaging
Three-dimensional echocardiographic imaging was performed using an iE33 scanner with an x5 matrix-array transducer (Philips Medical Systems, Andover, MA). Wide-angle, multiple-beat mode was used, in which four wedge-shaped subvolumes were obtained over four consecutive cardiac cycles during a single breath-hold. Special care was taken to include the entire LV cavity within the pyramidal scan volume. After gain settings were optimized for endocardial visualization using each imaging system, three or four data sets were acquired. Data sets were stored digitally for offline analysis.
Image Analysis
Images were analyzed using 4D LV Analysis software (TomTec Imaging Systems GmbH, Unterschleissheim, Germany) by two experienced readers. The same software version was used at both sites for analysis. This software was based on ST technology rather than the edge detection methodology. ST allows more precise measurements of volume and strain than edge detection as it tracks specific speckles rather than the black-white difference at the LV cavity–myocardial border. Measurements were performed using the data set with the best image quality, which was selected by consensus of the two readers. Apical two-chamber, three-chamber, and four-chamber views were automatically selected at end-diastole ( Figure 1 ). Once the user confirmed that the automatically selected views and frames were correct, the program automatically identified the LV endocardial boundary at end-diastole. After this step, the reader could optimize the boundary, and then the program would automatically construct a 3D LV endocardial shell and track the border in 3D space throughout the cardiac cycle. Subsequently, the endocardial surface was manually adjusted, when necessary, until a best match with the actual endocardial position was visually verified in the different views.

The left ventricle was automatically divided into 16 3D segments using standard segmentation. The following LV parameters were evaluated: end-diastolic volume (EDV), end-systolic volume (ESV), EF, stroke volume, global longitudinal strain (GLS), and two dyssynchrony indices, SDI and SDII. LV dyssynchrony can be measured by determining the time at which each LV segment reaches its minimal volume. SDI, the dyssynchrony index, is derived from the standard deviation of regional end-systolic times for the 16-segment model. Recently, a new dyssynchrony index, SDII, was derived. It is calculated as the standard deviation of shifts of the regional 3D displacement curves in relation to a mean curve for the 16-segment model. The shift comparison is performed using phase correlation and relates to the heart cycle length. Overall, SDII is expected to correct for LV apical rocking.
Statistical Analysis
To identify systematic differences in measurements between the two institutions, paired t tests were performed for each parameter before and after the intervention. To examine variability in measurements between the two institutions intraclass correlation coefficients and variability were calculated for each parameter before and after the intervention. Intraclass correlation coefficients are similar to conventional Pearson’s correlation coefficients but take into account the bias, and they are used to assess concordance between continuous variables. Variability was calculated as the percentage of the absolute difference between readers divided by the mean. All analyses were performed using Stata version 12.1 (StataCorp LP, College Station, TX).
Results
For all study patients, the average age was 55 ± 19 years, and 31 (62%) were men. The mean LV EF was 39 ± 16% (range, 9%–63%). Among the 50 data sets studied, the mean frame rate was 20 ± 4 Hz. Nine patients had left bundle branch block.
First we examined the impact of the standardization training on measurements of LV volumes and EF. Before the standardization training, there were systematic differences in LV EDV, ESV, stroke volume, and EF ( Table 1 ). After training, the difference was eliminated for all these measurements. Also, correlations of LV EDV and ESV measurements between the two centers increased, and variability decreased. For LV stroke volume, the correlation remained unchanged, but there was a slight increase in variability. For LV EF, there was a large increase in correlation and variability.
Variable | Preintervention cohort | Postintervention cohort | ||||||
---|---|---|---|---|---|---|---|---|
Measurements | Interinstitutional variability | Measurements | Interinstitutional variability | |||||
Institution 1 | Institution 2 | ICC | Variability (%) | Institution 1 | Institution 2 | ICC | Variability (%) | |
LV volumes and EF | ||||||||
EDV (mL) | 121 ± 31 | 139 ± 38 ∗ | 0.75 | 15.0 | 184 ± 88 | 191 ± 83 | 0.94 | 13.6 |
ESV (mL) | 60 ± 18 | 73 ± 25 ∗ | 0.69 | 19.5 | 141 ± 91 | 145 ± 84 | 0.96 | 15.9 |
Stroke volume (mL) | 62 ± 15 | 66 ± 16 ∗ | 0.86 | 9.9 | 43 ± 17 | 45 ± 17 | 0.87 | 12.1 |
EF (%) | 51 ± 6 | 48 ± 6 ∗ | 0.79 | 6.5 | 28 ± 16 | 28 ±15 | 0.97 | 12.2 |
Myocardial deformation | ||||||||
GLS | −13.9 ± 3.3 | −13.4 ± 2.9 | 0.81 | 10.2 | −6.3 ± 4.5 | −6.6 ± 3.9 | 0.91 | 23.4 |
LV dyssynchrony | ||||||||
SDI | 3.9 ± 1.1 | 4.0 ± 1.0 | 0.47 | 20.7 | 7.2 ± 3.2 | 6.6 ± 3.1 | 0.72 | 21.4 |
SDII | 4.0 ± 3.0 | 4.5 ± 3.5 | 0.42 | 37.5 | 11.1 ± 7.1 | 10.5 ± 7.3 | 0.87 | 26.4 |
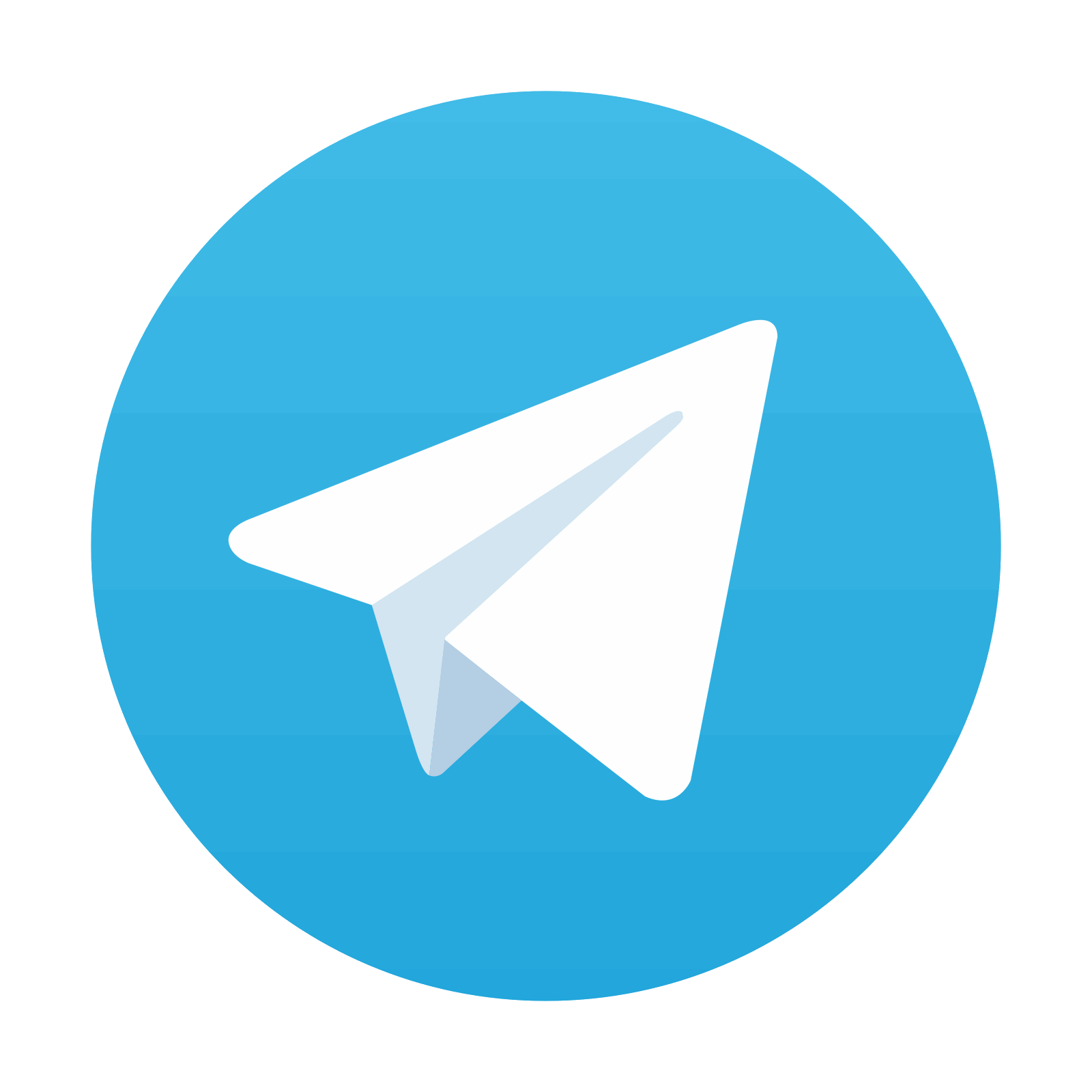
Stay updated, free articles. Join our Telegram channel

Full access? Get Clinical Tree
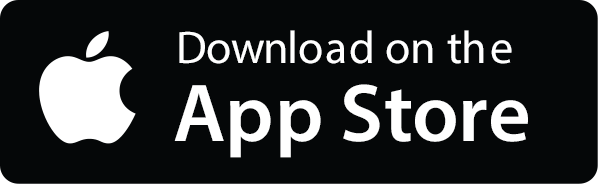
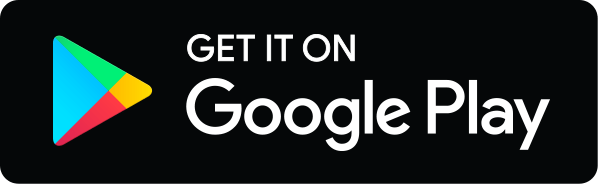
