Chapter 144
Renovascular Disease
General Considerations
Arsalla Islam, Randolph L. Geary
Based on a chapter in the seventh edition by Bryan W. Tillman and Randolph L. Geary
Renal artery disease encompasses a range of disorders affecting blood flow to the kidneys that are more prevalent than is generally recognized. Atherosclerosis, by far the most common cause of renal artery stenosis (RAS), affects 7% of elderly Americans1 and is the primary focus of this chapter (Fig. 144-1). Other lesions affecting renal blood flow include fibromuscular dysplasia2 (see Chapter 147), dissection and trauma (see Chapters 138, 149, and 158), arteritis (see Chapter 80), and congenital hypoplastic syndromes of the aorta and renal arteries (see Chapter 150).3,4 The importance of RAS relates primarily to its two major clinical manifestations—hypertension and impaired renal function—and their association with cardiovascular events, dialysis dependence, and mortality.5–7
Figure 144-1 Digital subtraction angiogram illustrating bilateral atherosclerotic renal artery stenosis.
Historical Background
In 1898, Tigerstedt and Bergman isolated a substance from rabbit kidneys that they called renin, which elevated blood pressure in experimental animals when it was reinfused.8 Their findings were controversial and initially dismissed until confirmed by others decades later.9 In 1905, Katzenstein linked experimental renal artery constriction to hypertension10; then in 1934, Goldblatt published elegant experiments showing that the hypertension from experimental RAS could be cured by nephrectomy.11 Goldblatt was also the first to demonstrate that the physiology of renovascular hypertension differed according to whether one or both renal arteries were involved, which he described as renin-dependent and volume-dependent hypertension, respectively.
The first successful treatment of renovascular hypertension was reported in 1938 by Leadbetter et al, who removed an ischemic ectopic kidney from a hypertensive child.12 Nephrectomy became an accepted treatment of hypertension in patients with a shrunken kidney, but results were unpredictable, with only one in four patients actually cured of hypertension.13 The first report of a direct renal artery reconstruction curing renovascular hypertension was in 1954 by Freeman and colleagues, who performed an endarterectomy for bilateral ostial lesions.14 Enthusiasm for renal revascularization increased dramatically after the introduction of aortography, and numerous reports of improved blood pressure control followed.15–18 By 1960, however, a critical reappraisal showed that blood pressure was improved in less than half of patients undergoing surgery.19 This inability to predict which patients would benefit from surgery led to pessimism about the utility of repair.
Ultimately, results of renal revascularization would improve only after the biochemical regulation of blood pressure was better understood. Once the renin-angiotensin-aldosterone axis was characterized and its role in renovascular hypertension defined, assays became available to accurately measure plasma renin activity.20–23 These and other functional tests ushered in an era of renal artery surgery in which physicians could more reliably identify patients likely to benefit from surgery.
Although hypertension has historically been the primary trigger for screening and intervention in renovascular disease, impaired renal excretory function from renal artery disease has a far greater impact on patient survival.24 Experiments early in the 20th century showed that the kidney was intolerant of warm ischemia and that renal artery obstruction caused renal atrophy in dogs.11 In 1962, in a study of eight patients with bilateral RAS, Morris, De Bakey, and Cooley showed that azotemia could be reversed by renal artery reconstruction.18 Similar reports followed from Dean and others, who coined the term ischemic nephropathy to describe renal insufficiency from impaired renal artery flow.25–29 Even more provocative were early reports of patients being removed from dialysis after renal artery reconstruction.30–32 The selection of azotemic patients who would benefit from surgery proved challenging, but subsequent studies identified patient characteristics predictive of improved or stabilized renal function after surgery.33,34
Finally, since the introduction of percutaneous angioplasty of the renal arteries by Gruntzig and associates in 1978,35 followed by balloon-expandable stents in 1989,36 endovascular treatment of RAS has increased exponentially. More than 21,660 renal revascularization procedures were performed in the United States under Medicare in 2000, the bulk of which were endovascular.37 Enthusiasm for renal artery stenting has outpaced clinical research to establish its niche in the contemporary management of renovascular hypertension or ischemic nephropathy. Recently published and ongoing prospective randomized trials of medical therapy versus renal artery stenting may help further refine the significant shortcomings we face in selecting patients likely to benefit from renal artery reconstruction (see Chapter 146).
Epidemiology
Prevalence of Anatomic Renal Artery Disease
Here we make the distinction between the presence of RAS defined by imaging (anatomic disease) and lesions resulting in clinical sequelae (functional disease).
Independent-Living Elderly
The prevalence of RAS, independent of associated hypertension or renal failure, has been estimated in population-based studies. In a community-based study of 870 independently living adults aged 65 years or older, the prevalence of critical RAS identified by duplex ultrasound screening was 7%.38 Of those, 12% had bilateral stenoses and 12% complete occlusion. Risk factors associated with stenosis included age, systolic hypertension, and reduced high-density lipoprotein cholesterol level. Importantly, only slightly more than half of affected individuals were clinically hypertensive, and in contrast to previous studies in high-risk populations, the prevalence was not significantly affected by ethnicity or gender.38,39
Autopsy Series
Additional estimates of the prevalence of RAS in the general population have come from autopsy series. These studies represent the only data currently available from unselected low-risk individuals of a variety of ages. In 1964, Schwartz and White reported a series of 154 consecutive autopsies in which the aorta and renal arteries were examined specifically for RAS.40 They found the prevalence of significant stenosis to be 6% in patients younger than 55 years and 40% in patients older than 75 years. Bilateral stenoses were common, found in approximately half of affected individuals, and the proximal renal artery was the most common lesion location. Holley and coworkers reported on 295 autopsies, excluded individuals with known intrinsic kidney disease, and reported a prevalence of 22% significant RAS, most commonly involving the proximal renal arteries.41
Prevalence of Renal Artery Stenosis in High-Risk Populations
Atherosclerotic Coronary Artery and Peripheral Arterial Disease
Given the systemic nature of atherosclerosis, the prevalence of RAS should increase in populations manifesting ischemia in other vascular beds. In fact, angiographic series have documented concomitant disease in 15% to 45% of patients being evaluated for extrarenal atherosclerosis (Table 144-1).38,42–52
The incidence of RAS in patients with coronary artery disease ranges from 15% to 22%.42,48 Jean and colleagues performed aortography in 196 patients undergoing heart catheterization and found that 18% of those with coronary artery disease had coexisting RAS42; Harding demonstrated an association between RAS and increasing angiographic severity of coronary artery disease.49 Some series reported a lower prevalence of RAS in African Americans39 and in women, but these observations contrast with population-based studies in which race and gender have little effect on the prevalence of RAS.1,38
The prevalence of RAS is also high among patients with severe peripheral50 and carotid atherosclerosis (see Table 144-1). In patients being treated for carotid stenosis, the prevalence of RAS is reportedly 20% to 27%.47,51,52 Miralles and colleagues screened 168 consecutive patients undergoing elective treatment of aortoiliac occlusive disease for concomitant RAS and found that 40% had a critical lesion of one or both renal arteries.43 The percentage increased to greater than 50% for the subset that also had carotid disease.43
Renal Insufficiency
Renal parenchymal injury in patients at risk of RAS can be complex and often multifactorial so that the contribution from hypoperfusion resulting directly from RAS is generally difficult to determine.53,54 Risk factors for renal failure (e.g., hypertension, diabetes, hyperlipidemia) overlap significantly with those for atherosclerosis, the primary cause of RAS, so it is not surprising that the prevalence is high in patients with chronic renal insufficiency (14%).55
RAS has been reported in 9% to 22% of patients with end-stage renal disease requiring dialysis,44,56,57 but the prevalence varies significantly by age, ethnicity, and gender. It is highest in elderly white men and surprisingly infrequent among African Americans with end-stage renal disease.43,44,55–57 In our experience, virtually all patients with ischemic nephropathy from RAS also present with severe hypertension.31 Those who are normotensive or only mildly hypertensive typically have another cause of their azotemia and are unlikely to benefit from revascularization.
Hypertension
Although not all patients with RAS exhibit hypertension,1,38 this is the most common clinical manifestation leading to diagnostic screening. Hypertension associated with RAS tends to be more severe and refractory to medical therapy compared with essential hypertension.1 This point is illustrated by a study of 500 patients undergoing surgery for renovascular disease in whom the average systolic blood pressure was 200 mm Hg and the average number of antihypertensive medications was 2.6 (additional demographics are provided in Table 144-2).58
Table 144-2
Demographics of Patient Population Undergoing Surgical Renal Revascularization
Parameter | Value |
Age | 65 ± 9 years |
Gender | 49% male, 51% female |
Systolic blood pressure | 200 ± 35 mm Hg |
Diastolic blood pressure | 104 ± 21 mm Hg |
Number of antihypertensive medications | 2.6 ± 1.1 |
Creatinine concentration (mean) | 2.6 mg/dL (230 µmol/L) |
Comorbid extrarenal atherosclerosis | 90% |
Coexisting coronary artery disease | 70% |
Diabetes | 16% |
Using duplex ultrasonography to screen 629 adults presenting with newly diagnosed hypertension, we found that 25% had RAS. This doubled for patients older than 60 years with severe hypertension (diastolic blood pressure > 110 mm Hg) and rose to 71% for the subset that also had renal insufficiency (serum creatinine concentration > 2.0 mg/dL [177 µmol/L]). Similarly, Holley and coworkers reported an extremely high prevalence of RAS (62%) in older patients with severe hypertension.41 Atherosclerotic RAS is not found in children, but hypertension in this age group can result from other anatomic lesions that reduce renal perfusion, so screening duplex ultrasound is often warranted (see Chapter 150). In a review of hypertensive children younger than 5 years, Lawson and coworkers reported that 78% had a correctable renin-mediated cause.46
Natural History of Renal Artery Stenosis
Anatomic Progression
Critical to decision making in the treatment of RAS is an understanding of the natural history when it is managed expectantly. Many patients whose lesions are identified incidentally or by screening are asymptomatic or have hypertension effectively controlled with medications, the etiology of which is likely to be “essential hypertension.” Some have argued that even in these patients, the consequences of lesion progression are great enough to warrant prophylactic renal artery reconstruction. Even in patients with severe hypertension, with or without renal insufficiency, understanding of the natural history of RAS is critical to properly balance risks and benefits in individuals being considered for reconstruction.
Older studies using serial angiography in high-risk populations with RAS reported progression of stenosis in 11% to 35% of patients.59–62 Approximately 10% of lesions progressed to complete occlusion, but only when the baseline stenosis was severe.59,61 Duplex surveillance has also been used to observe high-risk populations. Zierler and associates performed serial examinations of 80 patients with renovascular hypertension whose arteries were initially classified as normal, greater than 60% stenosis, or less than 60% stenosis.63 After 3 years, 8% of arteries initially classified as normal and 43% of those with subcritical blockages had progressed to greater than 60% stenosis. Progression to complete occlusion was observed in 7% of arteries with preexisting RAS greater than 60%. Factors associated with lesion progression included older age, higher systolic pressure, smoking, female gender, and poorly controlled hypertension.
Functional Progression
Individuals with RAS may or may not have severe hypertension or reduced renal function, but progression of renal artery disease has been associated with both, and their clinical consequences (progression to dialysis, cardiovascular morbidity, and death) provide the rationale for treatment. For instance, among 41 patients with renovascular hypertension who were randomized to medical therapy, Dean and coworkers found that 46% had a significant rise in serum creatinine concentration and 37% showed more than a 10% decrease in kidney length.59 Schreiber and colleagues also reported that a significant number of patients with greater than 60% RAS showed decline in renal function during follow-up, even when the degree of stenosis remained stable.60
In another series of 122 patients with renovascular hypertension observed prospectively, Caps and associates reported that 21% of kidneys with greater than 60% stenosis at baseline had lost more than 1 cm in kidney length after 2 years, with an associated rise in creatinine concentration.64 Risk of atrophy correlated with degree of stenosis and systolic hypertension. Others have confirmed a relatively high rate of progression of RAS with medical management, accompanied by kidney shrinkage, loss of excretory function,65 and even progression to dialysis in 7% to 12% of patients within 3 to 4 years.65,66
In a prospective longitudinal population-based study of the natural history of atherosclerotic RAS published by our group in 2006, we found that among 119 patients studied, 13 had significant RAS identified at initial screening, of which none progressed to complete occlusion.67 However, 9 kidneys without stenosis at baseline developed critical RAS on follow-up, including one occlusion. The overall incidence of progression to greater than 60% RAS after 8 years of follow-up was only 4%, a rate far lower than the previously mentioned series of highly selected high-risk patients.
Taken together, these studies demonstrate that progression of anatomic RAS is less likely in low-risk patients in whom RAS is found incidentally, whereas in high-risk groups with severe RAS, accelerated hypertension and loss of renal function are more common. In addition, the risk of progression is highest in the elderly with advanced, preexisting renal artery disease.
Morbidity
The major risks of RAS are excess cardiovascular morbidity and mortality and progression to dialysis dependence from associated hypertension and loss of excretory renal function. A significant increase in end-organ damage has been reported in patients with renovascular hypertension compared with patients with essential hypertension, as revealed by left ventricular hypertrophy, elevated creatinine concentration, and proteinuria.68 These differences were found despite matching patients for hypertension severity. Mailloux and coworkers projected that renal artery disease accounts for 10% to 18% of incident dialysis dependence among the elderly.56 This is especially troublesome, given the profound reduction in life expectancy (median survival, 25 months) and quality of life and the high cost of dialysis in the elderly.56
Others have demonstrated an association between RAS and cardiovascular events, including stroke and perioperative morbidity.69,70 In 1235 patients with coronary artery disease demonstrated by arteriography, Conlon and associates showed that those with greater than 50% RAS had a markedly reduced 4-year survival (67% vs. 88%), primarily from excess cardiovascular events.71
The link between renovascular disease and cardiovascular events may include accelerated progression of existing atherosclerosis, altered lipid metabolism associated with renal insufficiency, heart hypertrophy from hypertension, and other adverse systemic effects of excess renin and angiotensin II on the cardiovascular system.44,72 Until recently, no prospective or population-based studies addressed this question. In 2004, Edwards and colleagues reported the risk of adverse coronary events among 870 independently living individuals older than 65 years in a cohort of the Cardiovascular Health Study.73 All were screened for significant RAS by duplex ultrasound, and during a mean follow-up of only 14 months, the 7% of participants with greater than 60% RAS had twice the number of cardiovascular events, after controlling for coexisting risk factors including hypertension and prevalent cardiovascular disease.
Pathogenesis
Etiology of Renal Artery Lesions
The most common pathologic process underlying renal artery disease is atherosclerosis. To illustrate this point, 91% to 98% of patients undergoing endovascular treatment of RAS have atherosclerotic lesions.74,75 Endovascular series may be biased toward atherosclerosis because procedures are often performed in patients being studied or treated for atherosclerosis in other arterial beds. In 840 patients undergoing open renal artery reconstruction at our institution from 1987 to 2008, excluding patients who underwent combined repair of aortic aneurysms or aortic occlusive disease, 81% of RAS was atherosclerotic, 14% fibromuscular dysplasia, and 1% from dissection. Children with hypoplastic renal arteries, midaortic syndrome, or dissection accounted for 3% of this cohort.
Atherosclerotic renal artery disease commonly involves the renal ostia, but stenoses may occur at any level within the renal arteries, including small intraparenchymal vessels.40,41 Cross-sections of renal artery plaques show histologic features typical of atherosclerotic lesions in other vascular beds, with variable accumulation of monocytes and lymphocytes, smooth muscle cells, lipid, and mineralization (see Chapter 5). Peculiar to ostial renal and visceral artery lesions and relevant to their treatment is the finding that the first few millimeters of ostial plaque is contiguous with a sheet or sleeve of aortic plaque oriented perpendicular to the branch artery (Fig. 144-2). The aortic plaque is typically thick and heavily calcified, creating a rigid sheet that resists balloon dilatation. For this reason, balloon angioplasty alone has been abandoned in favor of balloon-expandable stents when an endovascular repair is indicated for ostial RAS.76
Pathophysiology of Renovascular Hypertension
Renovascular hypertension results from a complex cascade of events precipitated by relative kidney hypoperfusion beyond a stenosis. The renal parenchyma responds by activation of the renin-angiotensin-aldosterone axis, which in turn leads to peripheral vasoconstriction and intravascular volume expansion.9 For most patients, the onset of renovascular disease is insidious, resulting in chronic hyper-reninemia before intervention, causing secondary adaptive hypertrophy and remodeling of the heart and vasculature that accentuate and sustain hypertension and potentially limit benefits of arterial reconstruction.
Juxtaglomerular cells release renin in response to decreased pressure, which in turn catalyzes cleavage of circulating angiotensinogen synthesized by the liver to the decapeptide angiotensin I. Angiotensin-converting enzyme (ACE) then cleaves angiotensin I to form the octapeptide angiotensin II, the primary effector of renin-induced hypertension.9,77 This cascade occurs locally within the kidney, within the systemic circulation, and in many extrarenal tissues. ACE is produced in high levels in lung but is also active in the glomerulus, endothelium, heart, and brain.78,79 Other proteases have also been identified that can cleave angiotensin I, including tonin, cathepsin, chymase, and kallikrein.77,80 Excessive angiotensin II formed in extrarenal tissues through these local renin-angiotensin systems contributes further to disease.
The effects of angiotensin II are mediated by signaling downstream of its receptors, angiotensin receptor types 1 and 2. Angiotensin receptor type 1 (ATR1) is a G protein–coupled receptor that activates vasoconstrictor and mitogenic signaling pathways in cells resident within blood vessels; its ligation causes peripheral arteriolar vasoconstriction and stimulates renal tubular reabsorption of sodium and water.9,77 Crosstalk between ATR1 and other receptor classes (e.g., receptor tyrosine kinases such as platelet-derived growth factor receptors) can amplify smooth muscle cell vasoconstrictor and mitogenic signaling.81 ATR1 activation also induces expression of the potent vasoconstrictors norepinephrine and endothelin-1.82,83 ATR1 ligation can also upregulate peptide growth factors (and their respective receptors), including epidermal growth factor and vascular endothelial growth factor, and stimulate the formation of reactive oxygen species such as superoxide that can inactivate the vasodilator nitric oxide. These actions promote vasoconstriction and hyperplastic remodeling of the wall of peripheral arteries and arterioles.
Angiotensin II also promotes intravascular volume expansion directly, by activating ATR1 on renal tubular cells to increase sodium absorption, and indirectly, by stimulating adrenal cortical release of aldosterone, which also promotes renal tubular sodium reabsorption.84 The resulting volume expansion can be blunted by a compensatory natriuresis from an opposite normal kidney.11,85,86 Patients with one functional kidney or bilateral RAS cannot mount this compensatory natriuresis, and the result is so-called Goldblatt volume-dependent hypertension.11
Angiotensin II further contributes to hypertension by acting on specific nuclei in the brain. Glial cells and regions of the brain responsible for blood pressure regulation also express both ACE and ATR1.87 Angiotensin II increases renal sympathetic activation and also stimulates release of vasopressin from the pituitary, which in turn stimulates thirst and water reabsorption in the kidney to expand intravascular volume and causes peripheral vasoconstriction.
The importance of angiotensin II is made apparent after pharmacologic inhibition of ACE or ATR1 with ACE inhibitors and angiotensin receptor blockers (ARBs), respectively. Both are effective in treating renovascular hypertension from unilateral disease and may provide an indirect if unintentional diagnostic intervention when prescribed to patients with bilateral RAS in whom loss of glomerular arteriolar tone may lead to an acute decompensation in filtration and rise in creatinine concentration. This effect is generally reversible once the medication is stopped.88
Although it is initially mediated by vasoconstrictor and hypervolemic effects of angiotensin II, the mechanism underlying renovascular hypertension changes over time. Chronic angiotensin II–mediated remodeling of the heart and resistance vessels contributes to sustained or amplified hypertension and associated cardiovascular embarrassment.89–92 The nature of vascular remodeling seen in RAS is distinct from that observed in essential hypertension, in which resistance vessels exhibit eutrophic remodeling, with little change in wall mass. In contrast, patients with RAS demonstrate hypertrophic vessel remodeling with expansion of the medial layer,93 which is responsive to ACE inhibitors.94 Blocking of angiotensin II production can also improve cardiac remodeling and inhibit vascular hypertrophy, endothelial dysfunction, thrombosis, and platelet aggregation.95,96
Pathophysiology of Ischemic Nephropathy
The term ischemic nephropathy has been used for decades to describe renal insufficiency from RAS, but its molecular basis remains incompletely defined.97 Hypoperfusion results in impaired glomerular filtration,98 but the role of frank ischemic necrosis is less well established.34,99 Acute renal artery occlusion causes ischemic infarction of the affected kidney within minutes to hours, but a gradual decrease in renal blood flow impairs renal excretory function through a number of pathways.
Clinically, the relationship between renovascular disease and loss of excretory function is strongly associated with elevated blood pressure.24 Mechanistically, as for renovascular hypertension, angiotensin II appears to play a central role. By increasing efferent arteriolar tone,100 angiotensin II increases glomerular capillary pressure to maintain filtration in the presence of reduced renal perfusion.9 However, secondary effects of chronic angiotensin II excess within the kidney include ATR1-induced expression of several profibrotic cytokines and growth factors.
Angiotensin II increases the expression of transforming growth factor-β, a master regulator of extracellular matrix production, and platelet-derived growth factor, a potent mitogen for smooth muscle cells, renal fibroblasts, and glomerular mesangial cells essential for normal renal function.78,79,81 Angiotensin II also induces nuclear factor-κB, a potent master switch for proinflammatory gene expression pathways. Excess aldosterone has also been shown to activate transforming growth factor-β and thereby contribute to glomerulosclerosis and tubulointerstitial injury.101–107 Evidence is also emerging that atherosclerotic renal artery lesions themselves contribute to loss of excretory function from chronic spontaneous release of atheroembolic debris, causing significant damage to the kidney.108
In the setting of unilateral RAS, the opposite kidney suffers injury directly from severe hypertension and indirectly from sustained elevations in circulating angiotensin II or plasma aldosterone levels, leading to afferent and efferent arteriolar vasoconstriction with sustained decrease in glomerular filtration rate along with afferent arteriolar hypertrophy and ultimately arteriosclerosis. Once established, the side effects persist despite subsequent medical control of renovascular hypertension, repair of the opposite RAS, or even nephrectomy of the opposite ischemic kidney.
Clinical Presentation
History and Physical Examination
Roughly half of individuals with anatomic renal artery disease have no associated symptoms. Those with functional lesions typically present with severe hypertension, with or without reduced excretory renal function. Other symptoms, physical findings, and laboratory abnormalities are nonspecific, so practitioners need to be aware of which individuals are at greatest risk. Renal artery disease should be in the differential diagnosis of any child with unexplained hypertension.109 Advanced age, severe hypertension refractory to multiple antihypertensive agents, and history of coexisting coronary artery or peripheral arterial disease should raise suspicion in adults. Although renal artery disease often leads to impaired renal function, most patients who develop renal failure have another underlying cause, making it difficult to assign cause and effect to RAS. In our experience based on responses to open surgical revascularization, virtually all patients with reversible ischemic nephropathy have severe coexisting hypertension. Therefore, azotemia in the absence of hypertension, even in a patient with documented RAS, suggests the presence of coexisting intrinsic renal disease that is irreversible or of another cause.
A scenario that may be diagnostic of bilateral RAS is a patient who presents in acute renal failure on starting treatment with an ACE inhibitor or ARB.88,89 Increased efferent arteriolar tone induced by angiotensin II is a critical compensatory mechanism to maintain filtration pressure in bilateral renal artery disease. Pharmacologic blockade of efferent arteriolar constriction by ACE inhibitors or ARBs may lead to acute decrease in filtration with rise in creatinine concentration. When it is recognized early, simply stopping the culprit medication results in the return of renal function to baseline, and subsequent imaging confirms the presence of bilateral RAS. On the other hand, the presence of bilateral renal disease should not preclude treatment with an ACE inhibitor or ARB as these are often beneficial in patients with atherosclerosis, and not all patients with bilateral RAS will decompensate on therapy.88 These patients will need careful follow-up, however, to be certain that estimated glomerular filtration rate or creatinine concentration remains stable.88
Individuals with acute exacerbations of unrecognized or poorly controlled renovascular hypertension may present in “hypertensive crisis.” Long-standing hypertension can lead to compensatory myocardial hypertrophy with abnormal diastolic relaxation. These patients are particularly sensitive to volume overload and present in congestive heart failure or “flash” pulmonary edema. Severe hypertension can also lead to neurologic symptoms ranging from headache and visual disturbances to overt stroke. The severe increase in hydrostatic pressure drives fluid into the extravascular space, causing edema of the extremities and internal organs, including the brain. Acute hypertension can also damage the kidney, particularly in unilateral RAS, resulting in proteinuria and elevated creatinine concentration. As blood pressure is normalized, proteinuria and azotemia often resolve and return to baseline. In this setting, it is important to allow a patient with acute hypertensive nephropathy time to recover before imaging with iodinated contrast agents is performed.
On physical examination, patients may have severe elevation of systolic and diastolic blood pressure, abdominal bruits, and nonspecific stigmata of heart and atherosclerotic vascular disease.33,110 Diminished lower extremity pulses may be present in children with coarctation or midaortic syndromes and in adults with atherosclerotic peripheral arterial disease. Pulse deficits may also be found in young adults with Takayasu’s arteritis111 or in patients presenting with aortic dissection and malperfusion.
Laboratory Findings
Although nonspecific, blood chemistries may show elevated urea nitrogen and creatinine concentrations. The electrocardiogram may show a strain pattern with increased voltage, consistent with left ventricular wall thickening. Echocardiography can confirm ventricular hypertrophy with impaired diastolic relaxation. Specialized biochemical assays, such as plasma renin levels, are rarely useful in the contemporary diagnosis of renovascular disease unless they are combined with selected invasive renal vein renin sampling (see Renal Vein Renin Assays). Measurement of plasma aldosterone in patients with hypokalemia may identify those with primary hyperaldosteronism (Conn’s disease), and assays of urine metanephrines or serum catecholamines can identify metabolically active adrenal tumors leading to hypertension (pheochromocytoma).112
Diagnostic Evaluation
Noninvasive Imaging
Duplex Ultrasonography
The ideal imaging modality for renal artery disease would be a rapid, noninvasive study with excellent resolution, accuracy, and reproducibility. It would carry minimal risk to the patient and be inexpensive. Of all the tools presently available, color duplex ultrasonography best fits this profile and has become the primary method of screening for renal artery disease in many centers. Duplex ultrasonography avoids ionizing radiation and iodinated contrast material, does not require intravenous access, and is safe in patients with medical implants. The primary limitation of renal duplex imaging is that it is technically demanding relative to other studies performed in the average vascular laboratory, so accuracy is highly operator dependent, ranging from 60% to more than 90%. Study times can range from 10 to 60 minutes, depending on the patient’s body habitus and the technician’s experience. Factors that may interfere with insonation of the renal artery include excessive bowel gas, obesity, and altered resistance to flow from advanced renal parenchymal disease. In our experience, these factors contribute to incomplete examinations in about 4% of patients.113
Patients are fasted overnight to reduce bowel gas, and the examination begins from an anterior approach with the patient supine. The aorta is examined with a 5-MHz curved linear array transducer, using color-flow imaging to exclude aneurysms, stenosis, or occlusion of the perirenal aorta. A peak aortic velocity is then measured at the level of the superior mesenteric artery using an angle of insonation of 60 degrees or less. The main renal arteries are then studied and velocity waveforms recorded from the proximal, mid, and distal vessel. The patient is then studied in left and right lateral positions, and velocity waveforms are again recorded from the proximal, mid, and distal renal arteries, maintaining an angle of insonation of 60 degrees or less. Renal veins are evaluated for patency by color Doppler study, and phasic waveforms are confirmed with pulsed Doppler study. Velocity waveforms are also recorded from the renal parenchyma in the superior, mid, and inferior poles to calculate a resistive index (peak systolic velocity—end diastolic velocity/peak systolic velocity) and acceleration times (interval from onset of systole to peak systole, in milliseconds). The examination is completed by recording kidney length.
The accuracy of duplex ultrasonography in detecting significant RAS, validated against conventional arteriography, can exceed 90% in experienced hands.113–116 Although a number of criteria have been reported to predict critical stenosis, the most consistent has been an increase in peak systolic velocity in the main renal artery (e.g., >1.8 or >2.0 m/s)113,115,117 associated with post-stenotic turbulence.118,119 In 74 consecutive patients undergoing renal arteriography, duplex ultrasonography correctly identified lesions causing greater than 60% stenosis with 93% sensitivity, 98% specificity, and 96% accuracy in kidneys with single renal arteries.113 Sensitivity decreased to 86% in kidneys with multiple renal arteries (15% of kidneys), but specificity remained high at 98%.113 Some centers use the ratio of renal artery to aortic peak systolic velocity, with a ratio of more than 3.5 defining stenoses exceeding 60%.120,121 Similar data have been reported from a number of centers,51,115,117,122–124 but others have found the ratio to be less useful or inaccurate.125,126
Attempts to simplify the duplex protocol for diagnosis of critical RAS include studies of renal blood flow in the hilar vessels and within the kidney parenchyma well beyond the actual stenosis. A flank approach is used to record Doppler waveforms from the hilum or parenchyma, and blunted waveforms with delayed systolic upstroke are indicative of significant proximal disease. Acceleration time is used to estimate the associated delay in systolic upstroke by calculating the interval from onset of systole to its peak. Acceleration times exceeding 100 milliseconds suggest a critical stenosis within the proximal renal artery.118 A number of investigators have documented a reasonable correlation between elevated acceleration time and stenosis on conventional angiography.116,127 In our experience, though, acceleration time has a high positive predictive value (97%), but the sensitivity is too low for this to be used alone as a screening criterion.118
The resistive index has also been used to indirectly identify critical RAS. A normal value is less than 0.7, and values greater than 0.8 can indicate critical RAS, but abnormal results are not specific and more often are caused by intrinsic renal disease. Renal parenchymal scarring and fibrosis lead to elevated resistance to flow (e.g., nephrosclerosis, glomerular sclerosis) independent of main renal artery disease. Radermacher and colleagues described 131 patients undergoing renal revascularization and found that a resistive index above 0.8 predicted poor hypertension response and progression of renal insufficiency after revascularization, consistent with irreversible intrinsic medical renal disease.128 Conversely, 94% of patients with an index below 0.8 had improved blood pressure and lack of progression to dialysis after reconstruction. The role of the resistive index in managing patients with RAS remains controversial, and we have largely abandoned its use in guiding patient care.119
At Wake Forest, we currently base the duplex diagnosis of anatomic RAS (>60% stenosis in the proximal renal artery) on a focal increase in peak systolic velocity to more than 1.8 m/s with post-stenotic turbulence or absent Doppler signal from an imaged artery indicating complete occlusion.118 Although we collect the other data described before, they are complementary to the peak systolic velocity. In our experience, renal-aortic velocity ratios, resistive indices, acceleration times, and hilar analysis have proved less reliable and thus are seldom used in clinical decision making.118,119
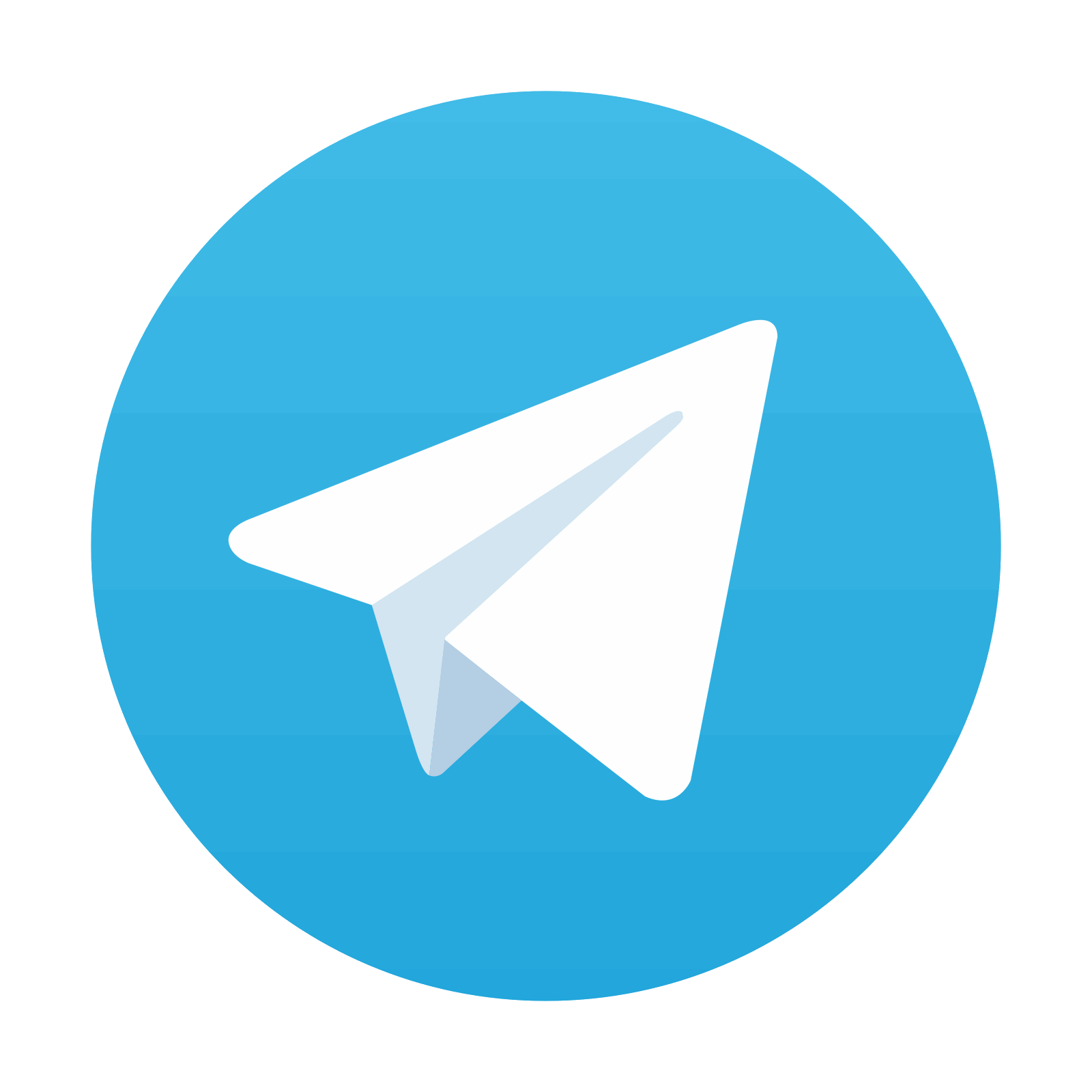
Stay updated, free articles. Join our Telegram channel

Full access? Get Clinical Tree
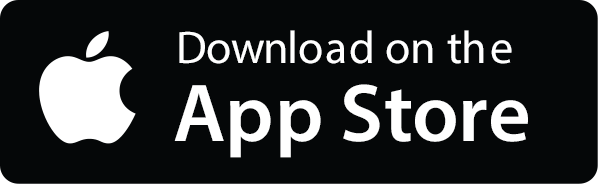
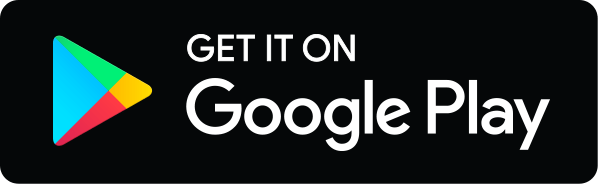