Major advancements in vascular imaging techniques have facilitated the diagnosis of renovascular disease, and modern medical therapies as well as surgical and percutaneous interventional techniques offer the clinician a wide array of therapeutic tools in the management of renovascular disease. Despite these developments however, the indications for medical therapy versus surgical or percutaneous revascularization are controversial, and the clinician’s ability to predict the effect of revascularization on clinical outcomes remains limited.
Vascular lesions affecting the renal artery can be caused by atherosclerosis, fibromuscular dysplasia (FMD), aneurysms, congenital or traumatic arteriovenous fistula (AVF), extrinsic compression, trauma, and embolization. The overwhelming majority of arterial artery lesions, however, are secondary to either atherosclerosis or FMD, which will be discussed below. A separate section will be dedicated to renal artery aneurysms, arteriovenous malformations (AVMs), and spontaneous renal artery dissections.
Often clinically silent and discovered incidentally, FMD accounts for less than 10% of cases of renal artery stenosis (RAS), and although it can affect the intima, in the majority of cases it involves the media, resulting in the typical “string of beads” appearance (Figure 32-1).1 The cause remains largely unknown; however it may have a genetic component and it is more frequent in hypertensive patients and smokers.2 FMD usually affects women between 15 and 50 years of age, but it can be also observed in males and older patients as well.1 It occurs most frequently in the renal artery, but can also involve the carotid and vertebral arteries, sometimes in association with intracranial aneurysms, as well as other visceral vessels.1,3,4
FIGURE 32-1.
Fibromuscular dysplasia with “string of beads” appearance involving the mid and distal portions of the renal artery. Differently from atherosclerotic renovascular disease, FMD typically spares the ostium of the renal artery.
Reprinted with permission from Slovut DP, Olin JW. Fibromuscular dysplasia. N Engl J Med. 2004;350(18):1862–1871.

In a series of angiogram of potential renal donors, incidental renal FMD was found in 3.8% patients, 75% of whom were females; in another study of patients with resistant hypertension screened with angiography, 16% had FMD.5,6 Because it is a disease of young patients with few cardiovascular risk factors, FMD is easily distinguishable from atherosclerotic RAS. FMD, unlike atherosclerotic RAS, tends to affect the mid and distal portion of the renal artery, and not the ostium. Its appearance can sometimes resemble vasculitis, but FMD is not an inflammatory process and it lacks the systemic manifestations and abnormal markers typical of vasculitis.
The severity of stenosis can be difficult to accurately measure with both noninvasive testing as well as with catheter-based angiography, but progression of disease has been documented in up to 37% of patients.7,8
Atherosclerosis accounts for approximately 90% of all renovascular lesions, and it usually involves the ostium and the proximal portion of the artery, although occasionally it can extend more distally.9
Atherosclerosis of the renal artery is more prevalent in the elderly, in patients with diabetes, and in patients with evidence of coronary or peripheral vascular disease elsewhere. In elderly white and black patients participating in the Cardiovascular Health Study (CHS), the prevalence of significant (≥60%) RAS detected by renal duplex sonography was 6.8%.10 Renovascular disease was not correlated to ethnicity, but was independently associated with age, hyperlipidemia, and hypertension. In a series of hypertensive patients undergoing coronary angiography, 19% had ≥50% stenosis and 7% had ≥70% stenosis by quantitative angiographic analysis. In a larger series of 3987 patients undergoing coronary angiography, aortography demonstrated ≥75% RAS in 4.8% patients.11 In 3.7% patients, the renal arteries were affected bilaterally.12 In patients with aortic aneurysms, aorto-occlusive or lower-extremity occlusive disease greater than 50% stenosis was present in more than 30% of patients.13
The increased prevalence of RAS in patients with coronary or peripheral arterial disease reflects the systemic nature of atherosclerosis and the overlapping existence of the disease in multiple vascular beds.
Atherosclerotic RAS is a progressive disease. In a series of 295 kidneys followed by renal artery duplex scans, the 3-year cumulative incidence of renal artery disease progression stratified by initial degree of stenosis was 18%, 29%, and 49% for renal artery classified as normal, with <60% stenosis and with ≥60% stenosis, respectively.14 In this study, there were nine occlusions which occurred in patients who had ≥60% stenosis at the time of initial evaluation. Schreiber et al., however, have reported progression to total occlusion in 39% of patients with ≥75% stenosis at renal arteriography.15 In the Dutch Renal Artery Stenosis Intervention Cooperative study, a randomized trial of medical therapy versus balloon angioplasty for the treatment of hypertension in RAS patients, progression to complete occlusion occurred in 16% of patients treated medically.16
Besides the initial degree of stenosis, other factors related to disease progression include diabetes mellitus and hypertension; interestingly, progression of RAS is also found in patients in whom optimal blood pressure control has been achieved with medical therapy.17
RAS can result in decreased renal size. In a series of 204 kidneys in 122 subjects, Caps et al. reported a 2-year cumulative incidence of renal atrophy of 5.5%, 11.7%, and 20.8% in kidneys with normal renal arteries, with <60% stenosis and with ≥60% stenosis.18 Furthermore, RAS is the documented cause of end-stage renal artery disease (ESRD) in up to 15% of patients initiating dialysis each year, and in these patients, the overall prognosis is worse than in ESRD secondary to other etiologies.19,20 Even in patients who have not reached ESRD, the presence of RAS is related to increased mortality, with 2-year survival rates of 96% in patients with unilateral RAS, 74% with bilateral RAS and 47% in RAS affecting a solitary functioning kidney.21,22
Experimental studies of renovascular hypertension pioneered by Goldblatt et al. demonstrated that renal artery obstruction is followed by an increase in arterial blood pressure, and identified important differences in the pathophysiology of hypertension in unilateral and bilateral RAS.23
In unilateral RAS, the normal kidney counterbalances the pressor mechanisms triggered by the stenotic kidney. Reduction in perfusion of the affected kidney causes renin release, leading to the formation of angiotensin II and, in turn, to increased plasma aldosterone levels, sodium retention in the underperfused kidney, and peripheral vascular resistance. The contralateral kidney counterregulates by responding to increased pressure with urinary sodium excretion (“sodium natriuresis”) and suppression of renin release, which tend to lower blood pressure and decrease perfusion pressure in the stenotic kidney. This, in turn, leads to increased renin and angiotensin production in a self-perpetuating cycle. In this situation, systemic hypertension is secondary to the pressor effect of angiotensin II. In fact, blockade of angiotensin action in the “two-kidney, one clip” rat model can completely prevent the increase in systolic blood pressure, at least in the early phases.24
Angiotensin II, however, can also increase blood pressure by modulating autonomic nervous system activity, resulting in neurogenic vasoconstriction, and has complex effects on the formation of reactive oxygen species with vasoconstrictive properties, as demonstrated by the detection of increased levels of isoprostanes in experimental models.25,26 Recent work has demonstrated impaired endothelial function, as assessed by response of forearm blood flow to acetylcholine, in patients with renovascular hypertension, with improvement after renal angioplasty, thus suggesting that excessive oxidative stress may indeed be involved in impaired endothelium-dependent vasodilatation.27
Vasoconstriction can also be mediated by endothelin and thromboxane A2, both upregulated in renovascular hypertension.28,29 The complex interplay of these diverse mechanisms varies individually and over time, partially explaining the difficulties in predicting reversal of hypertension after renal artery revascularization procedures. During the early phases of renovascular hypertension, treatment of RAS may indeed result in the resolution of the blood pressure abnormalities, but in later stages, when angiotensin II levels maybe lower or normal and hypertension may have transitioned to a less reversible state, it may become ineffectual.
In bilateral RAS, or in RAS affecting a solitary kidney, the “one-kidney, one clip” (1K1C) rat experimental model exposes a different mechanism in the pathophysiology of hypertension, one in which the entire renal mass is underperfused. In this case, activation of the renin-angiotensin-aldosterone system is not counterbalanced by a normal contralateral kidney, and pressure natriuresis cannot occur. Hence volume expansion ensues, resulting in hypertension and circulatory congestion. Finally, the positive volume status can result in normalization of renal perfusion, resulting in decreased plasma renin and angiotensin II. In this situation, volume expansion is critical. However, if volume status is reduced by sodium restriction and/or diuresis, hypertension will return to its renin-mediated form; hence sodium and volume expansion can suppress the renin-angiotensin system even in renovascular disease.30,31
Differences in function and hormonal status in unilateral and bilateral RAS portend diagnostic and clinical implications. Side-to-side differences between the kidneys have in fact been employed in a variety of diagnostic tests and preinterventional functional studies, which have been largely supplanted by the advent of modern diagnostic imaging studies.
Patients with bilateral RAS are more prone to vascular congestion and pulmonary edema, tend to have more severe hypertension, can experience deterioration in renal function with angiotensin-converting enzyme (ACE) inhibitors and angiotensin II receptor blockers (ARB), and have a higher mortality.32,33
The term “ischemic nephropathy” refers to the deterioration of renal function occurring in renovascular disease, and which can lead to ESRD in 14% to 20% of affected patients.19,34
The nature of ischemic nephropathy is complex and multifactorial. Since the main function of the kidney is filtration, renal blood flow is among the highest of all organs, and only 10% is necessary for this organ’s metabolic needs.35 Furthermore, the kidney is capable of autoregulating blood flow in the presence of RAS of up to 75% diameter reduction and, in conditions of impaired perfusion, oxygen delivery can be maintained by the development of collaterals from the adrenal and lumbar arteries.36 Given the oversupply of oxygenated blood, some authors have therefore suggested the alternative term of “azotemic renovascular disease” or “hypoperfusion injury” to describe the parenchymal changes resulting from chronic renal hypoperfusion, ultimately leading to fibrosis and ESRD.
In acute ischemic renal injury, rapid changes in perfusion can damage the outer medulla, which is less efficiently autoregulated than the cortex, thus resulting in acute tubular necrosis (ATN). In chronic hypoperfusion, however, the medulla is protected at the expenses of cortical blood flow, and the gradual reduction in renal perfusion results in the interplay of protective mechanisms, which result in morphologic and functional changes different from the ones observed in acute ischemic injury, and which remain incompletely elucidated.37
Proposed pathways activated in chronic renal hypoperfusion, and which can lead to parenchymal injury and interstitial fibrosis, are depicted in Figure 32-2, and involve the complex and interrelated effects of angiotensin II, nitric oxide (NO), endothelin, vasodilating and vasoconstrictive prostaglandins, and a variety of cytokines.
FIGURE 32-2.
Proposed mechanisms of parenchymal injury and interstitial fibrosis secondary to renal hypoperfusion. The figure outlines the complex and interrelated effects of angiotensin II, nitric oxide (NO), endothelin, vasodilating and vasoconstrictive prostaglandins, and of a variety of other cytokines. Some of these alterations develops in the absence of true ischemia.
Reprinted with permission from Lerman L, Textor SC. Pathophysiology of ischemic nephropathy. Urol Clin North Am. 2001;28(4):793–803, ix.

Angiotensin II maintains glomerular filtration pressure and glomerular filtration rate (GFR) by constricting the efferent arterioles, but its effects in the kidney also include local inflammatory responses, cell hypertrophy, and hyperplasia, which are mostly mediated by AT1 receptors.38 In fact, when angiotensin I is infused in rat experimental models, it results in focal and segmental glomerulosclerosis, which can be prevented by the AT1 blocker losartan.39 Other angiotensin II effects also include vascular smooth muscle proliferation, mesangial cell growth, platelet aggregation, activation of adhesion molecules and macrophages, induction of gene transcription for proto-oncogenes, and oxidation of low-density lipoproteins.37,40
Angiotensin II-mediated vasoconstriction is modulated by endogenous vasodilator prostaglandins and by NO, limiting the potentially ischemic effects of this hormone.41 NO downregulates gene expression of angiotensin II-type 1 receptor, a mechanism possibly implicated in the antiatherogenic effects of NO.42 NO also inhibits the growth of vascular smooth muscle cells, mesangial cell hypertrophy and hyperplasia, as well as synthesis of extracellular matrix.37,43 In RAS, diminished “shear stress” distal to the stenotic segment results in the production of NO, while decreased renal perfusion causes release of renin and generation of angiotensin II, thus shifting the balance in favor of vasoconstriction mediated by angiotensin II and by vasoconstrictive prostaglandins such as thromboxane. In fact, as RAS progresses, regulation of blood flow becomes less dependent on NO and more dependent on prostaglandins.44
While the synthesis of prostaglandins such as prostacycline and prostaglandin E2 protect the kidney from ischemia, thromboxane A2 mediates some of the deleterious effects of angiotensin II, and its synthesis increases in renovascular hypertension.29 Many of the vascular effects of angiotensin II are mediated by endothelin, a very powerful vasoconstrictive peptide, which is released from the renal epithelial cells in renal ischemia after stimulation by thrombin and cytokines like transforming growth factor-beta (TGF-β), interleukin-1, and tumor necrosis factor (TNF).45,46 These and other mechanisms, such as the generation of free oxygen radicals, interact with each other, eventually resulting in renal scarring even in the absence of “true” renal ischemia.26
The complexity and variability of these interactions in different individuals is another factor that makes predictions on the recovery of kidney function after revascularization difficult, and explains why patients with impaired renal function before revascularization may have no significant increase in their GFR after percutaneous or surgical interventions.31,47
RAS can be often asymptomatic and found incidentally and, as reviewed above, it is usually secondary to either FMD or atherosclerosis (Table 32-1).6,13 Although incidentally discovered RAS is common, renovascular hypertension occurs only in 1% to 5% of all patients with hypertension.13,48
Asymptomatic | Incidental |
---|---|
Renovascular hypertension | Accelerated hypertension Malignant hypertension Resistant hypertension |
Ischemic nephropathy | Renal insufficiency Kidney atrophy |
Cardiovascular manifestations | Recurrent pulmonary edema Angina pectoris Stroke |
The predominant manifestation of FMD is hypertension, most commonly in patients younger than 30 years and predominantly in women. Atherosclerotic RAS typically occurs in patients older than 50 years, and can be accompanied by other clinical manifestations of atherosclerosis, renal insufficiency, or by recurrent episodes of congestive heart failure and pulmonary edema.9,49 Accelerated and malignant hypertension are both suggestive of RAS, but renovascular hypertension can also present less dramatically, and can sometimes be difficult to differentiate from essential hypertension.50 Characteristics such as the absence of a family history of essential hypertension, duration of hypertension of less than 1 year, and the presence of an abdominal bruit and hypokalemia are more suggestive of the diagnosis of renovascular hypertension.9,51 RAS can be associated with both systolic and diastolic hypertension, but the diagnosis should be more strongly suspected in the presence of new-onset diastolic hypertension in patients older than 55 years.
Another common presentation of renovascular hypertension is the development of resistant hypertension in patients with previously well-controlled blood pressure, as defined by failure to reduce blood pressure to less than 140/90 mm Hg with a regimen of three drugs, which include a diuretic.52
A discrepancy in renal size can also be a clue to the presence of renovascular hypertension, and should prompt further diagnostic investigations.13,53
Azotemia in the setting of therapy with an ACE inhibitor maybe an indication of bilateral RAS or of RAS of a solitary functioning kidney, underscoring the vasoconstrictive role of angiotensin II on the efferent arterioles to preserve GFR in these situations.54
Patients with recurrent “flash” pulmonary edema, or with angina pectoris in the absence of any other causes, should also be evaluated for RAS. In the first case, symptoms maybe secondary to bilateral RAS, when pressure natriuresis cannot occur and vascular congestion ensues, while in the latter increased left ventricular afterload may play a role.32,55,56 In these cases, renal artery revascularization can result in a marked symptomatic improvement, possibly also as a result of improved blood pressure control.55,57
A summary of clinical clues suggestive of RAS is reported in Table 32-2. It is important to understand, however, that the differences between the clinical manifestation of RAS and those of essential hypertension can be small, and that none of the clinical characteristics mentioned above has, in itself, a high predictive value for RAS. Nevertheless, a recent study, which combined the presence of vascular disease in other vascular districts with abdominal bruits, body weight, smoking, and other factors into a clinical score, found it to be of good predictive value for RAS in patients referred for resistant hypertension.5
Hypertension Onset of hypertension before age of 30 years or after after age 55 years Accelerated or malignant hypertension in any age group Hypertension resistant to three or more antihypertensive drugs including a diuretic Acute onset or worsening of previously well controlled hypertension in any age group Renal Functional or Anatomic Abnormalities Impairment of renal function or acute renal failure associated with ACE inhibitors Hypertension with unexplained renal dysfunction Unilateral reduction in kidney size ESRD without a known cause Unexplained hypokalemia in a hypertensive patient Cardiac Symptoms Recurrent pulmonary edema Recurrent CHF without apparent cause Angina pectoris in the absence of critical coronary artery disease Atherosclerosis Elsewhere Epigastric abdominal or flank bruit Peripheral arterial disease affecting the lower extremities or the carotid arteries Aorto-iliac disease Abdominal aortic aneurysm Severe coronary artery disease |
Although a perfect test to diagnose RAS does not exist, modern imaging tests such as renal duplex ultrasonography, magnetic resonance angiography (MRA), and computed tomographic angiography (CTA) have become quite accurate and sophisticated in detecting the presence of RAS, as well as in assessing the anatomy of the kidney and adjacent structures. Importantly, none of these tests requires discontinuation of patients’ antihypertensive treatment, a major advantage in comparison to older diagnostic modalities such as captopril renography and peripheral plasma renin activity. The choice of which one of the major imaging modalities should be used depends on a variety of factors, including local availability and expertise, as well as cost. Potential advantages and disadvantages of each technique will be described below.
Renal artery duplex combines B-mode imaging with Doppler velocity measurements of blood flow. It provides information on location and degree of RAS, measurement of kidney size, and visualization of possible adjacent processes such as obstruction, masses, or abdominal aortic aneurysms. In addition, an assessment of intrinsic small vessel renovascular disease can be obtained, and a prediction on blood pressure response to revascularization can be made.58 Duplex ultrasonography is the least expensive of the imaging modalities, does not require the use of intravenous contrast, and with improvement in modern hardware and software, can provide visualization of the entire renal artery, including its distal portion. The overall sensitivity and specificity of duplex ultrasonography compared with arteriography are 98% and 98%, respectively.59 Potential disadvantages include prolonged examination time (up to 45–60 minutes), as well as its dependence on operator skills as well as on patient’s body habitus. Duplex ultrasonography can be technically quite challenging, especially in obese patients or in the presence of abdominal gas, hence this diagnostic test is preferentially conducted by experienced technicians in the morning and on fasting patients.
Usually the examination begins in the longitudinal plane, with the patient supine, acquiring velocity data from the celiac trunk and superior mesenteric arteries. The peak systolic velocity in the aorta is obtained just distally to the superior mesenteric artery; this will be used to calculate the renal artery-to-aorta velocity ratio. Using a transverse approach, the examination continues with location of the origin of the renal arteries from the aorta.60 The right renal artery is usually easier to find than the left (Figure 32-3). Since the left renal artery is difficult to follow all the way to the kidney from the anterior approach, the patient can be positioned in the right lateral decubitus, scanning from the left posterolateral approach.61 For the right, the patient can be positioned in the left lateral decubitus.
Kidney size and morphology are evaluated. The peak systolic and end diastolic velocities are then obtained from the proximal, mid and distal renal artery, bilaterally, and from the segmental arteries in the upper and lower poles of the kidneys. Attention should be paid to the possible presence of accessory renal arteries.
Renal arteries as well as segmental and interlobar arteries are characterized by low resistance (Figure 32-4). The renal-to-aortic ratio (RAR) is calculated by dividing the peak systolic velocity of the renal artery by the peak systolic velocity of the aorta. A normal ratio is <3.5. A renal-to-aorta peak systolic velocity ratio ≥3.5 combined with a peak systolic velocity at the lesion of >180 to 200 cm/s is consistent with ≥60% RAS (Figure 32-5). If the end-diastolic velocity is ≥150 cm/s, the stenosis is likely to be ≥80%.59,62 A significant stenosis should be accompanied by poststenotic turbulence and spectral broadening in the abnormal waveforms. Damping of the arterial signal distal to the stenotic lesion, defined numerically by acceleration index or acceleration time, and usually described visually as “pulsus parvus and tardus,” is also consistent with severe RAS (Figure 32-6). When no flow can be detected in the renal artery, low parenchymal Doppler velocities support the diagnosis of an occluded renal artery. In these cases the kidney is usually small (<9 cm in length).59
Since treatment of a stenotic renal artery does not guarantee a positive clinical response, several investigators have sought to identify other duplex criteria to predict a positive outcome after revascularization. The “resistance index” (RI), calculated as RI = (1 – [end-diastolic velocity/peak systolic velocity]) × 100, is being used to evaluate renal parenchymal disease, and has been studied by Radermacher and others in 138 patients undergoing angioplasty or surgery.58 An RI >80 found to identify patients in whom angioplasty or surgery will not improve renal function, blood pressure, or kidney survival. Although this is a potentially useful tool, it can be falsely elevated in patients with decreased cardiac output.
In conclusion, duplex imaging plays an important role in the diagnosis of RAS, but it can be technically demanding and time-consuming, especially in obese individuals or when excessive bowel gas is present. In our practice, we have found this tool very valuable in the follow-up of patients who have undergone renal artery stenting, in whom MRA accuracy maybe hindered by stent artifacts and the administration of intravenous contrast might be undesirable. In these patients, Duplex ultrasound has been in fact shown to be an excellent technique, with high sensitivity and specificity for the diagnosis of in-stent restenosis when a threshold peak systolic velocity ≥226 cm/s and a RAR ≥2.7 are used.63
Recent technologic advancements leading to the development of multidetector computed tomography (MDCT) have resulted in increased speed of image acquisition and high spatial resolution, making CTA a reliable and accurate modality to evaluate renovascular disease.64,65,66
The initial image output is in sets of sequential or overlapping axial images, and postprocessing of volumetric data is required to create three-dimensional angiographic representations.67 Sensitivity and specificity of CTA in the diagnosis of RAS have both been reported to be in excess of 90%,66,68 but it is important that the area of acquisition is extensive enough to detect the presence of accessory renal arteries.67 Furthermore, a study designed to visualize the renal arteries should include imaging of the iliac and femoral arteries in case any revascularization intervention should be needed. Advantages of CTA over catheter-based angiography include the fact that it is not invasive, and that adjacent anatomic structures can also be examined. Compared to MRA, CTA has higher spatial resolution and is less affected by artifacts caused by the presence of calcium or previously implanted metallic stents. CTA is also much less operator-dependent than duplex ultrasonography.
The most obvious disadvantage of CTA, however, is that it requires the administration of nephrotoxic iodinated contrast. Although the rapid acquisition of images allows reduction in contrast use, the typical amount of dye necessary for a CTA of the renal arteries can in fact exceed 100 mL.69,70 Additional limitations include exposure of patients to ionizing radiation, potential difficulties in estimating the degree of stenosis when arterial wall calcification is present, and lengthy postprocedure processing time. Furthermore, CTA, as well as duplex ultrasonography and MRA, is not as accurate as catheter-based angiography in the assessment of RAS secondary to FMD.1
Magnetic resonance arteriography (MRA) of the peripheral arterial circulation was initially performed without the use of contrast, using the so-called 2D time-of-flight technique (Figure 32-7). With this approach, acquisition times were prolonged and motion artifacts resulted in image degradation. The use of the gadolinium-enhanced gradient echo technique has significantly decreased acquisition times (now down to 20–40 seconds) and improved the accuracy of MRA, which, in contrast to CTA, does not require ionizing radiation and can be performed in patients with renal insufficiency, congestive heart failure, and dye allergy. Differently from renal duplex ultrasonography, MRA is less operator-dependent and allows visualization of surrounding anatomical structures as well as of accessory renal arteries.71 Furthermore, MRA may play a role in the physiologic assessment of renovascular disease by determining glomerular filtration rate (gadolinium clearance) and assessing renal perfusion.72,73,74
FIGURE 32-7.
Gadolinium-enhanced magnetic resonance angiogram demonstrating a right renal artery stenosis and a normal left renal artery. This technique allows visualization and measurement of kidney size.
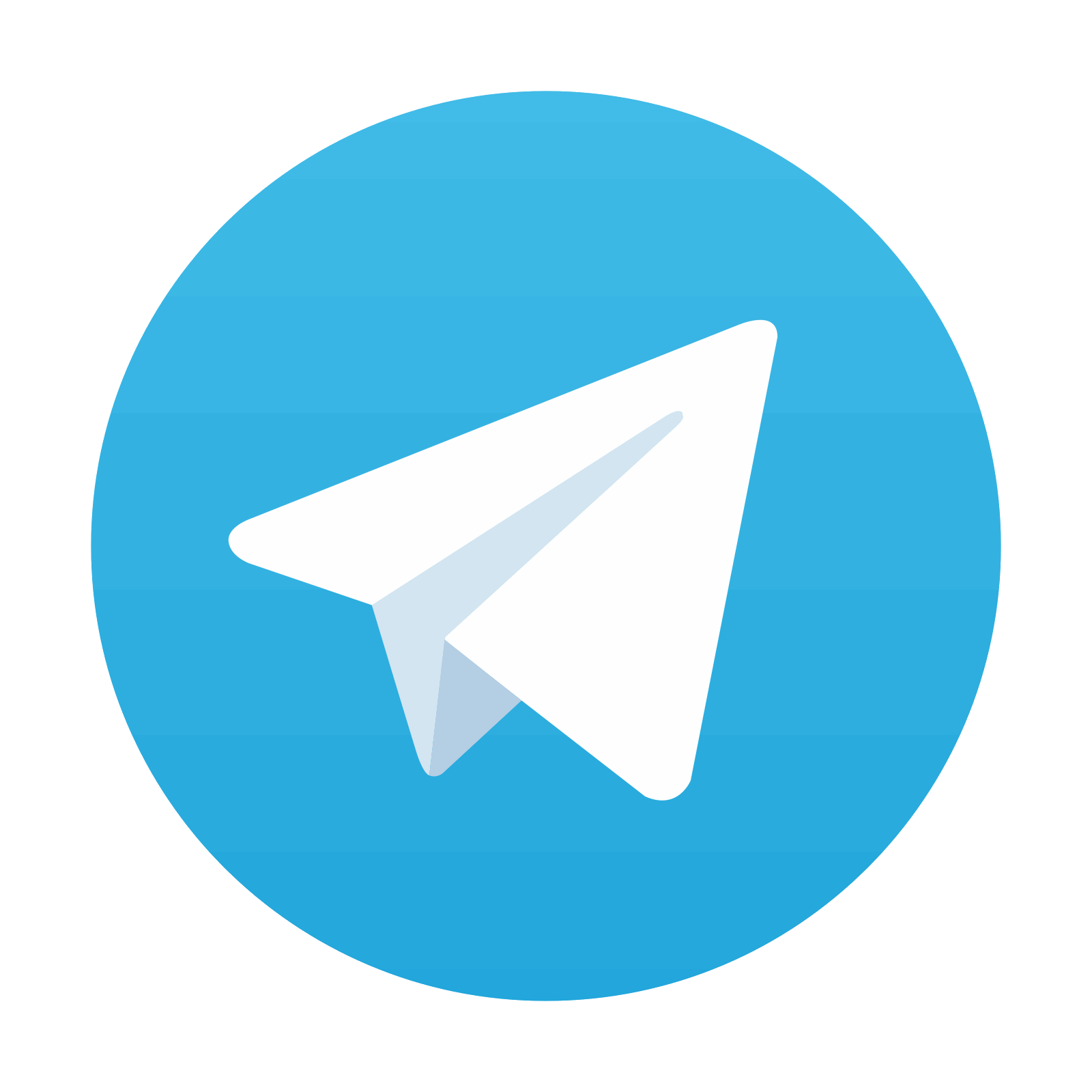
Stay updated, free articles. Join our Telegram channel

Full access? Get Clinical Tree
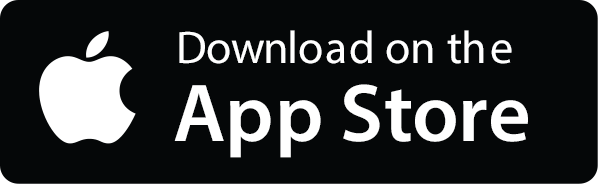
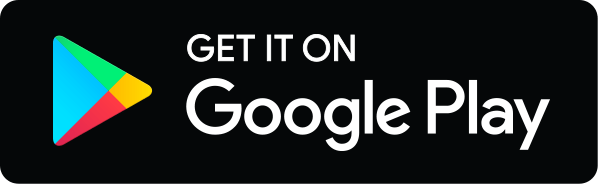
