Renal Artery Disease
Jeffrey W. Olin
Susan M. Begelman
Overview
Renal artery stenosis (RAS) is most commonly due to either fibromuscular dysplasia or atherosclerosis. The former predominates in young women, whereas the latter is almost exclusively seen in individuals older than the age of 55 years. Frequently, RAS is discovered incidentally during imaging studies for other reasons (atrophic or small kidney) or at autopsy. Incidental RAS is quite common (1,2), whereas renovascular hypertension only occurs in 1% to 5% of all patients with hypertension (3). The predominant clinical manifestation of fibromuscular dysplasia is hypertension. The hypertension can frequently be cured or significantly improved with percutaneous balloon angioplasty. The predominant clinical manifestations of atherosclerotic RAS include hypertension, renal failure (ischemic nephropathy), and recurrent episodes of congestive heart failure and flash pulmonary edema (4,5).
The presence of anatomic RAS does not necessarily establish that the hypertension or renal failure is caused by the RAS. Many patients have essential (primary) hypertension for years and then develop atherosclerotic RAS later in life. In patients with long-standing hypertension, it is unlikely that a cure of the hypertension can be achieved with surgical or percutaneous intervention. However, 50% to 80% of patients do experience improvement in blood pressure control (6,7,8,9,10,11,12). Ischemic nephropathy or flash pulmonary edema only occurs in the presence of bilateral renal artery disease or disease to a solitary functioning kidney. Percutaneous or surgical revascularization can lead to improvement or stabilization in renal function and improvement in heart failure if the patients are carefully selected (9,13,14,15).
Screening tests for RAS have improved considerably over the last decade. Whereas captopril renography was used almost exclusively in the past, direct imaging with duplex ultrasound, magnetic resonance angiography, or multidetector computed tomography (CT) angiography has replaced other modalities as the screening test of choice in most centers. Rarely does an arteriogram have to be performed for diagnostic purposes only.
Management of RAS consists of three strategies: medical management, surgical revascularization or percutaneous therapy with balloon angioplasty, and stent implantation. The treatment of choice for controlling hypertension in patients with fibromuscular disease is percutaneous angioplasty. Renal artery stenting has replaced surgical revascularization for virtually all patients with atherosclerotic disease who require a revascularization procedure.
Historical Perspective
In 1934, Goldblatt and colleagues (16) demonstrated that hypertension could be produced in dogs by constriction of one renal artery (two-kidney, one-clip model) or both renal arteries or one renal artery if the contralateral kidney was removed (one-kidney, one-clip model). Irving Page later demonstrated that renin produced a pressor substance, which he initially called
angiotonin and later named angiotensin, which was subsequently isolated by Skeggs in 1954 (17,18).
angiotonin and later named angiotensin, which was subsequently isolated by Skeggs in 1954 (17,18).
Pathophysiology
Experimental Models of Renovascular Hypertension
Pickering clarified the difference between renovascular hypertension and renal artery stenosis:
The demonstration of a RAS in a hypertensive patient does not necessarily establish a diagnosis of renovascular hypertension, because essential hypertension may accelerate the development of atheromatous plaques, which do not necessarily have any functional significance. Ideally, it is necessary to demonstrate that there is also renal ischemia, since this is thought to be the stimulus that raises the blood pressure, and leads to a decline of renal function (19).
Most investigators believe that there must be at least a 70% reduction in luminal diameter for a RAS to cause either hypertension or ischemic nephropathy (4,20,21).
Animal models for renovascular hypertension have helped to elucidate the pathophysiology of hypertension in patients with RAS (22,23,24,25). The renin–angiotensin–aldosterone system plays an important role, as shown in Figure 109.1 (26). In animals, the two-kidney, one-clip (2K-1C) model is the classic model for renin-mediated hypertension and is analogous to unilateral RAS in humans. The one-kidney, one-clip (1K-1C) model of renovascular hypertension is a model for volume-mediated hypertension and is analogous to bilateral RAS or RAS to a solitary functioning kidney in humans. Although the acute phases of both of these models are similar, different events occur in the chronic phase.
In the 2K-1C model (unilateral RAS), decreased renal blood flow stimulates the production of renin (Fig. 109.1A). Renin cleaves the proenzyme angiotensinogen to form angiotensin I and in the presence of angiotensin-converting enzyme is converted to angiotensin II. Angiotensin II has several important functions: (a) It elevates blood pressure directly by causing systemic vasoconstriction, (b) it stimulates aldosterone secretion, causing sodium reabsorption and potassium and hydrogen ion secretion in the cortical collecting duct, and (c) it changes the intrarenal hemodynamics, such as diminishing glomerular filtration, by decreasing glomerular capillary surface area and redistributing intrarenal blood flow. The salt and water retained (caused by excess aldosterone production) is rapidly excreted by the contralateral (normal) kidney by pressure natruresis. This produces a cycle of renin-dependent hypertension (23,27).
Administration of an angiotensin-converting-enzyme inhibitor (ACEI) blocks this cycle and, at least early in the course of renovascular hypertension, returns the blood pressure to normal. In the chronic phase the blood pressure remains elevated even though the plasma renin activity returns to baseline. When an ACEI or angiotensin-receptor blocker (ARB) is administered, the blood pressure does not immediately decline, but takes 5 to 7 days (28) to return to normal. The delayed blood pressure response suggests that the change in blood pressure is no longer primarily caused by the direct effects of angiotensin II, but involves some degree of salt retention as well.
In the 1K-1C model of renovascular hypertension, there is a similar decrease in blood flow to the affected kidney(s), acutely causing the secretion of renin and synthesis of angiotensin II and aldosterone (Fig. 109.1B) (26). Angiotensin II directly elevates blood pressure, and aldosterone causes salt and water retention. In this model there is not a normal kidney that can sense the elevated blood pressure, and therefore pressure natruresis does not occur. The increased aldosterone causes sodium and water retention and volume expansion. The expanded plasma volume suppresses plasma renin activity, thus converting the animal from renin-mediated hypertension to volume-mediated hypertension (29). During this stage, administration of an ACEI or ARB does not decrease blood pressure or change renal blood flow (30). Dietary restriction of sodium or administration of diuretics will return the subject to a renin-mediated form of hypertension and restore sensitivity to an ACEI or ARB. Functional renal insufficiency may occur in humans when ACEIs are administered to patients with bilateral RAS or RAS to a solitary kidney especially in the volume-contracted state (Fig. 109.2).
An important clinical concept is that long-standing hypertension may cause changes in intrarenal hemodynamics and structural changes in the microvasculature of the kidney leading to nephrosclerosis (30). Thus, if a patient with
nephrosclerosis later develops unilateral RAS, he or she may behave like a patient with bilateral disease because the contralateral kidney would no longer be able to excrete a salt load normally.
nephrosclerosis later develops unilateral RAS, he or she may behave like a patient with bilateral disease because the contralateral kidney would no longer be able to excrete a salt load normally.
Pathophysiology of Ischemic Nephropathy
Patients who develop azotemia while receiving angiotensin-converting-enzyme inhibitors or angiotensin II–receptor blocking agents often have bilateral RAS, RAS to a solitary kidney, or decompensated congestive heart failure in the sodium-depleted state (13,21,31,32,33,34).
There are two mechanisms by which renal functional impairment may occur with the use of antihypertensive agents. The first may occur with any antihypertensive agent when a critical perfusion pressure is reached below which the kidney no longer receives adequate perfusion. This has been shown by the infusion of sodium nitroprusside in patients with high-grade bilateral RAS. When the critical perfusion pressure was reached, the urine output, renal blood flow, and glomerular filtration rate declined, and they returned to normal when the blood pressure increased above this critical perfusion pressure (35). The exact pressure necessary to perfuse a kidney with RAS varies with the degree of stenosis and is different among different patients.
The second mechanism is confined to patients receiving ACEI or ARB agents and may occur despite no significant change in blood pressure (32). Patients with severe bilateral RAS or RAS to a solitary kidney may be highly dependent on angiotensin II for glomerular filtration. This is particularly common in patients who receive a combination of ACE inhibitor and diuretic (36) or in patients who are placed on a sodium-restricted diet (37). Under these circumstances, the constrictive effect of angiotensin II on the efferent arteriole allows for the maintenance of normal transglomerular capillary hydraulic pressure, thus allowing glomerular filtration to remain normal in the presence of markedly diminished blood flow (Fig. 109.2). In this instance glomerular filtration is highly dependent on angiotensin II. When an ACEI is administered, the efferent arteriolar tone is no longer maintained and glomerular filtration is therefore decreased. A similar situation occurs in patients with decompensated congestive heart failure who are sodium depleted (34,37).
Pathology of Renovascular Disease
Shanley suggested that loss of renal mass, loss of nephrons, and renal atrophy predominate in the pathologic picture of patients with renovascular disease (38). There is a point at which many of these changes are reversible, and the goal of management is to identify the patients early and treat them to prevent progressive renal failure. Atheromatous embolization has also been associated with renovascular disease (39). The compilation of injury leads to cortical scarring, interstitial fibrosis, tubular collapse, and destruction of the renal architecture.
Systemic and glomerular pressures play important roles in renal injury in patients with renovascular disease. In the two-kidney, one-clip model of renovascular hypertension, the unclipped kidney demonstrates significantly more vascular and glomerular damage than the clipped kidney (40). It is not clear whether this is due to a direct hemodynamic effect of the elevated blood pressure or to the mitogenic and hypertrophic effects of angiotensin.
Anatomic Considerations
Atheromatous Lesions
More than 90% of all renovascular lesions are secondary to atherosclerosis (4). Although atherosclerotic RAS may occasionally be isolated to the renal artery alone, it is more commonly a manifestation of systemic atherosclerosis involving the aorta and coronary, cerebral, and peripheral vessels. Atherosclerotic RAS most often occurs at the ostium or the proximal 2 cm of the renal artery (Fig. 109.3). Distal arterial or branch involvement is distinctly uncommon (26).
Fibromuscular Dysplasia
Fibromuscular dysplasia (FMD) is a nonatherosclerotic, noninflammatory disease that most commonly affects the renal arteries and is the second-most-common cause of RAS (41). The most common clinical presentation is that of hypertension in a young woman. Fibromuscular dysplasia has been demonstrated in virtually every vascular bed. Renal artery involvement occurs in 60% to 75% of patients with FMD, followed by extracranial cerebrovascular arteries in 25% to 30%, visceral arteries in 9%, and arteries of the extremities in approximately 5% of patients (41,42). It may present as a systemic disease (affecting a combination of the carotids, mesenteric, subclavian, and/or extremity vessels) in up to 28% of patients (43). The diagnosis is rarely made pathologically but is usually determined by its typical angiographic appearance (Fig. 109.4). Whereas atherosclerosis involves the origin and proximal portion of the renal arteries, FMD characteristically involves the distal two thirds of the artery and may involve the branches (41,44).
The lesions of FMD are thought to be congenital dysplasias with maldevelopment of the fibrous, muscular, and elastic tissues of the renal artery. They are subcategorized according to the layer of the arterial wall involved (45,46). This classification is important because each type of fibrous dysplasia has distinct histologic and angiographic features and each type occurs in a different clinical setting (Table 109.1).
Medial fibromuscular dysplasia has been further divided into medial hyperplasia (the only type in which there is true smooth muscle hyperplasia), perimedial dysplasia, and medial fibroplasia. Medial fibroplasia is the histologic finding in nearly 80% of all cases of FMD. It tends to occur in 25- to 50-year-old women and often involves both renal arteries. It has a “string of beads” appearance angiographically, with the “bead” diameter larger than the proximal, unaffected artery (Fig. 109.4). The areas of stenosis are often overshadowed by contrast medium in the microaneurysms, making the degree of actual stenosis difficult to assess. This beading is due to thickening of the media, interspersed by areas of aneurysmal dilation. Microscopically, the internal elastic membrane is focally variably thinned and lost. Within the alternating thickened areas much of the muscle is replaced by collagen, hence the term medial fibroplasia. In other areas, thinning of the media occurs to the point of complete loss, and microaneurysms can be seen as saccules lined by only
the external elastica. In extreme cases, giant aneurysms may be found in association with medial fibroplasia (Fig. 109.5). Progression to total occlusion is rare in this subtype. Medial fibroplasia responds very well to percutaneous balloon angioplasty. The other types of FMD are beyond the scope of this chapter and are nicely summarized in several recent reviews (41,46).
the external elastica. In extreme cases, giant aneurysms may be found in association with medial fibroplasia (Fig. 109.5). Progression to total occlusion is rare in this subtype. Medial fibroplasia responds very well to percutaneous balloon angioplasty. The other types of FMD are beyond the scope of this chapter and are nicely summarized in several recent reviews (41,46).
TABLE 109.1 Classification of Fibromuscular Dysplasia | ||||||||||||||||||||||||||||||||
---|---|---|---|---|---|---|---|---|---|---|---|---|---|---|---|---|---|---|---|---|---|---|---|---|---|---|---|---|---|---|---|---|
|
Miscellaneous Causes of Renovascular Disease
Other causes of renovascular disease include aneurysm, Takayasu arteritis, atheroemboli, thromboemboli, spontaneous renal artery dissection, arteriovenous malformations or fistulas, trauma (such as from radiation, lithotripsy, direct injury, or surgery), and neurofibromatosis. Retroperitoneal fibrosis producing external compression has also been associated with RAS.
Clinical Aspects of Renal Artery Stenosis
Primary or essential hypertension is present in more than 60 million Americans. Renovascular disease and renal parenchymal disease are the most common secondary causes of hypertension after obesity, excess alcohol ingestion, drug abuse, and oral contraceptives are excluded. Patients with atherosclerotic RAS have the usual risk factors for atherosclerosis. Whereas the effect of atherosclerosis on the coronary and carotid arteries is well recognized, involvement of the renal arteries is frequently overlooked. Because patients with atherosclerotic RAS succumb prematurely from myocardial infarction and stroke (8,47,48,49,50,51), early diagnosis and treatment is of vital importance to avoid premature morbidity and mortality.
Prevalence of Renal Artery Stenosis
Although there are excellent prevalence data in specific patient populations (e.g., those with coronary artery disease, aortic aneurysm, or peripheral arterial disease), the prevalence of RAS in the general population is not known. Not all cases of RAS are clinically significant. Dustan et al. (51) reviewed 149 aortograms and found that approximately half of patients with 50% or greater RAS did not have hypertension.
In a recent population-based study, Hansen and colleagues reported on the prevalence of renovascular disease in a cohort of elderly patients (52). Eight hundred thirty-four participants of the Cardiovascular Health Study underwent renal duplex ultrasound. Fifty-seven (6.8%) had anatomic renal artery stenosis. There was no difference in the prevalence of RAS in whites (6.9%) and African Americans (6.7%).
Several series have looked at the prevalence of renovascular disease in patients who have atherosclerotic disease elsewhere. To determine the prevalence of atherosclerotic RAS, we studied 395 consecutive patients who had undergone arteriography as part of an evaluation for an abdominal aortic aneurysm, aortoiliac occlusive disease, and peripheral arterial disease. In addition, 78 patients had an aortogram performed for suspected renal artery stenosis (Table 109.2) (1). These patients did not have the usual clinical clues to suggest RAS. High-grade bilateral renal artery disease was present in approximately 13% of patients. In the 319 patients reported in six different studies, 44% had bilateral RAS (9). Other studies showed that 22% to 59% of patients with peripheral arterial disease have significant renal artery stenosis (53).
It has also been established that RAS is common in patients with coronary artery disease. Of 7,758 patients undergoing cardiac catheterization during a 76-month period, 3,987 underwent aortography to screen for RAS at the time of catheterization (54). One hundred ninety-one (4.8%) patients had greater than 75% renal artery stenosis and 0.8% had severe bilateral disease. In the Mayo Clinic series (55), renal arteries were studied at the time of cardiac catheterization in patients with hypertension. Ninety percent of the renal arteries were adequately visualized, and no complications occurred from the aortogram. Greater than 50% RAS was present in 19.2% of patients, greater than 70% in 7%, and bilateral RAS in 3.7%.
Natural History of Renal Artery Stenosis
Knowledge of the natural history is extremely important in the subsequent management of patients with RAS. Most natural history studies reported in the literature were retrospective studies. The rates of progression ranged from 36% to 71% (56). In Schreiber et al.’s series, only 16% of patients progressed to total occlusion over a mean follow-up of 52 months. However, the rate of progression to total occlusion occurred more frequently (39%) when there was greater than 75% stenosis on the initial renal arteriogram (56).
Zierler et al. (57,58) used renal duplex ultrasound to prospectively study anatomic progression of atherosclerotic renovascular disease. If the renal arteries were normal, only 8% progressed over 36 months. However, at 3 years, 48% of patients progressed from less than 60% stenosis to 60% or greater stenosis. All four renal arteries that progressed to occlusion had 60% or greater stenosis at the initial visit. Progression
of RAS occurred at an average rate of 7%/year for all categories of baseline disease combined.
of RAS occurred at an average rate of 7%/year for all categories of baseline disease combined.
TABLE 109.2 Prevalence of Atherosclerotic Renal Artery Stenosis | |||||||||||||||||||||||||
---|---|---|---|---|---|---|---|---|---|---|---|---|---|---|---|---|---|---|---|---|---|---|---|---|---|
|
The effect of RAS on kidney size has been well studied (59,60). Using duplex ultrasound, Caps and colleagues prospectively followed 204 kidneys in 122 patients with known renal artery stenosis for a mean of 33 months. The 2-year cumulative incidence of renal atrophy was 5.5%, 11.7%, and 20.8% in kidneys with a baseline renal artery disease classification of normal, less than 60% stenosis, and 60% or greater stenosis, respectively (p = .009, log rank test) (59).
A question that has really never been satisfactorily answered, however, is how common is atherosclerotic RAS as a cause of end-stage renal disease (ESRD)? Scoble et al. (61) found that atherosclerotic renovascular disease was the cause of ESRD in 14% of patients starting dialysis therapy. Mailloux and colleagues (50) reviewed the causes of ESRD in 683 patients over a 20-year period. Eighty-three patients (12%) had documented RAS as a cause of ESRD. Because these investigators only performed arteriography in patients in whom they highly suspected RAS, it is possible that the true incidence of RAS as a cause of ESRD was seriously underestimated. A recent study reported that 16% of 49 patients starting renal replacement therapy had greater than 50% bilateral RAS or RAS to a single functioning kidney (62). Renal artery stenosis should be searched for in every patient starting dialysis if a clear-cut etiology for the ESRD is not known (63,64,65).
Connolly et al. (48) showed that the 2-year actuarial renal survival (percentage of patients remaining off dialysis) was 97.3% for patients with unilateral RAS, 82.4% for patients with bilateral RAS, and 44.7% for patients with stenosis or occlusion to a solitary functioning kidney. Patients on dialysis have a shortened life expectancy. The average life expectancy in a patient with ESRD older than age 65 years is only 2.7 years (66,67). The survival estimates are even worse if the patient has atherosclerotic renovascular disease as the cause of ESRD. Mailloux and associates (50) showed that median survival for patients with renovascular disease was 25 months compared to 55 months in patients with malignant hypertension and 133 months for patients with polycystic kidney disease. The 2-year survival on dialysis in patients with renovascular disease was 56%, 5-year survival was 18%, and 10-year survival was only 5%. These data underscore the fact that patients with atherosclerotic RAS who progress to ESRD and require dialysis have extremely high mortality rates.
Survival in Renal Artery Stenosis
In addition, the mere presence of RAS, even prior to developing ESRD, portends a poor prognosis. Patient survival decreases as the severity of RAS increases (54), with a 2-year survival rates of 96% in patients with unilateral RAS, 74% in patients with bilateral RAS, and 47% in patients with stenosis or occlusion to a solitary functioning kidney (48). Dorros and associates (8) demonstrated that as the serum creatinine increases, the survival decreases in patients with atherosclerotic RAS. The 3-year probability of survival was 92% ± 4% for patients with a serum creatinine less than 1.4 mg/dL, 74% ± 8% for patients with a serum creatinine of 1.5 to 1.9 mg/dL, and 51% ± 8% for patients with a serum creatinine 2.0 mg/dL or greater.
Long-term survival was investigated at Duke University in a cohort of 3,987 patients who underwent aortography at the time of cardiac catheterization. In patients with 75% or greater stenosis of at least one renal artery, the 4-year survival rate was 57% compared to a 4-year survival rate of 89% in patients with less than 75% stenosis. The effect of renal artery stenosis on survival remained robust regardless of how the coronary artery disease was treated (medical, percutaneous transluminal coronary angioplasty, or coronary artery bypass grafts) (54).
Clinical Clues to the Diagnosis of Renal Artery Stenosis
Hypertension
What was the age of onset?
Has the hypertension suddenly become more difficult to control?
Did the patient have malignant or accelerated hypertension?
Does the patient have resistant hypertension?
Individuals who develop hypertension between the ages of 30 and 55 years usually have primary (essential) hypertension. If the initial diagnosis of hypertension is made before the age of 30 years, it is usually due to fibromuscular dysplasia. Because atherosclerosis occurs in older individuals, it is usually the cause of RAS after the age of 55 years. It is not uncommon for patients to have years of primary hypertension and, as they age, develop atherosclerotic RAS. This cohort of patients may have had well-controlled blood pressure that suddenly became more difficult to control. Accelerated or malignant hypertension has also been associated with a very high prevalence of RAS. Resistant hypertension is defined as failure to reach goal blood pressure in patients who are adhering to full doses of an
appropriate three-drug regimen that includes a diuretic (68). The diagnosis of renovascular disease should be strongly considered in patients with resistant hypertension.
appropriate three-drug regimen that includes a diuretic (68). The diagnosis of renovascular disease should be strongly considered in patients with resistant hypertension.
TABLE 109.3 Clinical Clues to the Diagnosis of Renal Artery Stenosis | |
---|---|
|
Physical Examination
Does the patient have evidence of atherosclerosis elsewhere?
Does the patient have an epigastric bruit?
In general, the physical exam is of limited help. Evidence of coronary, cerebral, or peripheral arterial disease is associated with a higher likelihood of renal artery disease due to the systemic nature of atherosclerosis. Although a systolic abdominal bruit is common and nonspecific, the presence of a systolic/diastolic bruit especially over the epigastrium may point to underlying renal artery disease. The presence of a diastolic component to the bruit indicates that the degree of narrowing of the artery is severe because there is continued flow during diastole (69). A systolic/diastolic bruit more often occurs in patients with fibromuscular disease (53%) than in patients who have atherosclerotic disease (12.5%) (70). An abdominal bruit in a young, trim person with no hypertension may be due to compression of the celiac artery by the median arcuate ligament and is often of no clinical significance. The presence of a bruit is helpful, but the absence does not exclude the diagnosis of either atherosclerotic renovascular disease or fibromuscular dysplasia.
Renal Function
Are the kidneys of differing sizes?
Has the patient ever developed azotemia associated with angiotensin-converting-enzyme inhibitors or angiotensin II–receptor blocking agents?
Does the patient have unexplained azotemia?
Is the patient receiving dialysis without a clear cause for end-stage renal disease?
Gifford et al. (71) found that 71% of patients (53 of 75) with an atrophic kidney had severe stenosis or complete occlusion of the renal artery ipsilateral to the small kidney. Several studies have shown that if there is a discrepancy in the size between the two kidneys or if one kidney is atrophic, there is a 60% chance that the contralateral renal artery (normal-sized kidney) is severely stenotic (1,71,72). Therefore, the presence of an atrophic kidney or a discrepancy in size between the two kidneys demands a thorough investigation for the presence of renovascular disease.
There are numerous reports suggesting that patients who develop azotemia while receiving ACE inhibitors have bilateral RAS, RAS to a solitary kidney, or decompensated congestive heart failure in the sodium-depleted state (21,32,33,34,35,73,74). The mechanisms were discussed in detail earlier in this chapter.
Congestive Heart Failure and Pulmonary Edema
Does the patient have unexplained congestive heart failure?
Has the patient had recurrent episodes of flash pulmonary edema?
Recurrent congestive heart failure and “flash” pulmonary edema not related to ischemic heart disease can result from bilateral RAS or unilateral RAS to a single functioning kidney. In our renal artery stent series, 39 patients (19% of all patients undergoing renal artery stent implantation from 1991 to 1997) had recurrent episodes of congestive heart failure or “flash” pulmonary edema as the primary indication for renal artery stenting (13). Although it is not completely understood, the mechanism of congestive heart failure may be related in part to the inability to use ACEIs or ARBs, direct toxic effects of angiotensin II on myocardial function or volume expansion, and the inability to achieve adequate diuresis (13,75).
Diagnosis of Renovascular Disease
The ideal imaging procedure should (a) identify the main renal arteries as well as accessory or polar vessels, (b) localize the site of stenosis or disease, (c) provide evidence for the hemodynamic significance of the lesion, and (d) identify associated pathology (i.e., abdominal aortic aneurysm, renal mass, etc.) that may affect the treatment of the renal artery disease (72,76,77). The most sensitive and specific methods of identifying RAS include the noninvasive imaging techniques such as Duplex ultrasound, CT angiography, and magnetic resonance angiography (MRA).
Arteriography
Angiography, the gold standard for arterial imaging, is rarely required to make the diagnosis of RAS. Usually one or more of the noninvasive modalities can accurately make the diagnosis. Exceptions to this general rule may occur in patients with fibromuscular dysplasia that primarily affects the branches of the renal arteries and in patients with renal artery aneurysms (41). Angiography or intraarterial digital subtraction angiography (IA-DSA) has several distinct advantages as an imaging modality. All portions of the renal artery including the intrarenal branches can be well visualized. In addition, accessory renal arteries can be identified and one can determine kidney size and gross function (the presence of a nephrogram). However, angiography is invasive and costly compared to other imaging modalities. Although they are not common, complications associated with angiography include access-site complications such as a hematoma, pseudoaneurysm, or arterial venous fistula, as well as acute renal failure from contrast-induced nephrotoxicity and atheromatous embolization to the kidneys, bowel, or lower extremities. Therefore, angiography is a poor screening test for RAS but an excellent confirmatory test once it has been decided that revascularization is indicated.
Renal Arteriography at the Time of Cardiac Catheterization
A hotly debated and still controversial issue is the performance of renal angiography at the time of cardiac catheterization. Some cardiologists do it routinely on all patients, whereas others selectively image the renal arteries in patients who have clinical clues that suggest the presence of renal artery stenosis. In the Duke series in which 3,987 patients were screened with abdominal aortography at the time of cardiac catheterization, only 191 (4.8%) of patients had greater than 75% renal artery stenosis and only 33 (0.8%) had severe bilateral disease (54). Similarly, the Mayo Clinic (55) prospectively evaluated 297 hypertensive patients at the time of cardiac catheterization and only 19% had renal artery stenosis greater than 50%, 7% had stenosis greater than 70%, and 3.7% had bilateral disease. They did, however, demonstrate that evaluation of the renal arteries can be completed safely with the addition of only 62 cc of contrast. Therefore, although it is safe to perform angiography at the time of catheterization, the yield is low, and there is some evidence that knowing that there is renal artery stenosis present will tempt the angiographer to stent the renal artery in the absence of clear-cut indications (81,82). Therefore, we are opposed to routine “drive-by angiography” at the time of cardiac catheterization because it adds nothing to the overall management of the patient other than to tempt the angiographer to perform a procedure that is not indicated. However, if the patient has a clear-cut indication for intervention (inability to control the blood pressure with a good antihypertensive regimen, jeopardy of renal function, or recurrent episodes of congestive heart failure) and the clinician is prepared to perform angioplasty and stenting should significant RAS be discovered, then an aortogram at the time of cardiac catheterization should be performed.
Direct Screening Tests
Evaluation of the renal arteries is essential in acute or chronic renal failure, poorly controlled hypertension, or recurrent congestive heart failure that is not directly caused by myocardial ischemia. Imaging modalities are also useful for screening for restenosis after percutaneous or surgical revascularization of the renal arteries. Every patient who has undergone percutaneous intervention for RAS should be put in a surveillance program for detecting restenosis (5,77). Technological advances in computed tomographic angiography (CTA) and MRA provide aortic and renal artery imaging that compares well to the quality and accuracy of angiography and have virtually replaced catheter-based angiography for the evaluation of aortic, renal, and peripheral arterial disease (20). Factors such as the patient’s body habitus, the degree of renal impairment, and cost may help to determine which screening test is used. However, institutional experience and expertise are perhaps the most important factors determining which of the noninvasive imaging studies is used as a screening test for renovascular disease.
Duplex Ultrasonography
Duplex ultrasonography is an excellent test for detecting RAS. It is the least expensive of the imaging modalities and provides useful information about the degree of stenosis, the kidney size, and other associated disease processes such as obstruction, and it may even predict which patients can expect an improvement in blood pressure control or renal function after renal artery angioplasty and stenting (83,84). The location and degree of stenosis can accurately be determined by duplex ultrasound of the renal artery. Duplex ultrasound can be performed without altering the antihypertensive regimen and does not require contrast agents that may have an adverse affect on renal function.
Duplex ultrasonography combines B-mode ultrasound and Doppler examination. Improvements in ultrasound hardware, software, and transducer technology are responsible for improved visualization of the arteries and more precise Doppler interrogation of the entire artery. Renal artery ultrasound should be performed from both an anterior and an oblique [or posterior (flank)] approach. In the longitudinal (long-axis) view, the peak systolic flow velocity in the aorta is recorded at the level of the renal arteries. The aortic velocity and the highest renal artery peak systolic velocity are used to calculate the renal aortic ratio.
The renal arteries are best visualized in a transverse (short-axis) view. Figure 109.6 demonstrates a high-grade stenosis of the left renal artery before and after renal artery stent implantation. With the B-mode image and a 60-degree angle of insonance the arteries are interrogated with pulse-wave Doppler. The Doppler should be swept through the artery from its origin to the renal hilum. This allows the examiner to survey the artery for velocity shifts along the entire course of the renal artery. Velocities should be recorded at the origin, proximal, mid, and distal arterial segments. From an oblique approach the renal artery can be visualized at the renal hilum and followed to the aorta. Because medial fibroplasia most often occurs in the mid to distal renal artery, the oblique approach is particularly good for identifying these entities (72,85).
Overall, when compared to angiography, duplex ultrasound has a sensitivity and specificity of 84% to 98% and 62% to 99%, respectively, when used to diagnose RAS (86,87,88,89,90,91). We performed a prospective study comparing duplex ultrasound to angiography (Table 109.4) (91).
The renal aortic ratio (RAR), the ratio of the peak systolic velocity (PSV) in the renal arteries to the PSV in the aorta, is used to classify the degree of stenosis. A three-category classification scheme is commonly used: 0% to 59% stenosis, 60% to 99% stenosis, and total occlusion (Table 109.5).
The resistive index (RI) is calculated as (peak systolic velocity–end-diastolic velocity)/peak systolic velocity and is a measure of the resistance within the renal circulation (84,92
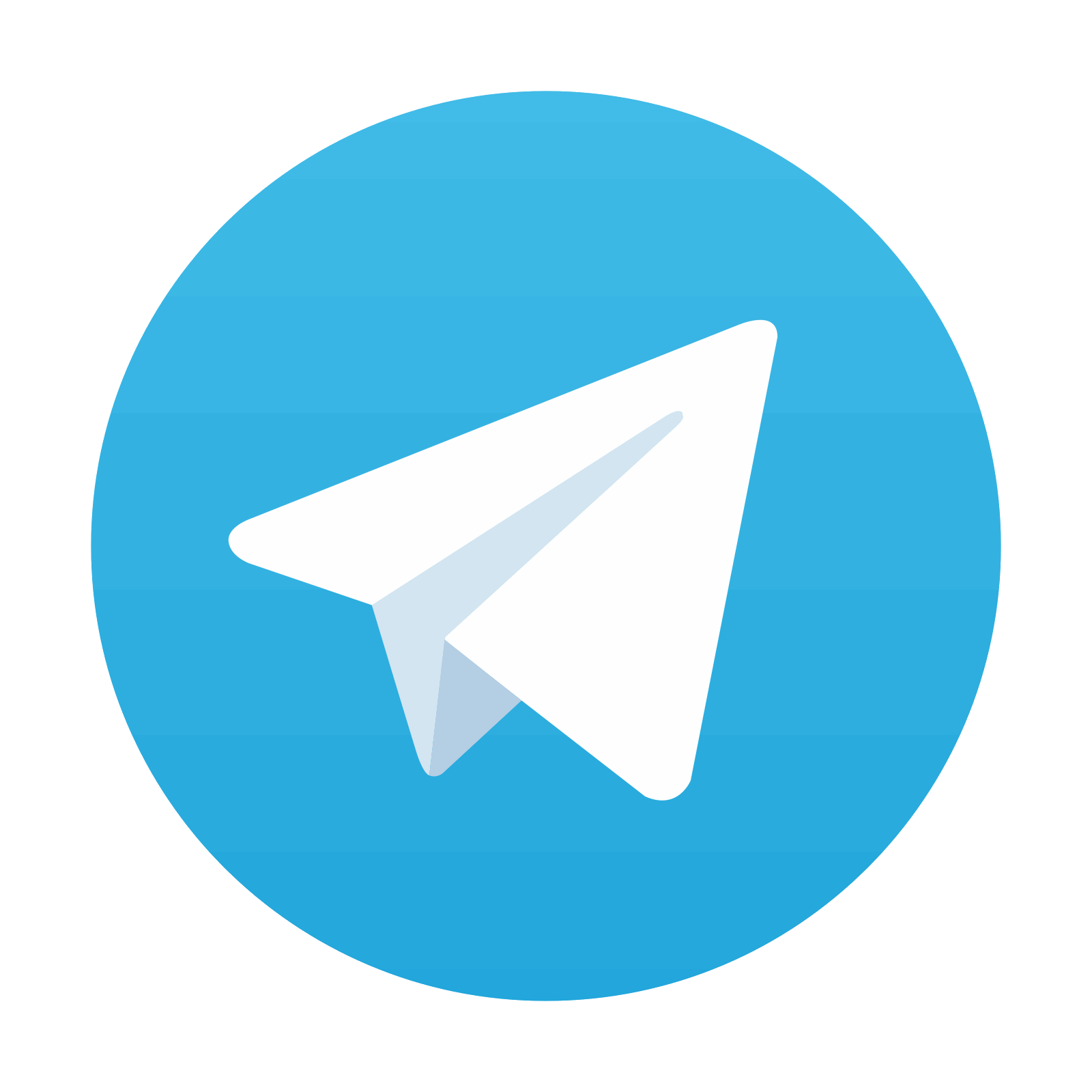
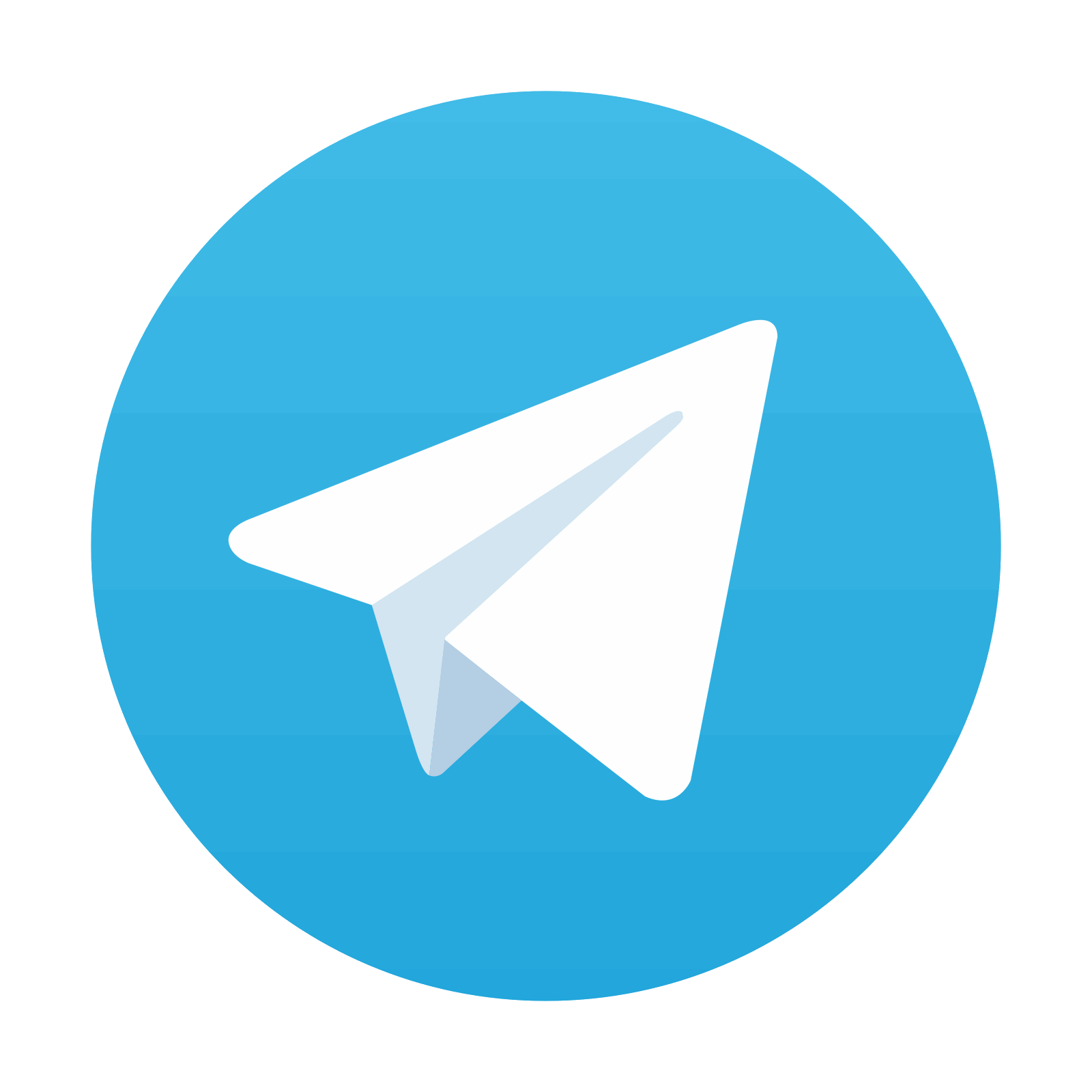
Stay updated, free articles. Join our Telegram channel

Full access? Get Clinical Tree
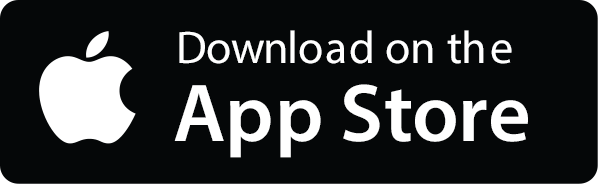
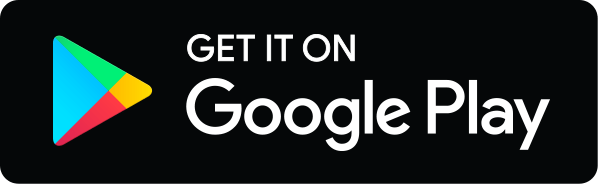
