Background
Cardiotoxicity is a well-known adverse effect of various chemotherapeutic agents that can be monitored by echocardiography. A decrease in left ventricular ejection fraction (LVEF) triggers consideration for therapy modification or interruption. The aim of this study was to evaluate how variability in LVEF estimates computed using three-dimensional echocardiography could influence cardiotoxicity onset detection.
Methods
One hundred eighty one patients with breast cancer treated with anthracycline and trastuzumab were analyzed. LVEF was computed using two commercial software packages. In a subgroup of 40 patients, three-dimensional echocardiographic data were reanalyzed to assess intra- and interobserver variability by two expert investigators using both packages. Global longitudinal strain (GLS) imaging was evaluated in 64 patients.
Results
End-diastolic volume, end-systolic volume, and LVEF measurements obtained applying the two software packages were in good agreement, with small bias and acceptable limits of agreement. Intra- and interobserver variability was smaller using one of the two software packages. However, for both packages, variability indexes were in the range of affecting LVEF estimates at a level that could lead to an inaccurate assessment of cardiac adverse effects of cancer therapeutic drugs. On the basis of LVEF, 11 of 181 patients (6.1%) had cardiotoxicity at 3-month follow-up. The absolute value of GLS was smaller in 16 of 64 patients (25%) thought to have cardiotoxicity on the basis of GLS results, including six of seven patients who had cardiotoxicity considering LVEF in this subgroup.
Conclusions
Following clinical definition of cardiotoxicity onset, variability in LVEF computation by three-dimensional echocardiography could be a confounding factor for cardiotoxicity diagnosis, and different software packages should not be used interchangeably for LVEF monitoring. GLS confirms its predictive value for subsequent cardiotoxicity.
Highlights
- •
The exclusive use of the LVEF index seems inappropriate for cardiotoxicity onset assessment.
- •
Variability in LVEF computation is a confounding factor for cardiotoxicity monitoring.
- •
Different software packages should not be used interchangeably for LVEF monitoring.
- •
GLS should be integrated with LVEF monitoring during and after chemotherapy administration.
In patients with breast cancer, cardiotoxic effects are well described in reference to several chemotherapeutic agents, including anthracyclines and trastuzumab, and several studies have revealed an incidence of dose-dependent myocardial injuries up to 18%. The definition of cardiotoxicity is under debate; Mor-Avi and Lang defined it as a reduction of the left ventricular ejection fraction (LVEF) of >5% to <55% with symptoms of heart failure or asymptomatic reduction of the LVEF of >10% to ≤55%. More recently the expert consensus statement for multimodality imaging evaluation of adult patients during and after cancer therapy from the American Society of Echocardiography and the European Society of Cardiovascular Imaging defined cardiotoxicity as a decrease in the LVEF of >10% to a value <53% as measured by two-dimensional (2D) echocardiography. As such, assessment of resting LVEF before chemotherapy administration and its monitoring during chemotherapy is recommended by both oncology and cardiology societies, and once LVEF decrease is confirmed 2 or 3 weeks after the baseline diagnostic study, chemotherapy modification or interruption should be taken into consideration. Consequently, LVEF estimation is crucial in diagnostic and therapeutic decision making in oncology patients.
In clinical practice, ultrasound imaging is the standard diagnostic and screening technique of choice for cardiac function assessment. Cardiac magnetic resonance imaging is also appropriate, but the guidelines suggest the use of echocardiography because it is the most readily available modality to screen for cardiotoxicity by means of serial measurements of LVEF. Echocardiography is mainly used to assess LVEF during cancer treatment for short- and long-term cardiotoxicity surveillance. Following the recommendations for chamber quantification, 2D echocardiography is the method of choice for LVEF calculation by applying the modified biplane Simpson technique. However, even following these strict recommendations, in patients undergoing cancer chemotherapy, 2D echocardiography showed variability in the detection of differences in LVEF close to 10%, calling into question the accuracy and reproducibility of LVEF by 2D echocardiography for cardiotoxicity assessment and monitoring. In a recent study, disagreement in LVEF by 2D echocardiography between two independent cardiologists was resolved by a consensus reached after subsequent joint evaluation of echocardiographic images. This emphasizes the fact that LVEF reproducibility is not ideal and that it ought to be better investigated and quantified in patients with breast cancer.
Three-dimensional (3D) echocardiography can be used to assess LVEF, and it has already proved its superiority for left ventricular (LV) functional quantification with respect to 2D echocardiography, and it may be preferable to 2D echocardiography for cardiotoxicity assessment as well.
In addition, several 3D echocardiographic software packages are available, and they can be used to evaluate cardiac function and compute LVEF. Previous studies have shown differences in cardiac functional assessment depending on the software package used in specific populations, including patients in sinus rhythm affected by cardiomyopathy and in patients affected by ischemic and nonischemic cardiomyopathy.
Considering the increasing number of patients affected by breast cancer and the increasing life expectancy along with the high occurrence of cardiotoxic effects, a better understanding of the relationship between LVEF variability and LVEF thresholds established to define cardiotoxicity onset is mandatory. To the best of our knowledge, no study has evaluated the variability of LVEF measurements in patients with breast cancer to verify whether such variability could interfere with cardiotoxicity diagnosis, misidentifying the intrinsic variability in LVEF computation as cardiotoxicity onset.
Therefore, the main aim of this study was to investigate whether variability in LVEF estimates from the data acquired with a 3D echocardiographic probe and computed using different software packages could affect cardiotoxicity onset definition in selected patients affected by breast cancer treated with anthracyclines and trastuzumab.
Materials and Methods
Study Population
One hundred eighty-one asymptomatic patients with histologic or cytologic diagnosis of breast cancer featuring overexpressed human epidermal growth factor receptor either at an early stage or locally advanced (stages I–IIIC), candidates for neoadjuvant therapy, or treated with radical surgery and candidates for adjuvant therapy were enrolled in the study. These patients were a subgroup of patients acquired within the Cartoon3D study (approved by CEIIAV Ethical Committee of Area Vasta Romagna, IRB IRST174.11_CARTOON3D Prot. 1695 I.5/62). The Cartoon3D study was a prospective clinical study in which the primary goal was to investigate whether new indexes of ventricular function from 3D echocardiography could provide cardiotoxicity onset information at an earlier stage compared with EF decrease. Inclusion criteria consisted of having had (1) a complete 2D and 3D echocardiographic examination with adequate apical acoustic window, (2) a neoadjuvant or adjuvant treatment program comprising an anthracycline-based regimen and trastuzumab and any concomitant hormonal therapy, and (3) adequate organ function (heart, bone marrow, renal, hepatic). The exclusion criteria were LVEF < 50%, valvular or ischemic heart disease or other diseases not allowing chemotherapy administration, or conditions that compromise patient compliance. The present study was an observational study in which all recruited patients, enrolled retrospectively, belonged to the Cartoon3D study, ensuring fulfillment of the inclusion and exclusion criteria defined in the Cartoon3D protocol.
All patients were treated with low-dose anthracyclines (240 mg/m 2 doxorubicin or 360 mg/m 2 epirubicin) followed by trastuzumab. The chemotherapy protocol included doxorubicin/epirubicin plus cyclophosphamide (in the adjuvant setting) or fluorouracil plus epirubicin plus cyclophosphamide (in the neoadjuvant setting) for four cycles (with dosing every 21 days), followed by 12 weeks of taxane with or without trastuzumab (consisting of paclitaxel with or without weekly trastuzumab for 12 weeks or docetaxel with or without trastuzumab every 3 weeks for four cycles); patients in the neoadjuvant setting also received concomitant hormonal therapy with gonadotropin-releasing hormone analog in premenopausal women or letrozole in postmenopausal women.
Cardiovascular risk factors such as hypertension, diabetes, hyperlipidemia, smoking, and alcohol use were recorded as well.
Cardiotoxicity onset was defined as a reduction of LVEF of >10% to <53%.
All patients gave written informed consent in accordance with the requirements of the local ethics committee.
Image Acquisition
Acquisition was performed using a Vivid E9 (GE Healthcare, Milwaukee, WI) ultrasound system equipped with a multiplanar full matrix array by an experienced cardiologist, with the patient in the left lateral decubitus position.
Three-dimensional echocardiographic acquisition of the entire left ventricle was performed by the same examiner in <10 seconds. All data sets were recorded according to recommendations for image acquisition and display using 3D echocardiography. The full-volume scan was acquired from the apical window, and care was taken to encompass the entire LV cavity. Consecutive four-beat electrocardiographically gated subvolumes were acquired during end-expiratory apnea to generate the full-volume data set.
The quality of the acquisition was then verified in each patient by an experienced cardiologist, by means of selecting nine-slice display mode available on the system to ensure that the entire LV cavity was included in the 3D echocardiographic full volume, and if unsatisfactory, the data set was reacquired.
Three-dimensional echocardiographic data sets were digitally stored in Digital Imaging and Communications in Medicine format and exported to a separate workstation equipped with commercially available software for offline analysis of LV volumes and LVEF. Each 3D echocardiography data set was analyzed using two software packages, denoted 3DE A and 3DE B . The same complete echocardiographic acquisition was repeated approximately 3 months after the previous acquisition, as suggested by the clinical protocol.
Image Processing
All analyses were performed offline, independently in all patients and in a random order by experienced cardiologists.
Quantification of 3D echocardiography LV volumes and LVEF was first performed using the 3DE A software package in semiautomatic mode. To avoid foreshortening, manual adjustment in apical four-, two-, and three-chamber views was performed. End-diastolic and end-systolic frames were defined as the first frame in which the mitral valve was closed and the frame immediately before mitral valve opening, respectively. These two frames were automatically detected by the software program from the electrocardiogram, and whenever required, they were manually adjusted to accurately identify these mitral valve positions. Following this step, as to the end-diastolic frame, the software required manual input of only two points on the four-chamber view image, one point identifying the center of the mitral annulus and one point identifying the LV apex. The endocardial border was automatically detected, and manual editing of the semiautomatically generated endocardial contour was applied to include LV outflow, as well as the papillary muscles and trabeculae within the LV cavity. To facilitate verification of the accuracy of the detected endocardial surface, arbitrary cross-sectional and long- and short-axis planes were displayed with the endocardium contour superimposed. At this stage, LV borders were manually adjusted whenever unsatisfactory.
Once completed for the end-diastolic frame, the analysis was repeated for the end-systolic frame as well. When surface detection was completed for LV end-diastolic volume (EDV) and LV end-systolic volume (ESV) and LVEF, stroke volume, cardiac output and heart rate were presented to the user.
When using the 3DE B software package, LV volume and LVEF assessment required alignment to the three standard apical views in a quad-screen display also including short-axis views at different levels of the left ventricle. End-diastolic and end-systolic frames were automatically defined.
Therefore, initialization was done by an accurate tracing of the endocardial borders in each long-axis view, at both end-diastole and end-systole. Manual tracing included trabeculae and papillary muscles. Starting from the manually detected contours, the program created a 3D volume cast and a volume-time curve by tracking the endocardial border of the whole heart throughout the entire cardiac cycle. Subsequently, a final reconstruction of the LV model was provided, with the calculation of LV volumes and LVEF.
Global longitudinal strain (GLS) was quantified offline using EchoPAC Workstation (GE Healthcare) and verified by an experienced cardiologist. GLS was measured in three apical views (apical long-axis and four- and two-chamber views) separately, and 18 myocardial segments were averaged to obtain GLS. Absolute values of GLS are expressed as percentages. Segments that were inadequately tracked were excluded from the analysis.
Statistical Analysis
The Kolmogorov-Smirnov test was applied to detect if data were normally distributed. The Wilcoxon rank sum test was used to test if estimates were significantly different ( P < .05). EDV, ESV, and LVEF are expressed as medians with interquartile ranges. Additional parameters are expressed as mean ± SD.
Three-dimensional echocardiographic values of EDV, ESV, and LVEF obtained using the 3DE A and 3DE B software packages were compared by means of linear regression and Bland-Altman analyses. To assess the consistency of such measurements, the intra-class correlation coefficient (ICC) was computed for EDV, ESV, and LVEF.
Intra- and interobserver variability was computed in a subgroup of the same 40 patients randomly selected by repeating the analysis 1 month later by the same observer and by a second expert reader, respectively. Variability measurements were computed as the SD between the estimates divided by their average value. Bland-Altman analysis of intra- and interobserver agreement was also performed. For both intraobserver and interobserver agreement, ICCs were evaluated to assess reliability.
To evaluate follow-up information regarding the time course of LV function in our study group, decline in LVEF at 3 months was computed and compared with variability measurements by means of the various methods.
In a subgroup of 64 patients, GLS from 2D echocardiography was available at the time of patient enrollment and at 3-month follow-up. GLS change was evaluated and compared with LVEF decrease.
All measurements were independently recorded. Statistical analysis was performed using MedCalc version 14.12.0 (MedCalc Software, Ostend, Belgium).
Results
Clinical, demographic, and cancer therapy characteristics of our population are reported in Table 1 .
Variable | Value |
---|---|
Age (y) | 52 ± 10 |
Weight (kg) | 63 ± 11 |
BMI (kg/m 2 ) | 24 ± 4 |
Cancer type | |
Ductal | 157 |
Lobular | 16 |
Unknown | 8 |
Grading | |
Grade 1 | 5 |
Grade 2 | 55 |
Grade 3 | 96 |
Unknown | 25 |
Cardiac risk factors | |
Hypertension | 30 |
Hypercholesterolemia | 15 |
Diabetes | 20 |
Smoking | 43 |
Drinking | 29 |
Analysis time for end-diastolic and end-systolic frames was about 4 ± 2 min with 3DE A software and about 2 ± 1 min with 3DE B software, including manual corrections when necessary.
EDV, ESV, and LVEF measurements computed using 3DE A and 3DE B showed a skewed distribution. No significant difference was found between 3D echocardiographic EDV, ESV, and LVEF estimates obtained using the 3DE A and 3DE B software package when applying the Wilcoxon rank sum test ( P = .88 for EDV, P = .23 for ESV, and P = .12 for LVEF; Table 2 ). Linear regression and Bland-Altman analysis of EDV, ESV, and LVEF values obtained using the two software packages are reported in Figure 1 and Table 2 , showing poor correlation coefficients and negligible biases.
EDV | ESV | LVEF | |
---|---|---|---|
Median (IQR) volume/LVEF by 3DE A (mL/%) | 82 (71–95) | 32 (27–40) | 60 (56–64) |
Median (IQR) volume/LVEF by 3DE B (mL/%) | 82 (70–100) | 31 (25–39) | 61 (56–67) |
r | 0.70 | 0.73 | 0.56 |
Linear regression | y = 0.59 x + 33.69 | y = 0.66 x + 12.25 | y = 0.50 x + 29.53 |
Bias (%) (mL/%) | 0 (0) | 1 (3.1) | −1 (−1.7) |
LOA (mL/%) | −36.5 to 27 | −13.5 to 17 | −12.8 to 11.7 |
ICC (range) | 0.69 (0.60–0.76) | 0.72 (0.65–0.79) | 0.54 (0.43–0.64) |
Intraobserver and interobserver variability results are reported in Figure 2 and Table 3 . Bland-Altman analysis of intra- and interobservers agreement is shown in Figure 3 . This group of 40 patients included three patients who had cardiotoxicity after a mean follow-up period of 86 ± 21 days. In these three patients, both intra- and interobserver variability results when using 3DE A were slightly lower compared with the average values; on the contrary, both variability values were consistently higher when computed using repeated measurements from the 3DE B software package.
3DE A | 3DE B | |||||||
---|---|---|---|---|---|---|---|---|
r | Mean ± SD (%) | Range (%) | ICC (range) | r | Mean ± SD (%) | Range (%) | ICC (range) | |
EDV | ||||||||
Intraobserver variability (%) | 0.83 | 4.0 ± 5.5 | 0–24.6 | 0.83 (0.71–0.91) | 0.88 | 5.5 ± 3.8 | 0.1–17.9 | 0.88 (0.78–0.93) |
Interobserver variability (%) | 0.92 | 4.5 ± 5.1 | 0–24.0 | 0.90 (0.82–0.95) | 0.81 | 6.0 ± 3.6 | 2.4–21.4 | 0.81 (0.67–0.89) |
ESV | ||||||||
Intraobserver variability (%) | 0.87 | 5.7 ± 5.7 | 0–21.1 | 0.85 (0.74–0.92) | 0.81 | 8.3 ± 5.3 | 0–19.4 | 0.79 (0.63–0.88) |
Interobserver variability (%) | 0.89 | 5.3 ± 7.7 | 0–45.6 | 0.89 (0.79–0.94) | 0.78 | 8.2 ± 6.8 | 0–32.9 | 0.77 (0.61–0.87) |
LVEF | ||||||||
Intraobserver variability (%) | 0.82 | 3.6 ± 3.5 | 0–17.0 | 0.81 (0.67–0.89) | 0.67 | 5.1 ± 3.1 | 0.6–11.1 | 0.67 (0.46–0.81) |
Interobserver variability (%) | 0.59 | 4.6 ± 4.2 | 0.1–18.4 | 0.59 (0.34–0.76) | 0.55 | 5.7 ± 5.0 | 0.2–26.4 | 0.53 (0.27–0.72) |
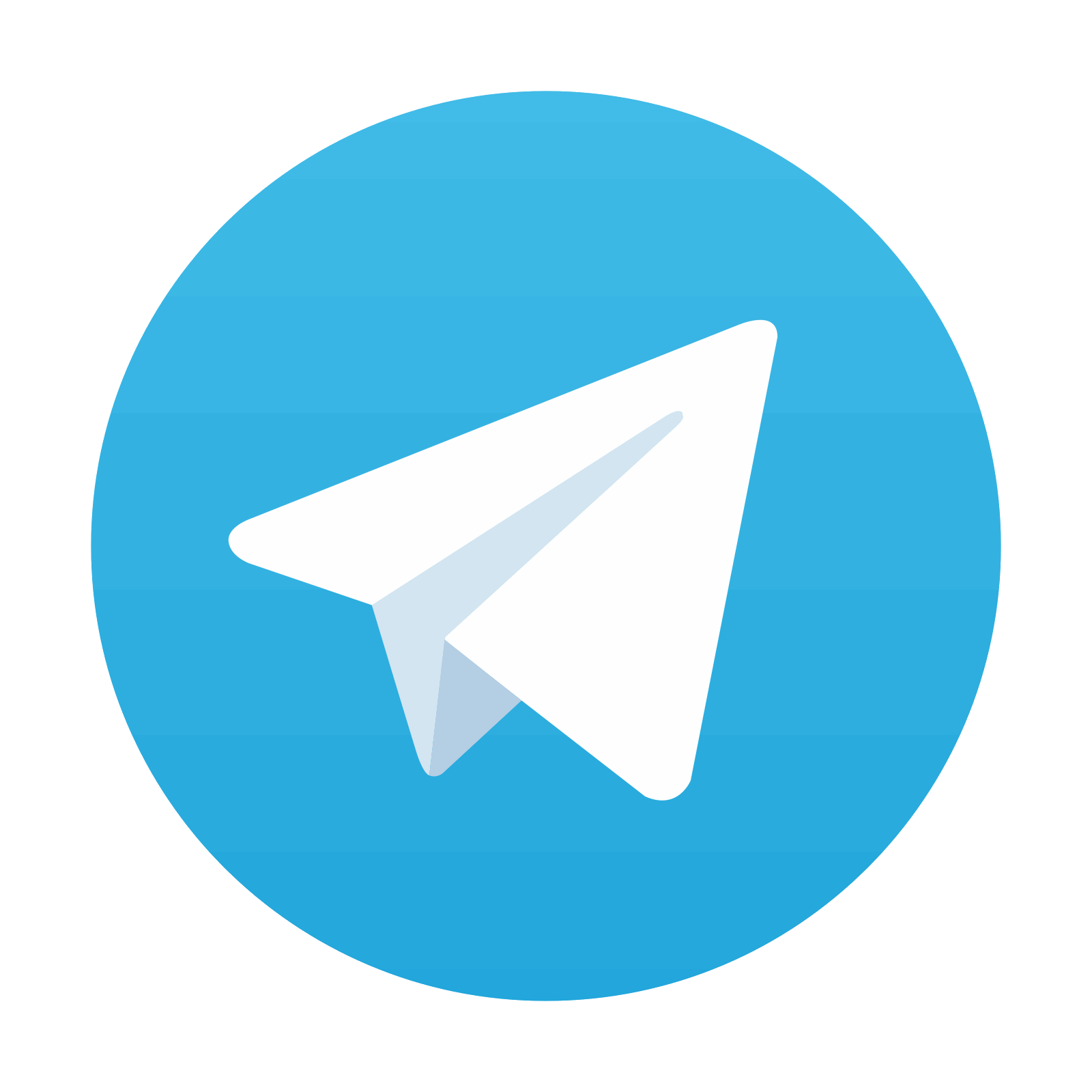
Stay updated, free articles. Join our Telegram channel

Full access? Get Clinical Tree
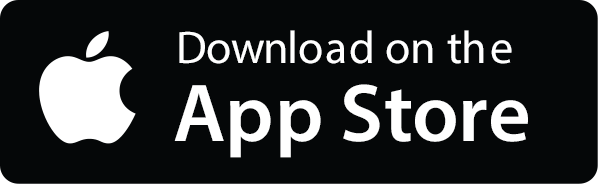
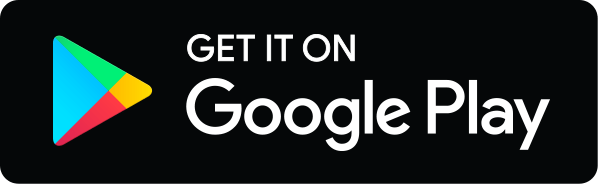
