Smooth muscle cell proliferation and extracellular matrix formation are responsible for disease progression in de novo and restenotic atherosclerosis. Internal elastic lamella (IEL) layer maintains the structural integrity of intima, and disruption of IEL may be associated with alterations in neointima, type III collagen deposition, and lesion progression in restenosis. Nineteen restenotic plaques (12 patients) procured during peripheral interventions were compared with 13 control plaques (12 patients) without restenosis. Hematoxylin & Eosin and elastic trichrome stains were used to measure length and percentage of IEL disruption, cellularity, and inflammation score. Type I and III collagens, smooth muscle cell (smc), fibroblast density, and nuclear proliferation (Ki67) percentage were evaluated by immunohistochemistry. IEL disruption percentage (28 ± 3.6 vs 6.1 ± 2.4; p = 0.0006), type III collagen content (0.33 ± 0.06 vs 0.17 ± 0.07; p = 0.0001), smc density (2014 ± 120 vs 923 ± 150; p = 0.0001), fibroblast density (2,282 ± 297 vs 906 ± 138; p = 0.0001), and Ki67 percentage (21.6 ± 2 vs 8.2 ± 0.65; p = 0.0001) were significantly increased in restenotic plaques compared to de novo plaques. Logistic regression analysis identified significant correlation between IEL disruption and neointimal smc density ( r = 0.45; p = 0.01) and with type III collagen deposition ( r = 0.61; p = 0.02) in restenosis. Increased IEL disruption may trigger cellular proliferation, altering collagen production, and enhancing restenotic neointima. In conclusion, understanding the pathologic and molecular basis of restenosis and meticulous-guided interventions oriented to minimize IEL damage may aid to reduce neointimal proliferation and the occurrence of restenosis.
Peripheral arterial disease (PAD) is a highly prevalent condition that markedly enhances risk for cardiovascular morbidity and mortality. Contemporary approaches in treating symptomatic PAD increasingly involve percutaneous methods to avoid morbidity from surgical revascularization. Restenosis remains a frequent complication of percutaneous treatment, thereby limiting overall efficacy of the vessel wall. Despite this common complication, pathologic correlates and mechanisms for lower extremity restenosis have not been well defined. One possibility is that vessel wall injury during percutaneous procedures may lead to internal elastic lamella (IEL) disruption resulting in neointima formation. Subsequent intimal cellular proliferation may lead to luminal narrowing thereby increasing risk for restenosis. In this context, the extent of IEL disruption may emerge as a trigger for the pathologic smooth muscle cell (smc) and fibroblast proliferation. Intimal smc proliferation contributes to collagen deposition and neointimal expansion. Reparative type III collagen produced in response to injury and associated healing process may link to restenosis. This study was designed to define the histopathologic correlation of human lower extremity restenotic atherosclerotic plaque with de novo plaque pertaining to IEL disruption and the impact on smc and fibroblast proliferation with type III collagen deposition leading to increased neointimal expansion and restenosis.
Methods
Peripheral atherosclerotic plaques from 24 patients were obtained in vivo during atherectomy procedure using Silver hawk atherotome (Fox Hollow Technologies, Redwood City, California) at the cardiac catheterization laboratory, Mount Sinai Hospital. Nineteen plaque sections from 12 patients with clinically documented restenosis and 13 plaque sections from 12 patients with native de novo atherosclerosis were studied. All tissue samples were collected at the time of intervention within 20 minutes after the procedure, washed in saline and fixed in 10% buffered formalin and immediately submitted for processing into paraffin blocks. This study was approved by the institutional review board at Icahn School of Medicine at Mount Sinai, New York. All the specimen procurements were done after informed consent was obtained for experimentation with human subjects. Also, this study conforms to the Declaration of Helsinki. Relevant demographic and clinical profiles were collected from case records and analyzed.
Hematoxylin & Eosin (H&E) sections were used to screen the presence or absence of lipid gruel, calcification, and the cellularity of the plaque. The cellularity was scored by semiquantitatively assessing the presence of total nucleated cells randomly screened in 10 high-power fields (HPF) in tunica intima and media and scored as follows: grade 1: scanty to minimal nucleated cells (occupying ≤25% of HPF); grade 2: moderate nucleated cells (occupying ≥25% to 50% of HPF); and grade 3: dense nucleated cells (occupying ≥50% of HPF) in the tunica intima and media. Furthermore, to evaluate the specific cell proliferation, we have used smc and fibroblast cell quantification using immunohistochemistry. H&E-stained sections were also used to measure the inflammation score of the plaque using semiquantitative analyzes by enumerating the number of mononuclear inflammatory cells and randomly counted in 10 HPF in the tunica intima and media and scored as follows: grade 1: 0 to 5 inflammatory cells; grade 2: 6 to 25 inflammatory cells; and grade 3: >25 inflammatory cells per HPF in the intima and tunica media.
Elastic trichrome–stained sections were screened for the presence or absence of IEL disruption, indicating the line of discontinuity in the IEL length. Furthermore, the total linear length of IEL in micrometer and the length of IEL disruption were measured with the aid of ocular micrometer attached to Olympus BX50 microscope. The percentage of IEL disruption was calculated by dividing the ratio of the mean IEL disruption length by mean total IEL length of the plaque and expressed as percentage of IEL disruption in both restenotic and de novo plaques. In addition, the correlation of degree of IEL disruption with the smc density was calculated.
To identify collagens, plaque tissue sections were stained with picrosirius red stain and used to quantify type I and III collagen as published previously. The area occupied by individual type I and III collagen was quantified in the entire intimal plaque area and divided by the total intimal plaque area in 10 random HPF and expressed as collagen density per square millimeter. Furthermore, the histochemical method of Sirius red was validated using the gold standard immunohistochemistry. Briefly, immunochemistry was performed using specific, non–cross-reacting mouse monoclonal antibody against type I collagen, rabbit polyclonal antibody against type III collagen (Abcam Inc., Cambridge, Massachusetts, 1:200 dilutions). Adopting avidin–biotin complex protocol (Vector Lab, Burlingame, California), the protein expression was detected by 3′3′-diaminobenzidine (Sigma, St. Louis, Missouri) chromogen. Positive and negative controls were stained by using human aorta fibrotic plaque tissue (for type I and III collagens). Sections were examined under an Olympus BX 50 light microscope (Olympus America, Center Valley, Pennsylvania), and the expression of type I and III collagen was measured. Using semiquantitative scale, grading was scored as follows: grade 0 = absent; grade 1 = <25% staining; grade 2 = 26% to 50% staining; grade 3 = 51% to 75% staining; and grade 4 = >76% to 100% staining.
To identify smc and fibroblast, immunochemistry procedure was performed using specific primary and secondary antibodies; for smc, α-actin (mouse monoclonal α-actin; Enzo Lab Inc., Farmingdale, New York, 1:40 dilutions) was used for labeling, and for fibroblast, FSP-1 (rabbit polyclonal; Abcam Inc., 1:100 dilutions) was used. Appropriate positive (colon for smc, skin for FSP-1) and negative (mouse and rabbit IgG) controls were included to distinguish nonspecific binding. Using the α-actin and FSP-1 immunostained slides, total number of positively stained α-actin and FSP-1 cells in 10 random HPF (×40) for each plaque was enumerated. The total plaque area occupied in HPF was measured in mm 2 using a computerized planimetry system. The density of α-actin and FSP-1–positive stained cells was calculated by dividing the total number of α-actin/FSP-1–positive stained cells by the total area measured in HPF.
Nuclear proliferation index was evaluated using Ki67 antibody (rabbit monoclonal Ki67, Abcam Inc, Cambridge, Massachusetts, 1:100 dilutions) for measuring the proliferating cells in the neointima. Appropriate positive (human tonsil tissue) and negative (mouse IgG) controls were included to distinguish nonspecific binding. The total number of Ki67-positive nuclei in the plaque intima that exhibited nuclear Ki67 protein expression was enumerated using light microscopy in 10 random HPF and estimated in relation to total number of nuclei studied in each field and expressed as percentage of Ki67-positive nuclei among the intimal cells.
Furthermore, immunofluorescent co-localization studies were performed to identify the intimal resident cells that express Ki67. Using primary antibodies for mouse monoclonal α-smooth muscle actin (α SMA; Sigma Aldrich, Missouri, 1:500 dilution) for detecting smc and rabbit polyclonal FSP-1(Abcam Inc., 1:100 dilution) for identifying fibroblasts were co-localized with rabbit monoclonal Ki67 (ab16667; Abcam Inc., 1:100 dilution) for cell proliferation. Additionally, positive and negative controls were included in parallel by substituting the primary antibody for IgG or specific IgG isotypes from the same species and at the same final concentration as the primary antibody. Secondary antibodies, donkey anti-mouse Alexa Fluor 488 or anti-rabbit Alexa Fluor 594 (A-21202 and A-21207, respectively; Invitrogen, New York, 1:500 dilutions), were used. Mounting medium containing DAPI (4′, 6-Diamidino 2-Phenylyindole) (H-1200-Vector Lab, California) was then applied. Quantification of FSP-1 and smc expressing Ki67 were performed in a blinded fashion. Mean cellular co-localization of all the images captured in ×20 magnification fields by the confocal Leica microscope was quantified. Images were acquired using Leica TCS SP5 DMI, inverted confocal laser scanning microscope at Mount Sinai’s Shared Resource Facility, and analyzed using Leica LAS AF lite software system.
Data are presented as mean ± SEM. For 2-group comparisons, Gaussian-distribution samples were juxtaposed with the 2-tailed Student t test and were preceded by a Levene F test for equality of variances. Non–Gaussian-distribution samples were compared by the Mann–Whitney nonparametric test. For multiple comparisons, one-way ANOVA was used. The following variables were used in the analysis: IEL disruption, percentage of IEL disruption, intimal and medial cellularity, intimal and medial inflammation grade, smc (α-actin) density, fibroblast (FSP-1) density, type I and III collagen density, and total Ki67 percentage. Linear regression analysis was used to evaluate correlations. IBM SPSS/PASW 20.0 software (SPSS Inc., Chicago, Illinois) was used for the analysis. A value of p ≤0.05 was considered statistically significant.
Results
Age incidence was similar in both groups (p = non-significant). Male gender showed a decrease in the incidence in restenotic group compared to de novo group (p = 0.02). Incidence of coronary artery disease and diabetes mellitus was higher in restenotic group compared to de novo group (p = non-significant). Our study infers elderly female gender preponderance in restenosis. All other clinical parameters showed no difference between the groups ( Table 1 ).
Case# | Restenosis | Age (years) | Male | BMI (kg /m 2 ) | Stenosis (%) | CAD | Claudication | Rest pain | Smoking | Hypertension | DM status | Cholesterol (mg/dl) | Hemoglobin (gm/dl) | Creatinine (mg/dl) |
---|---|---|---|---|---|---|---|---|---|---|---|---|---|---|
1 | Yes | 71 | 1 | 27.0 | 90 | 1 | 1 | 1 | 1 | 1 | 0 | 176 | 14.3 | 1.2 |
2 | Yes | 83 | 0 | 25.0 | 100 | 1 | 1 | 1 | 1 | 1 | 0 | 151 | 14.2 | 0.8 |
3 | Yes | 76 | 0 | 27.6 | 85 | 0 | 1 | 1 | 1 | 1 | 0 | 174 | 10.2 | 0.7 |
4 | Yes | 69 | 0 | 26.5 | 85 | 1 | 1 | 1 | 0 | 1 | 0 | 231 | 11.9 | 1.0 |
5 | Yes | 53 | 0 | 35.8 | 100 | 1 | 1 | 0 | 0 | 1 | 1 | 144 | 13.9 | 0.6 |
6 | Yes | 58 | 0 | 37.6 | 85 | 1 | 1 | 1 | 1 | 1 | 1 | 129 | 13.6 | 0.7 |
7 | Yes | 72 | 0 | 30.2 | 85 | 0 | 1 | 1 | 1 | 1 | 0 | 161 | 13.5 | 0.7 |
8 | Yes | 60 | 1 | 24.1 | 99 | 1 | 1 | 1 | 1 | 1 | 1 | 118 | 8.8 | 2.5 |
9 | Yes | 56 | 0 | 25.5 | 100 | 1 | 1 | 1 | 1 | 1 | 1 | 127 | 11.9 | 0.9 |
10 | Yes | 64 | 1 | 29.1 | 80 | 1 | 0 | 0 | 0 | 1 | 1 | 202 | 13.8 | 0.8 |
11 | Yes | 60 | 1 | 23.1 | 80 | 0 | 1 | 1 | 1 | 1 | 1 | 122 | 8.8 | 2.4 |
12 | Yes | 75 | 1 | 28.9 | 90 | 1 | 1 | 0 | 0 | 1 | 1 | 176 | 13.0 | 1.2 |
13 | NO | 62 | 1 | 23.9 | 80 | 1 | 1 | 0 | 1 | 1 | 0 | 122 | 15.3 | 0.7 |
14 | NO | 60 | 1 | 23 | 80 | 1 | 1 | 0 | 1 | 1 | 1 | 128 | 11.9 | 1.2 |
15 | NO | 59 | 1 | 23.2 | 90 | 1 | 1 | 1 | 1 | 1 | 0 | 122 | 10.2 | 1.5 |
16 | NO | 75 | 1 | 28.1 | 90 | 1 | 1 | 0 | 1 | 1 | 1 | 181 | 12.4 | 2.0 |
17 | NO | 82 | 1 | 24.4 | 70 | 0 | 1 | 1 | 0 | 1 | 0 | 125 | 13.5 | 1.2 |
18 | NO | 63 | 1 | 26.1 | 89 | 0 | 1 | 0 | 1 | 1 | 1 | 152 | 13.6 | 0.9 |
19 | NO | 56 | 0 | 27.6 | 90 | 1 | 1 | 0 | 1 | 1 | 1 | 124 | 13.9 | 1.2 |
20 | NO | 76 | 1 | 26.7 | 80 | 0 | 1 | 0 | 1 | 1 | 0 | 147 | 12.3 | 1.4 |
21 | NO | 55 | 1 | 21.0 | 85 | 0 | 1 | 0 | 1 | 1 | 0 | 192 | 13.0 | 1.5 |
22 | NO | 74 | 0 | 21.2 | 80 | 0 | 1 | 0 | 0 | 1 | 0 | 285 | 12.0 | 0.7 |
23 | NO | 58 | 1 | 27.5 | 85 | 1 | 1 | 1 | 1 | 1 | 0 | 230 | 14.0 | 1.2 |
24 | NO | 62 | 1 | 23.9 | 60 | 0 | 1 | 1 | 1 | 1 | 0 | 137 | 15.3 | 0.7 |
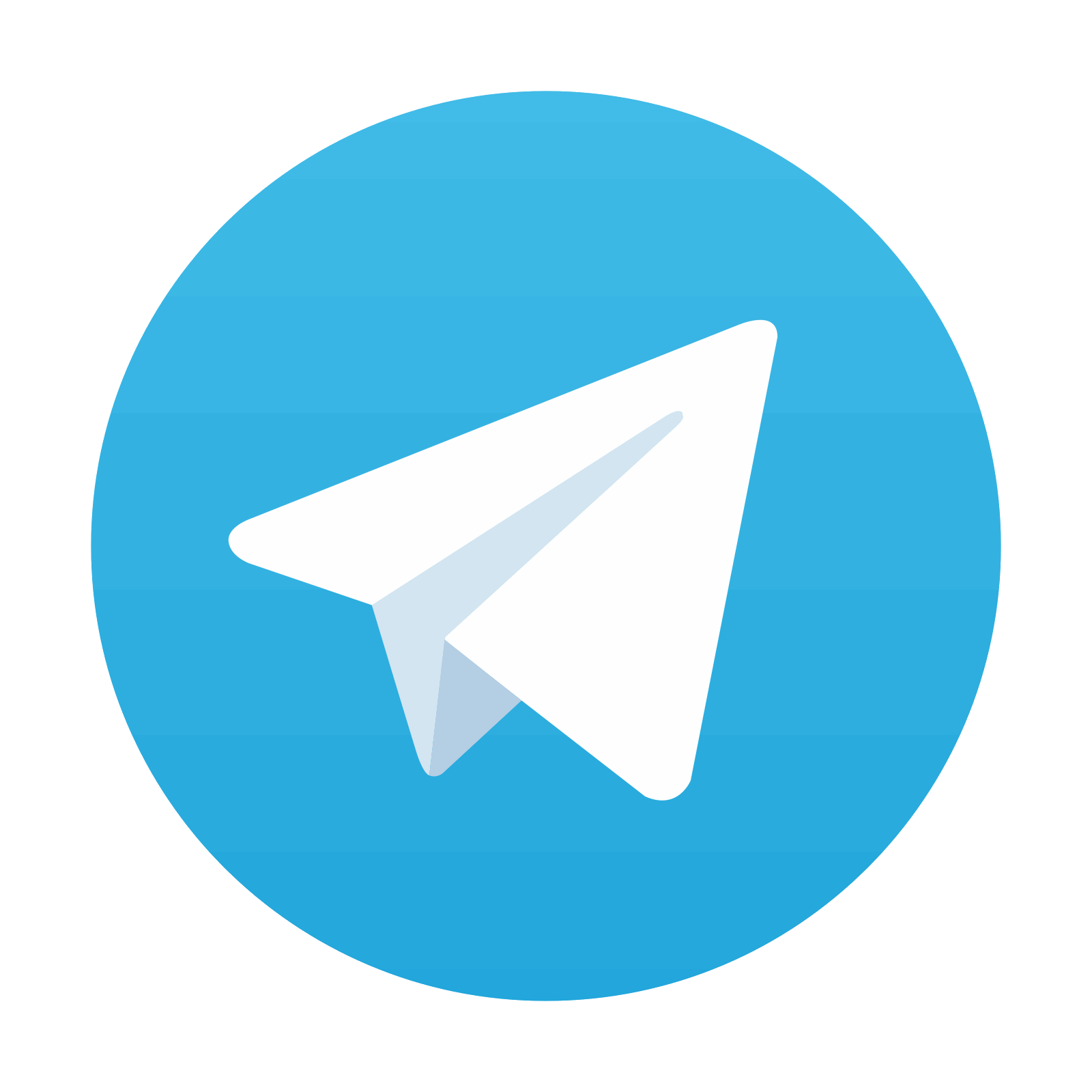
Stay updated, free articles. Join our Telegram channel

Full access? Get Clinical Tree
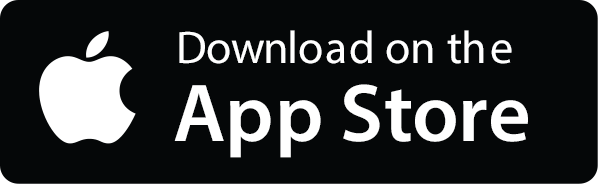
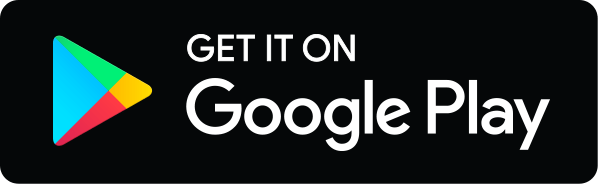
