Background
Mechanical discoordination as studied by magnetic resonance imaging has been shown to be a better predictor of left ventricular (LV) reverse remodeling after cardiac resynchronization therapy (CRT) compared with mechanical dyssynchrony.
Materials and Methods
This study assessed the value of acute recoordination derived from speckle-tracking echocardiography for predicting response to CRT compared with acute resynchronization. Thirty patients with heart failure scheduled for CRT were studied at baseline, immediately after CRT, and after 6 months of CRT. Acute recoordination after CRT was indexed by an acute reduction in radial discoordination index (RDI), defined as the ratio of average myocardial thinning to thickening during the ejection phase.
Results
CRT responders were defined as those patients whose LV end-systolic volume decreased by ≥ 15% at the 6-month follow-up. Immediately after CRT, the responders (n = 18) demonstrated a significant reduction in RDI ( P < .001), which was sustained at the 6-month follow-up ( P < .001). The nonresponders, however, did not show a significant change in RDI after CRT. LV reverse remodeling at the 6-month follow-up was significantly correlated with acute recoordination ( r = 0.75, P < .001) but weakly correlated with acute resynchronization ( r = 0.43; P = .02).
Conclusions
Receiver operating characteristic analysis revealed that acute recoordination provided the best separation for prediction of CRT responders compared with acute resynchronization, baseline dyssynchrony, or baseline discoordination. LV recoordination after CRT is an acute phenomenon and predicts response to CRT at 6-month follow-up better than resynchronization.
Cardiac resynchronization therapy (CRT) has proven helpful in patients with heart failure and a wide QRS complex. Despite its efficacy, 30% to 40% of patients do not benefit from CRT. Several studies suggested that mechanical dyssynchrony is a potential tool to identify CRT responders. In addition, immediate reduction in left ventricular (LV) dyssynchrony after CRT was thought to predict LV reverse remodeling at 6-month follow-up. However, the Predictors of Response to CRT (PROSPECT) study showed disappointing results on the use of mechanical dyssynchrony in predicting response to CRT.
In a recent study using circumferential strain by magnetic resonance imaging, Kirn et al demonstrated that mechanical discoordination (opposite strain within the LV wall) predicted reverse remodeling after CRT better than mechanical dyssynchrony. In the present study, we hypothesize that LV recoordination rather than resynchronization is a better approach to predict response to CRT. A novel approach to detect LV recoordination with speckle-tracking echocardiography was evaluated for predicting CRT response compared with resynchronization derived from classic dyssynchrony metrics. Immediate LV recoordination after CRT was indexed by an acute reduction in radial discoordination index (RDI), defined as the ratio of average myocardial thinning to thickening during the ejection phase.
Materials and Methods
Patients
Consecutive patients with heart failure scheduled for CRT implantation were enrolled. The selection criteria for CRT included moderate to severe heart failure (New York Heart Association functional class III and IV) despite optimal medical therapy, LV ejection fraction (EF) ≤ 35%, and QRS duration > 120 ms. Two age-matched groups with narrow QRS (duration < 120 ms) served as controls: 1) patients without structural heart disease and with a normal echocardiogram (normal group) and 2) patients with LV EF < 35% (low EF group). Patients’ clinical status, LV volumes, and EF were assessed before CRT implantation and after 6 months of CRT. LV dyssynchrony and discoordination were assessed at baseline, immediately after CRT, and at a 6-month follow-up. Each parameter was measured from 3 consecutive beats and averaged for purpose of analysis.
Cardiac Resynchronization Therapy Procedure
The pacing leads were positioned at the right ventricular apex or mid-septum, at the right atrial appendage, and in the posterior or posterolateral branch of coronary vein. The atrioventricular interval was optimized using the established method to ensure adequate LV filling. No adjustments were made to the interventricular interval before the 6-month follow-up. The local ethics committee approved the study protocol, and all subjects gave informed consent.
Echocardiography
Subjects were imaged in the left lateral decubitus position with a commercially available system (Vivid 7, General Electric Vingmed Ultrasound, Horten, Norway). Standard 2-dimensional and color Doppler data triggered to the QRS complex were saved in cine-loop format. The LV volumes and EF were measured from the conventional apical 2- and 4-chamber images using the biplane Simpson method. The severity of mitral regurgitation was evaluated by color jet area and as percent jet area relative to left atrial size. Patients with a reduction of ≥ 15% in LV end-systolic volume at the 6-month follow-up were considered CRT responders.
LV dyssynchrony and discoordination were evaluated by speckle-tracking echocardiography from the parasternal short-axis views at the level of papillary muscles and mitral valve. Gain settings and imaging width were adjusted to optimize the gray scale (frame rate of 50-80 hertz) and not to compromise the image resolution. Each time, we analyzed a single beat and the gating was set to begin with the onset of the QRS complex. End systole was chosen as the single frame for marking the region of interest to include the maximal wall thickness for strain analysis. The inner marker was traced to the endocardial-cavity interface at end systole, and the outer marker was traced to the LV epicardium to obtain reproducible time–strain curves. The software (EchoPac 6.1, General Electric Vingmed Ultrasound) automatically tracked the image speckle and produced 6 regional radial strain and strain rate curves. The region of interest was redrawn if tracking quality was poor or the curves were viewed to be inadequate.
Radial Dyssynchrony and Discoordination Analysis
The data of radial strain and strain rate were exported to a spreadsheet program (Microsoft Excel, Microsoft Corp, Seattle, WA) to calculate dyssynchrony and discoordination. The time to peak strain from each of the 6 mid-LV segments was measured. Dyssynchrony indices were defined as the time difference between the anteroseptal and posterior segments (AS-P delay), the standard deviation of times to peak strain for 6 segments (RS-SD), and the time difference between the earliest and the latest segments of the 6 segments (MD-6). The immediate resynchronization was defined as the percentage difference between preimplantation LV dyssynchrony and the one at immediate follow-up.
The radial strain rate signals from the 6 segments were split into positive signals e p (t) and negative signals e n (t). Averaging e p (t) and e n (t) over 6 basal-LV or mid-LV signals resulted in average myocardial thickening and thinning, respectively. Integration of these signals in the ejection phase resulted in a positive strain ϵ p , representing the amount of myocardial contraction, and a negative strain ϵ n , representing the amount of myocardial thinning. The timing of ejection phase (between aortic valve opening and aortic valve closure) was determined from the Doppler velocity signal at the LV outflow tract. RDI was calculated as a ratio of the amount of average myocardial thinning relative to the amount of average myocardial thickening (denominator) during the ejection phase. The RDI was measured from 6 basal LV segments (RDI-B), 6 mid-LV segments (RDI-M), and 12 (ie, 6 basal and 6 mid) LV segments (RDI-12). The difference between the preimplantation RDI and the RDI at immediate follow-up defined the acute recoordination.
Statistical Analysis
Data were expressed as mean ± standard deviation for continuous variables and as absolute frequencies and relative percentages for categoric variables. Continuous variables were compared by means of 1-way analysis of variance for overall comparison and the Dunnett test for post hoc comparisons. The chi-square test with the Fisher exact test (if necessary) was used to compare categoric variables. Dyssynchrony and discoordination indices were summarized with the median and 25th and 75th percentiles and compared with the Mann–Whitney test for each pair of groups. Changes over time within a group were analyzed by the Friedman test and Wilcoxon test for post hoc comparisons. The Pearson correlation analysis was performed to determine the relationship between acute recoordination/resynchronization and reverse remodeling at the 6-month follow-up. Receiver operating characteristic analysis was used to determine the optimal cutoff value of the variables for prediction of response to CRT. Reproducibility was tested using the Bland–Altman method. For all tests, a value of P < .05 was considered statistically significant.
Results
Baseline variables of the studied patients are summarized in Tables 1 and 2 . All patients who received CRT survived during the 6-month follow-up. Nonresponders were similar to responders in all aspects, except for greater prevalence of ischemic cardiomyopathy. Both groups improved clinically, as indicated by the decrease in New York Heart Association class. However, after 6 months of CRT the LV volumes and mitral regurgitation decreased and LV EF increased in the responders only.
Narrow QRS control groups | ||||
---|---|---|---|---|
Normal (n = 20) | Low EF (n = 40) | CRT group (n = 30) | P | |
Age (y) | 65 ± 9 | 67 ± 8 | 69 ± 10 | .22 |
Female, n (%) | 9 (45) | 19 (48) | 18 (60) | .48 |
Ischemic cause, n (%) | 0 (0) | 20 (50) ∗ | 12 (40) ∗ | .001 |
LV EF (%) | 64 ± 9 | 26 ± 6 ∗ | 23 ± 8 ∗ | <.001 |
LV EDV (mL) | 79 ± 19 | 163 ± 60 ∗ | 172 ± 65 ∗ | <.001 |
QRS duration (ms) | 91 ± 8 | 95 ± 11 | 166 ± 23 ∗,† | <.001 |
Nonresponders (n = 12) | Responders (n = 18) | P | |
---|---|---|---|
Age (y) | 67 ± 11 | 71 ± 8 | .24 |
Female, n (%) | 6 (50) | 12 (67) | .36 |
Ischemic cause, n (%) | 8 (67) | 4 (22) | .02 |
QRS duration (ms) | 170 ± 29 | 164 ± 20 | .47 |
Medication, n (%) | |||
ACE inhibitors/ARB | 11 (92) | 17 (94) | 1.0 |
B-blockers | 10 (83) | 16 (89) | 1.0 |
Diuretics | 9 (75) | 12 (57) | .70 |
NYHA class | |||
Baseline | 3.2 ± 0.3 | 3.1 ± 0.3 | .65 |
6 months | 2.6 ± 0.7 † | 1.5 ± 0.5 ∗ | <.001 |
LV EDV (mL) | |||
Baseline | 194 ± 75 | 158 ± 55 | .14 |
6 months | 209 ± 90 | 101 ± 33 ∗ | .002 |
LV ESV (mL) | |||
Baseline | 156 ± 72 | 121 ± 48 | .12 |
6 months | 171 ± 83 | 56 ± 24 ∗ | .001 |
LV EF (%) | |||
Baseline | 21 ± 8 | 24 ± 7 | .37 |
6 months | 21 ± 8 | 46 ± 8 ∗ | <.001 |
Mitral regurgitation (%) | |||
Baseline | 22 ± 10 | 24 ± 12 | .68 |
6 months | 20 ± 10 | 8 ± 5 ∗ | .003 |
Comparisons of Baseline Dyssynchrony and Discoordination
Comparisons of dyssynchrony and discoordination indices among control and CRT groups are shown in Table 3 . All indices differed significantly between control and CRT groups. Significant differences were present between nonresponders and responders in baseline RDI-M, AS-P delay, and RS-SD. Figures 1 and 2 s how baseline dyssynchrony and discoordination analyses in a normal subject, a nonresponder, and a responder. In the normal subject, all mid-ventricular segments contracted synchronously and the peak strain of each segment occurred almost simultaneously after the end of ejection phase ( Figure 1 ). During the ejection phase, myocardial thickening was dominant and myocardial thinning was negligible. In the responder, peak radial strain occurred before the ejection phase in 3 early contracted segments ( Figure 2 ). These early contracted segments developed myocardial thinning that was indicated by the negative strain rate during the ejection phase. Similarly, the strain analysis in the nonresponder showed a comparable measure of dyssynchrony by calculating the time-to-peak difference between the early and late contracted segments. However, in the nonresponder, the early peak strain occurred later at the early ejection phase with a smaller peak value and in fewer segments compared with the responder. Therefore, the amount of myocardial thinning relative to myocardial thickening during the ejection phase was smaller than that of the responder.
Narrow QRS control groups | CRT group | ||||
---|---|---|---|---|---|
Normal (n = 20) | Low EF (n= 40) | All CRT patients (n = 30) | Nonresponders (n = 12) | Responders (n = 18) | |
RDI-B | 1 (0, 2) ∗ | 11 (6, 19) † | 16 (7, 59) | 17 (9, 38) | 14 (2, 62) |
RDI-M | 1 (0, 1) ∗ | 18 (10, 30) ∗ | 58 (37, 115) | 36 (10, 163) | 70 (51, 115) ‡ |
RDI-12 | 1 (0, 1) ∗ | 15 (8, 22) ∗ | 30 (15, 69) | 18 (11, 58) | 32 (18, 78) |
AS-P delay | 53 (28, 78) ∗ | 118 (75, 180) ∗ | 305 (189, 353) | 192 (110, 318) | 326 (193, 354) ‡ |
RS-SD | 28 (20, 39) ∗ | 90 (58, 129) ∗ | 175 (131, 198) | 120 (78, 181) | 174 (130, 202) ‡ |
MD-6 | 62 (48, 85) ∗ | 227 (125, 310) ∗ | 371 (306, 470) | 292 (191, 435) | 365 (305, 466) |
∗ P < .001 vs all CRT patients.
† P < .05 vs all CRT patients.
Recoordination and Resynchronization after Cardiac Resynchronization Therapy
Table 4 shows index changes over time after CRT. In the nonresponders, all discoordination and dyssynchrony indices failed to show a significant change after CRT. In contrast, all indices differed significantly after CRT in the responders. In the post hoc comparisons, all indices demonstrated a significant reduction immediately after CRT. At the 6-month follow-up, the reductions in RDI and all dyssynchrony indices by CRT were still present. Figure 3 presents the individual values of RDI-M and AS-P delay before and after CRT. The baseline RDI-M values of the responders and nonresponders were widely separated. The responders had a medium to high value (35%-160%) that consistently decreased immediately after CRT ( P < .001). In contrast, the nonresponders had a low (<40%) or high RDI-M (>200%) value that increased or did not decrease sufficiently at the immediate follow-up ( Figure 3 A). The values of AS-P delay showed substantial reductions immediately after CRT in most of the responders but also in some of the nonresponders ( Figure 3 B).
Nonresponders (n = 12) | Responders (n = 18) | |||||||
---|---|---|---|---|---|---|---|---|
Baseline | Immediate | 6 mo | P | Baseline | Immediate | 6 mo | P | |
RDI-B (%) | 17 (9, 38) | 18 (2, 71) | 11 (4, 37) | .08 | 14 (2, 62) | 5 (2, 7) ∗ | 3 (1, 4) ∗,‡ | .001 |
RDI-M (%) | 36 (10, 163) | 58 (31, 125) | 54 (29, 218) | .21 | 70 (51, 115) | 10 (3, 17) ∗ | 2 (1, 4) ∗,† | <.001 |
RDI-12 (%) | 18 (11, 58) | 33 (10, 75) | 35 (11, 49) | .78 | 32 (18, 78) | 7 (4, 11) ∗ | 3 (1, 4) ∗,† | <.001 |
AS-P delay (ms) | 192 (110, 318) | 124 (78, 299) | 157 (77, 393) | .17 | 326 (193, 354) | 109 (69, 188) ∗ | 76 (14, 111) ∗,† | <.001 |
RS-SD (ms) | 120 (78, 181) | 108 (80, 173) | 107 (92, 218) | .78 | 174 (130, 202) | 71 (50, 146) ∗ | 50 (11, 75) ∗,† | <.001 |
MD-6 (ms) | 292 (191, 435) | 218 (167, 379) | 258 (223, 440) | .47 | 365 (305, 466) | 152 (114, 321) ∗ | 117 (28, 162) ∗,† | <.001 |
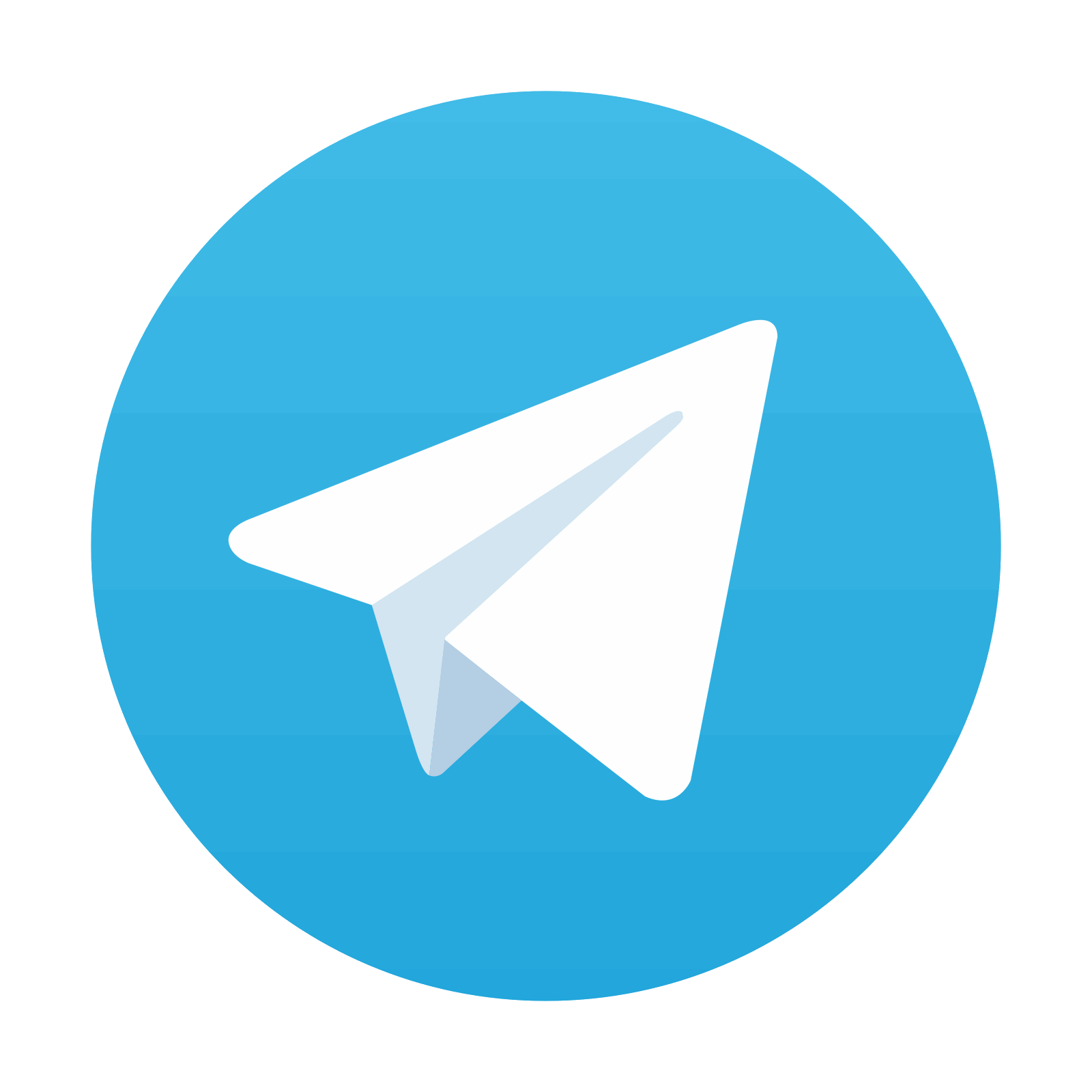
Stay updated, free articles. Join our Telegram channel

Full access? Get Clinical Tree
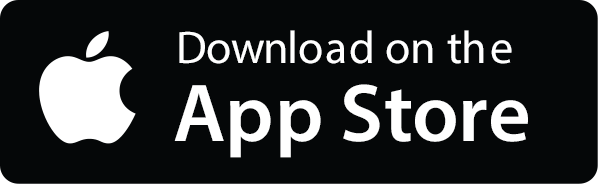
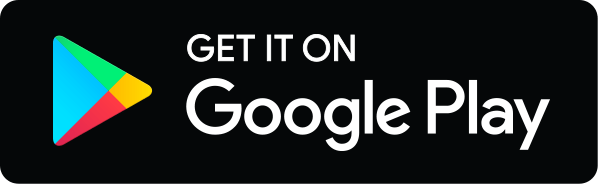
