Keywords
Embryo heart rate perturbationsBlood’s motive energyHeart pacingSinus node cooling and heatingIntrinsic heart rateMatching of heart rate and outputTo further substantiate the importance of metabolism as the primary factor, affecting the movement of blood, let us look at experiments in which the metabolic rate has been changed by subjecting the embryo to environmental hypo- or hyperthermia. Since the chick embryo is poikilothermic, its hemodynamic functions are exquisitely sensitive to change in temperature. When the temperature was raised from 37 to 40 °C in HH stage 18, 21, and 24 chick embryos, there was a linear increase in heart rate and aortic flow, while the stroke volume remained unchanged [1]. Similarly, a global decrease in temperature to 31.1 °C in the chick embryos at the same developmental stages resulted in a proportional decrease in heart rate, vitelline artery pressure, aortic flow, and no change in stroke volume [2].
In his pioneering efforts to demonstrate autonomous movement of the blood, Manteuffel-Szoege subjected stage 24 chick embryos to progressive cooling and observed the movement of blood in the vitelline vessels. Blood in the venous limb of the circuit was the first to slow down and the flow ceased at 20–25 °C; however, the blood in the arteries continued to oscillate with each heartbeat, without any net macroscopic movement. By 30–90 min after cessation of the circulation, congestion was noted in the arterial limb of the vitelline circulation, confirming continuous movement of blood at the level of microcirculation. In the second experiment, the embryo’s heart was excised and the movement of the blood was observed under normothermic conditions. In spite of hemorrhage, the movement of the blood continued in the vitelline veins for 10–15 min, but interestingly, rather than flowing backward, the blood in the arteries continued to move in the centrifugal (peripheral) direction. On the basis of this (and other experiments to be reviewed in Part II), Manteuffel-Szoege concluded that the blood possesses its own motive energy which is “inseparably connected with the thermal conditions” of the embryo [3].
In addition to the altering of the embryo’s thermal environment, the embryonic heart rate can be selectively manipulated by transient thermal probe application. The method allows the study of rapid alterations in heart rate, without associated metabolic effects. Since such an experiment is notoriously difficult to perform in an animal with intact cardiovascular reflexes and hormonal feedback loops, the early embryo circulation is, once more, an ideal model.
Cuneo et al. subjected stage 17–27 chick embryos to selective increase/decrease of heart rate by applying a heated/cooled metal probe to the area of the chick’s sinus venosus and measured changes in aortic flow and stoke volume during each developmental stage. For each developmental stage, there was a decrease in aortic flow, with either an increase or a decrease of heart rate beyond the embryo’s own “intrinsic” rate. Moreover, there was a reciprocal relation between the “intrinsic” heart rate and stroke volume, i.e., it increased during slower rates and diminished when the heart was stimulated faster than its own intrinsic rate [4]. Applying a similar method, the heart-rated dependence of ventricular filling was also confirmed by Phelan et al. [5].
The unique nature of the coupling between blood flow and intrinsic heart rate has been further demonstrated by direct cardiac pacing. When the site of presumed sinus venosus in stage 24 embryos was artificially paced at rates that were 150% above the “intrinsic” heart rate, the stroke volume (SV) decreased from 0.36 ± 0.02 to 0.12 ± 0.02 mm3/beat. Only a slight increase in SV was noted when pacing rate was set at 50% or 75% of the intrinsic heart rate. The disproportionately low stroke volumes were ascribed to lack of synchrony between active (atrial) and passive (ventricular) filling. While pacing near the intrinsic rates resulted in aortic flows that were comparable to normal, there was a marked decrease of aortic flow at the extreme pacing rates, either above or below the intrinsic. This was in marked contrast to heart rate perturbation with a heated probe, where the synchrony between the active and passive ventricular filling was maintained even at decreased heart rates [6].

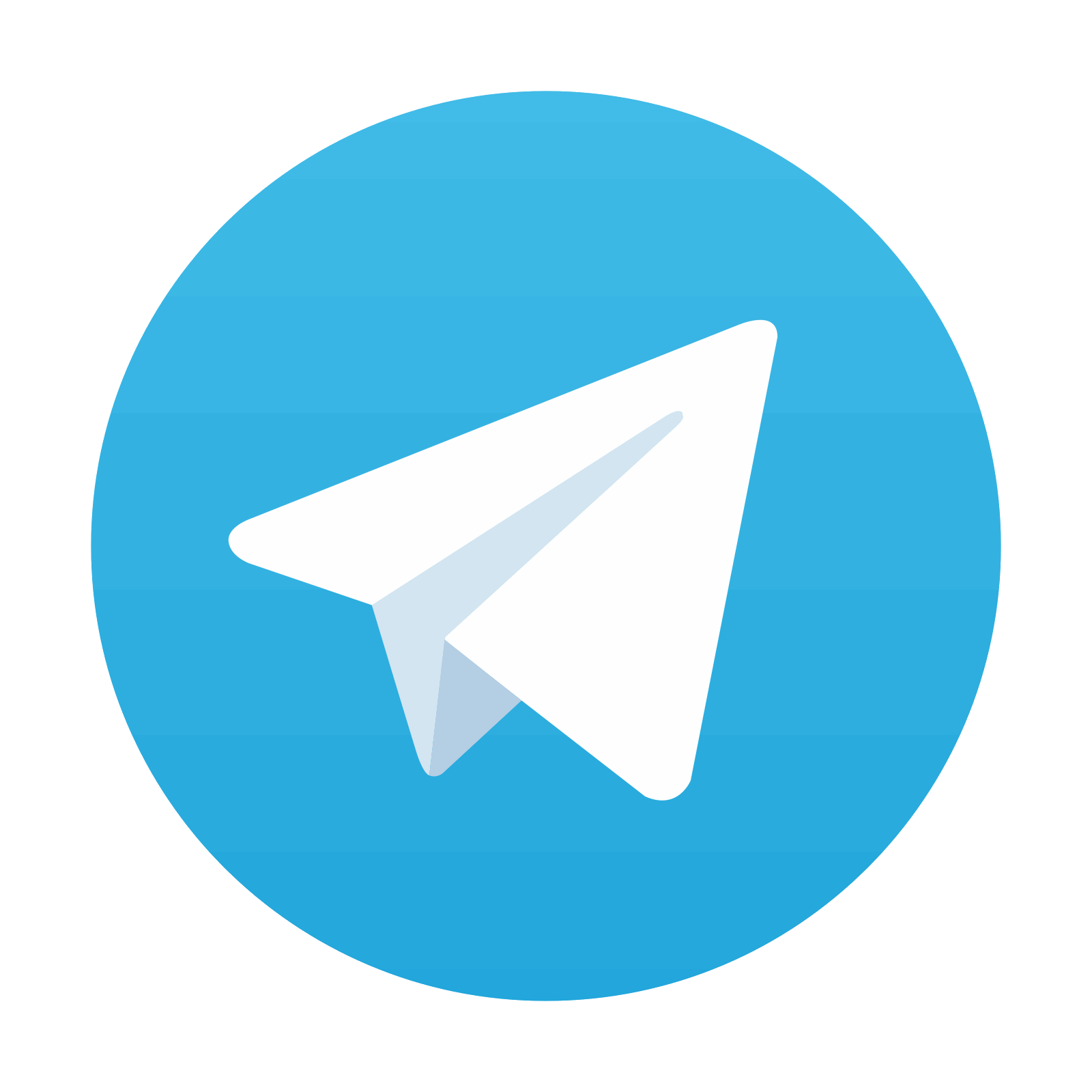
Stay updated, free articles. Join our Telegram channel

Full access? Get Clinical Tree
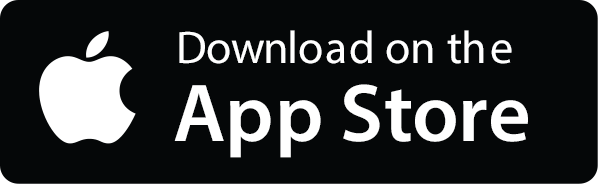
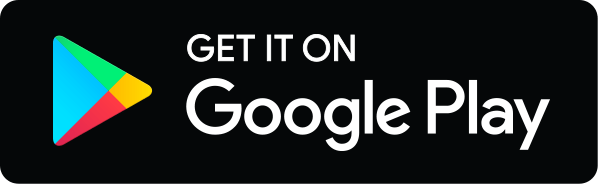