Chapter 21
Radiation Safety
Stephen W.K. Cheng
Advances in radiology, nuclear medicine, and radiation therapy have led to a dramatic increase in the use of radiation for diagnostic, interventional, and therapeutic purposes. Although radiation exposure from diagnostic procedures is generally low and comparable to natural background doses, therapeutic use of radiation involves a higher level of exposure, which can be harmful if not controlled.1
Radiation poses risk to workers and patients alike. Cardiologists, radiologists, and vascular and orthopedic surgeons operate medical x-ray equipment frequently. Operating room assistants are often not properly educated regarding the potential damaging effects of radiation and are not trained to minimize exposure to themselves or to the patient. Patients may undergo procedures without being properly informed of the risks. These deficiencies may result in unnecessary exposure to both patients and staff.
The concern about radiation exposure is particularly pressing in the field of vascular surgery, where interventional procedures have evolved to be the mainstays of clinical practice. Percutaneous angioplasty and stenting of peripheral and carotid arteries, as well as endovascular stent-grafts for treating abdominal and thoracic aortic diseases are now first-line treatment options. Advanced techniques such as fenestrated and branched endografts for complex thoracoabdominal aneurysms also carry significant radiation exposure to surgeons and the patient. Computed tomography (CT) as the primary modality of investigation and follow-up has become widely accepted.
The principles of radiation safety have been well addressed by a number of national bodies—for instance, the International Commission on Radiological Protection (ICRP) and the National Council on Radiation Protection and Measurements (NCRP). These organizations provide valuable resources and publications that disseminate information and recommendations about radiation exposure and protection. This chapter deals only with diagnostic and interventional use of radiation and will not address the use of radiation in nuclear medicine procedures and radiotherapy, because the relevance of these latter uses to vascular surgery practice is limited.
Types of Radiation
Radiation is a form of energy emitted as electromagnetic waves or particles. It can be classified as nonionizing (ultrasound, magnetic resonance, laser, microwaves) or ionizing (x-rays, gamma rays). Nonionizing radiation does not possess the energy to ionize atoms of the absorbing matter. Ionizing radiation consists of alpha and beta particles, neutrons, and energetic photons (ultraviolet and above), which contain sufficiently high energy to interact with atoms and produce biologic injury. The most common forms of ionizing radiation used in medicine are x-rays, gamma rays, beta rays, and electrons.
Measurement
The amount of ionization that radiation produces in air, measured in roentgens (R), does not accurately reflect its potential to cause biologic injury. More important, the actual damage or biologic effect of radiation depends on the total energy of radiation absorbed per unit mass, the sensitivity of the organ, and the actual strength of the radiation. To quantify radiation for the purposes of risk assessment and setting standards and limits, the terms absorbed dose, equivalent dose, and effective dose are commonly used (Table 21-1).
Absorbed Dose
The absorbed dose is a measure of the amount of energy deposited in a medium by ionizing radiation per unit mass of matter and is equal to the amount of heat generated by the radiation per tissue weight in a specified material. The International System (SI) unit for absorbed dose is the gray (Gy), named after Louis Harold Gray, a British physicist. One gray equals 1 joule (J) of energy absorbed per kilogram (J/kg). Previously, the unit most commonly used was the “radiation absorbed dose (rad)” (1 Gy = 100 rad). The absorbed dose is not a good indicator of biologic effect because different ionizing radiation produces different degrees of damage. The gray describes the physical amount of radiation and is generally used only when treatment with radiation is prescribed, such as in radiotherapy.
Equivalent Dose
The equivalent dose is a measure of the radiation dose to tissue and takes into account the different degrees of damage by different types of radiation by introducing a radiation weighting factor (WR). The SI unit of equivalent dose is the sievert (Sv), named after Rolf Sievert, a Swedish physicist. Thus equivalent dose = absorbed dose × WR. (Another unit, the roentgen equivalent man, or rem, is still sometimes used; 1 Sv = 100 rem.)
WR is calculated by the type of radiation (1 for x-rays and gamma rays and 3 to 10 for protons and neutrons). The sievert better describes the biologic effect of radiation and is commonly used when risk from ionizing radiation is assessed. It also allows quantification of risk and comparison to other commonly encountered modes of exposure.
Effective Dose
Because different tissues and organs have different sensitivity to radiation, the concept of effective dose is introduced to take into account the part of the body irradiated and the volume and time over which the dose is applied. The effective dose is formed by weighting the equivalent dose by another tissue weighting factor (WT) according to the distribution of radiation and the radiosensitivity of various organs. To avoid confusion, WR and WT are sometimes grouped together into one single weighting factor (W). The effective dose is also measured in sieverts.
Effective dose in radiation protection can be used prospectively for planning and optimization of radiation protection, as well as retrospectively for assessment of risk. It is mainly used as a protective and regulatory quantity and not for epidemiologic study of populations.
Because grays and sieverts quantify relatively large amounts of radiation, in medical use radiation is typically described in milligrays (mGy) or millisieverts (mSv).
Biologic Effects of Radiation
Ionizing radiation damages living cells, which can repair themselves, die, or undergo a mutation. The effects of radiation on biologic tissue are generally classified as two types: deterministic effects and stochastic effects.
Deterministic Effects
Deterministic effects are dose dependent and result in cell death. These are acute events when a threshold level of radiation has been exceeded, and the higher the dose, the greater the injury (Fig. 21-1). The threshold is not absolute and can vary among individuals. Table 21-2 shows some threshold levels of human organs with corresponding deterministic effects. Doses required to produce deterministic effects are often large and exceed 1 to 2 Sv. Symptoms arise when a significant proportion of cells are killed by radiation, and subsequent inflammation or fibrosis may produce additional damage to the organ. Examples of deterministic effects include radiation dermatitis, cataracts, infertility, and organ atrophy or fibrosis (Figs. 21-2 and 21-3). Recently, concerns have increased about the late manifestation of cataracts from low doses of ionizing radiation, and the recommended threshold of the lens has been lowered to 0.5 Sv.
Whole-body exposure to 10- to 20-Gy high-energy radiation, delivered at one time, can be fatal to humans. For acute whole-body equivalent doses, 0.5 to 1 Sv may produce light radiation sickness; 1 Sv causes slight blood changes; and 2 to 3 Sv causes nausea, hair loss, and hemorrhage. An acute dose of 3 Sv causes death in 50% of individuals within 30 days, and with doses higher than 6 Sv, survival is unlikely.
Stochastic Effects
Stochastic (or probabilistic) effects cause DNA damage to single cells, which results in mutation. This is an all-or-none phenomenon, with the probability of occurrence increasing as the cumulative radiation exposure increases without an established threshold level (Fig. 21-4). The severity of the effect of mutation is unrelated to the dose. Mutations lead to cancer and heritable genetic defects. Theoretically, stochastic effects can occur even at low doses, but it is assumed that with radiation doses of less than 100 mSv/yr, the probability of stochastic effects is very low. At such low doses, it is assumed that the probability of incurring cancer or heritable effects will be directly proportional to the equivalent dose. This is known as the linear-nonthreshold model of incremental risk.
Leukemia and other cancers have been shown to be associated with radiation exposure. It is estimated that the probability of fatal cancer developing as a result of radiation exposure is 4% per 1 Sv of lifetime dose equivalent (or 0.004% per mSv). The background risk of spontaneous fatal cancer is around 20%. The nominal nonfatal cancer risk has been estimated at 0.8% per sievert. Radiation-induced cancer risks from relatively low-dose exposure are difficult to interpret. A statistically significant increase in cancer risk has not been demonstrated in populations exposed to doses of less than 100 mSv. Studies of atomic bomb survivors showed an increased incidence of leukemia and other tumors of the lungs, thyroid, breast, skin, and gastrointestinal tract. There is usually a latent period of 2 to 5 years for leukemia, about 5 years for thyroid cancer, and 10 or more years for other cancers to become manifested. The risk with antenatal exposure and for children and adolescents is slightly higher by a factor of 2 to 3, whereas for older adult patients, the relative risk of malignancy is lower. Fetal effects are discussed in the Radiation and Pregnancy section, later in this chapter.
Hereditary effects from radiation exposure have not been observed in humans from studies of the offspring of atomic bomb survivors. Based on extrapolation from animal studies, the ICRP proposed a nominal risk of heritable effects of 0.1% per sievert.
Exposure and Recommended Limits
Background Radiation
We are constantly exposed to radiation through naturally occurring radioactive materials, as well as by cosmic radiation and human activities. The average annual natural background radiation, which varies depending on the geographic location, is around 3 mSv/yr in the continental United States. Living at higher altitude is associated with a higher dose. The greatest source of domestic radiation is radon gas (about 2 mSv/yr). Radon arises from the decay of radium; it seeps out of the soil and may concentrate in poorly ventilated concrete homes because of its high density. Radon is the second-most frequent cause of lung cancer, after cigarette smoking. Other man-made sources include building materials, fuel, televisions, smoke detectors, and various fluorescent devices.
Occupational and Medical Exposure
For the general public, about 80% of lifetime exposure to radiation comes from natural sources and the rest from man-made sources such as medical radiographs and CT scans. The total average exposure for Americans is about 3.6 mSv/yr. In the United Kingdom, the average yearly dose is about 2.2 mSv. Some of the common occupation-related radiation dosages are addressed in Table 21-3. Pilots, who are exposed to solar radiation, are at a higher risk. On average, the amount of cosmic radiation doubles with every 2000-mile increase in altitude; cosmic radiation is strongest at the poles. Taking a long-haul commercial flight results in about 0.1 mSv of radiation.
Table 21-3
Background and Occupational Exposure
Occupation | Effective Dose (mSv/Yr) |
Natural background radiation | 3 |
Aircrew (1000 hr) | 5 |
Frequent flyer (75,000 miles/200 hr) | 1 |
Catheterization laboratory physician | 2-60 |
Catheterization laboratory nurse | 8-16 |
The typical doses of common diagnostic radiology examinations are given in Table 21-4. These values vary, depending on the machine and manufacturer, the study technique, and patient’s build.
Table 21-4
Typical Effective Dose in Common Diagnostic Procedures
Examination | Effective Dose (mSv) |
Chest radiograph (PA) | 0.02 |
Chest radiograph (lateral) | 0.04 |
Skull radiograph | 0.03 |
Pelvic radiograph | 0.7 |
Abdominal radiograph | 1.0 |
Thoracic spine (AP) | 0.4 |
Lumbar spine (AP) | 0.7 |
Mammogram | 0.1 |
Barium swallow | 1.5 |
Barium enema | 7.0 |
CT of the head | 2 |
CT of the thorax | 8 |
CT of the abdomen | 10 |
CT of the pelvis | 10 |
Cardiac CT (calcium score) | 2 |
Coronary angiogram | 5-15 |
AP, Anteroposterior; CT, computed tomography; PA, posteroanterior.
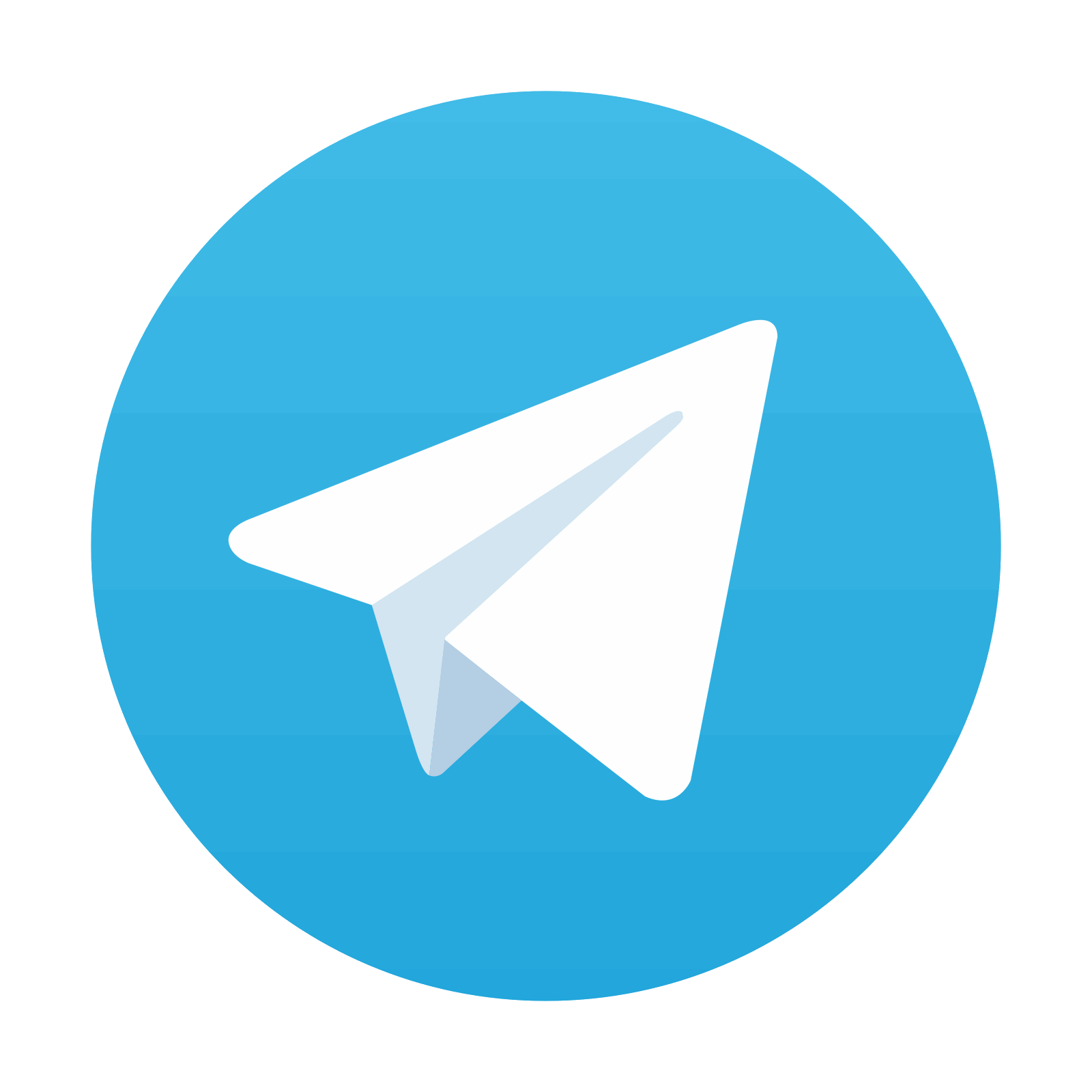
Stay updated, free articles. Join our Telegram channel

Full access? Get Clinical Tree
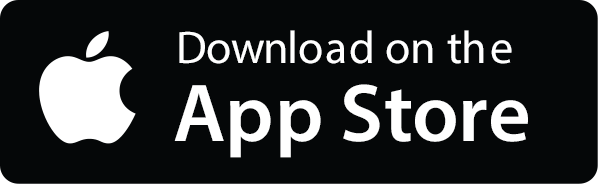
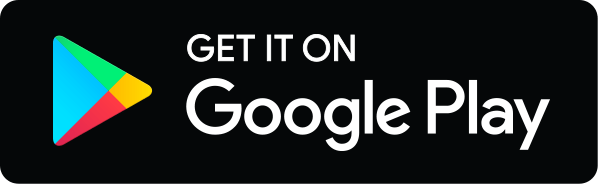