Abstract
Pulmonary vein isolation (PVI) represents the cornerstone of current catheter ablation techniques for the treatment of atrial fibrillation (AF), with significantly improved efficacy compared with antiarrhythmic drug therapy as demonstrated in multiple randomized clinical trials. To achieve PV antrum isolation, multiple approaches with different ablation strategies have been described; the technique currently endorsed by the HRS/EHRA/ECAS expert consensus statement on catheter and surgical ablation of AF consists of antral PVI confirmed by a circular mapping catheter. In this chapter, the techniques and outcomes for different PVI techniques are reviewed.
Keywords
atrial fibrillation, intracardiac echocardiography, nonpulmonary vein triggers, pulmonary vein isolation, radiofrequency ablation
Key Points
- •
Pulmonary vein isolation (PVI) is the cornerstone of current ablation techniques for the treatment of atrial fibrillation (AF).
- •
Confirmation of electrical isolation with a circular mapping catheter is crucial to improve the short- and long-term outcomes of this procedure.
- •
Wide antral PVI improves the long-term arrhythmia-free survival compared with ostial PVI.
- •
PV reconnection is a major contributor to AF recurrences after PVI, especially in patients with paroxysmal AF.
- •
Despite proven PVI, a subset of patients still experiences recurrent arrhythmia, mostly as a result of a higher prevalence of non-PV triggers.
- •
PVI is associated with worse arrhythmia-free survival in patients with nonparoxysmal AF than those with paroxysmal AF
Pulmonary vein isolation (PVI) represents the cornerstone of current catheter ablation techniques for the treatment of atrial fibrillation (AF), with significantly improved efficacy compared with antiarrhythmic drug therapy as demonstrated in multiple randomized clinical trials. After the pivotal demonstration by Haïssaguerre and coworkers that focal discharges from the pulmonary veins (PVs) are implicated in the initiation of AF, empirical PVI has been performed with the highest procedural success in patients with paroxysmal AF, in whom spontaneous PV firing is frequently the only trigger for AF paroxysms. Over the years, PVI techniques have undergone a profound evolution, from initial attempts consisting of focal PV ablation of documented AF triggers within the PV, to the current standard of care, that is empirical ablation targeting the junction between the PVs and the left atrium (LA), and the PV antrum. To achieve PV antrum isolation, multiple approaches with different ablation strategies have been described; the technique currently endorsed by the HRS/EHRA/ECAS expert consensus statement on catheter and surgical ablation of AF consists of antral PVI confirmed by a circular mapping catheter. In this chapter, the techniques and outcomes for different PVI techniques will be reviewed.
Patient Selection and Preprocedural Considerations
Indications for pulmonary vein isolation
Current guidelines for the management of AF give a class I indication with the highest level of evidence to PVI in patients with symptomatic paroxysmal AF, who have failed treatment with at least one class I or III antiarrhythmic drug (second-line treatment). Based on the results of randomized trials, PVI as first-line treatment in patients with no or minimal structural heart disease can be considered when performed in expert centers, where outcomes are comparable with antiarrhythmic drug (AAD) treatment, without an increase in complication rates. To date, there is no indication for PVI to reduce cardiovascular morbidity/mortality or hospitalization.
Antiarrhythmic Drugs
Patients undergoing PVI should have their antiarrhythmic drugs discontinued for at least five half-lives before the ablation procedure to facilitate the identification of AF triggers, especially those outside the PVs that are not empirically targeted. In this regard, although adequate drug washout can be achieved in reasonable time before the procedure for most AADs, patients receiving chronic treatment with amiodarone represent a unique challenge because of the long half-life of the drug, and thus require longer elimination time. There might be an incremental benefit of adequate preprocedural amiodarone wash-out, suggesting that discontinuation of the drug 4 to 6 months before the procedure increases the chance of disclosing latent non-PV trigger sites, resulting in better long-term arrhythmia-free survival.
Anticoagulation Status
A proper anticoagulation status is crucial to minimize the risk of periprocedural thromboembolism and bleeding. In recent times, a substantial body of evidence has been built on the benefit of performing PVI under uninterrupted anticoagulation with both warfarin and the novel oral anticoagulants; this reduces the risk of stroke without increasing the risk of bleeding. Of note, among the novel oral anticoagulants, our experience with uninterrupted dabigatran has been disappointing, with an increase in the risk of bleeding or thromboembolic complications compared with uninterrupted warfarin; therefore we usually prefer to use warfarin or factor Xa inhibitors in the periablation setting. Another important strategy to minimize the risk of periprocedural stroke is to administer an intravenous heparin bolus (usually 100 U per kg up to 10.000 U with warfarin, or 12.000 to 15.000 U with factor Xa inhibitors) right after vascular access is obtained. The goal is to achieve full anticoagulation (goal activated clotting time [ACT] 350–500 seconds) before transseptal access, thus reducing the chance of thrombus formation when sheaths and catheters are in the LA.
Anesthesia Protocol
The anesthesia protocol adopted during PVI can also affect the procedural results. Although moderate to deep sedation/anesthesia can be employed, general anesthesia, with or without high-frequency jet ventilation, has the important advantage of controlling respiration by eliminating deep breathing. This improves catheter stability, leading to more effective RF energy delivery, thus reducing procedural times and improving long-term outcomes compared with conscious sedation.
Vascular and left atrial access
To reduce vascular complications, central venous access should be obtained with real-time ultrasound guidance; this reduces the number of attempts, time to access, and risk of arterial puncture. Moreover, an arterial line for hemodynamic monitoring should not be placed routinely, given the higher risk of hematomas in anticoagulated patients. Noninvasive intermittent blood pressure monitoring every 2 to 5 minutes is generally sufficient. For LA access, two separate transseptal punctures are performed (one for the ablation catheter, the other for the circular mapping catheter [CMC]), preferably under intracardiac echocardiography (ICE) guidance. The goal is to cross into the LA along the posterior interatrial septum, such that the left-sided PVs are in clear view ( Fig. 14.1 ; ). Although a second transseptal sheath can be introduced via wire exchange, a separate transseptal puncture minimizes sheath-to-sheath interaction. LA access can be obtained using a variety of transseptal sheaths. In our center we prefer sheaths with a moderate primary curve and no secondary curve (such as the SL-0 for the ablation catheter and a LAMP-90 for the circular mapping catheter), which allow easier catheter maneuverability in the posterior aspect of the LA.

Techniques and Results of Pulmonary Vein Isolation
Triggers from the PVs represent the dominant mechanism of initiation of AF. The initial description of PV trigger ablation consisted of focal ablation within the PVs. This approach had minimal long-term benefit and was associated with a significant risk of PV stenosis. Subsequent evolution of the procedure involved segmental ablation at the anatomic ostium of the PVs, as defined by angiography or ICE, to isolate muscle sleeve connections between the PV and LA electrically, an approach commonly referred to as segmental ostial PVI. In addition to ostial PVI, initial attempts targeted only the PVs with evidence of arrhythmogenic activity. However, it was rapidly realized that empirical isolation of all PVs was necessary to increase the procedural success, as the majority of patients presented multiple arrhythmogenic PVs. In addition, symptomatic PV stenosis remained an important risk with ostial PVI, and AF triggers localized in the more proximal antral region were not addressed with such strategy. The technique further evolved targeting the LA tissue more proximal to the PV ostium, in a region defined as the PV antrum.
To achieve antral PVI, multiple approaches have been described. The approach currently adopted in our institution is a wide PV antrum isolation (encompassing the LA posterior wall between the PVs) guided by a CMC and ICE ( Fig. 14.2 ). The importance of a wider antral isolation is the empirical elimination of triggers arising from the LA posterior wall, which should be considered as an extension of the PVs from an embryologic, anatomic, and electrophysiologic standpoint. Compared with ostial PVI, wide antral PVI is associated with a significantly lower rate of arrhythmia recurrence, driven by a reduced rate of AF at follow-up without increasing the rate of periprocedural complications. This approach has been demonstrated to be superior to other ablation techniques in studies of direct comparison, and is one of the most reproducible and standardized techniques to achieve PVI. Circumferential PV ablation without confirmation of electrical isolation with a CMC has been demonstrated to be ineffective in achieving long-term arrhythmia control. Moreover, adopting a CMC-guided approach also offers the potential advantages of shortening the procedural time, limiting the delivery of RF energy to discrete areas showing the earliest potentials (segmental approach). By contrast, in a circumferential PV ablation approach, contiguous lesions are created without necessarily achieving complete conduction block, and postincisional reentrant atrial tachycardias are not uncommon.

Although not necessary, the use of a 3-dimensional electroanatomic mapping system, such as EnSite NavX Velocity/Precision (St. Jude Medical, St. Paul, Minn) and CARTO 3 (Biosense Webster, Diamond Bar, CA) can facilitate PVI significantly, allowing efficient registration of ablation sites and documentation of the extent of antral ablation ( Fig. 14.3 ). They allow real-time display of any cardiac catheter during ablation; most importantly, their ability to display the CMC allows for very rapid creation of a LA volume map and facilitates the direction of the ablation catheter toward the desired poles. While maneuvering the CMC, care should be taken not to displace it anteriorly, as this can result in its entrapment in the mitral valve apparatus, a rare complication that might result in valve damage requiring open heart surgery. The use of ICE during mapping facilitates locating the four PVs ( ). The left-sided PVs can be seen with clockwise rotation of the ICE catheter a few degrees past the left atrial appendage (LAA) and the mitral valve annulus, whereas to image the right PVs, the ICE catheter is advanced slightly and further rotated clockwise. Moreover, ICE has been shown to reduce PV stenosis, compared with PV angiography. The LAA can be recognized by a high amplitude signal on the superior-anterior aspect of the LA.

The CMC is used as a roving catheter for mapping and directing the ablation catheter throughout the procedure ( Fig. 14.4 ). It should be small enough to move within the chamber easily, but of a large enough diameter that it does not readily fall deep inside the PVs. In most adults, a 20-mm, 10-pole CMC provides a balance between maneuverability and size. RF energy delivery with open-irrigation catheters is the standard of care in performing PVI. Open-irrigation catheters allow the use of greater power without a significant increase in temperature and clot formation, enabling more efficient and predictable energy delivery, resulting in larger ablation lesion sets. The main disadvantage of using open irrigation catheters is a higher risk of steam pops. There is a high discrepancy between the recorded tip temperature and the actual tissue temperature, thus steam pops might occur even with normal tip temperature. These can be minimized with appropriate titration of energy delivery, as guided by monitoring tip temperature, impedance drop, and if available, contact force. Our catheter of choice is a unidirectional open-irrigated 3.5-mm tip with an F curve. A smaller curve may be considered in the minority of patients with a very small LA, whereas a J curve is necessary for patients with a larger LA.

Using an irrigation-tip catheter, a power of 40 W is typically used. Occasionally, on the anterior aspect of the PVs (for example, a thick ridge), a higher power is needed to achieve potential abatement, but we generally do not exceed 45 W. With contact force sensing ablation catheters, this can be obtained by optimizing contact during radiofrequency (RF) energy delivery (at 40 W, our target contact force lies between 7 and 15 g). RF energy is delivered for approximately 20 seconds per lesion site or until the electrical signals have diminished. In areas near the esophagus, energy delivery should be limited to up to 10 seconds per lesion site, and keep moving the catheter to different areas during ablation to prevent esophageal heating. It is very important to monitor the esophageal temperature closely, frequently readjusting the esophageal probe so that it is close to the ablation area. A rapid rise in temperature is often noted, and when this happens, the ablation catheter should be moved away or RF delivery stopped, independently of the absolute value of esophageal temperature recorded. Despite movement of the catheter, a delayed rise in esophageal temperatures is often observed. As a general rule, esophageal temperatures greater than 38°C to 39°C should be avoided to minimize the risk of deep tissue injury. It is also important to note that the esophagus is wider than the temperature probe ( Fig.14.5 ); therefore sometimes no or minimal temperature change is recorded, despite ablating over the esophagus, as assessed by ICE ( Fig. 14.6 ). If ICE is not available, it is important to move quickly whenever ablating in the posterior wall regardless of the location of the esophageal probe.


We usually begin with ablation of the electrical potentials surrounding each PV. The end point of PVI is complete PV electrical isolation, as confirmed by entrance and exit block ( Table 14.1 ). Entrance block is confirmed by the absence of electrical signals where these signals had been previously observed. Occasionally, dissociated PV potentials can be recorded ( Fig. 14.7 ) to confirm exit block. In addition, pacing from within the PV can be performed to confirm exit block, although this is not necessary. Once PVI is achieved, we focus on the remaining antral areas. The CMC is particularly useful for identifying additional electrical potentials along the posterior wall and roof of the LA (see Fig. 14.4 ). The procedural end point is to eliminate all electrical activity in the antrum and roof of the LA (see Figs. 14.2 & 14.8 ). After PV antral isolation, with patients in sinus rhythm, 20 to 30 mcg per kg per minute of isoproterenol are infused to assess for PV reconnection and elicit non-PV triggers. If consistent (repetitive premature atrial contractions [PACs], PACs triggering atrial flutter/AF, and focal atrial tachycardia), their sites of origin are further targeted with ablation. We do not routinely use adenosine to test for acute PVI.
Definition | Pros | Cons | |
---|---|---|---|
Entrance block | Elimination of all the potentials within the PV antrum, as assessed by a circular mapping catheter | Tested in multiple clinical studies | Rare cases of unidirectional entrance block have been described |
Exit block | Failure to conduct from within the PV antrum to the atrium | Identifies bidirectional conduction block across the PV antrum | Not validated in large studies |
Voltage reduction | Reduction of local voltages after circumferential PV ablation | — | Not equivalent to PVI, which has to be confirmed with a circular mapping catheter. Multiple randomized trials have demonstrated the ineffectiveness of this end point |
Termination of AF | Restoration of sinus rhythm (or conversion to intermediate AT) during ablation | When achieved in the process of PVI (when the isolated PV remains in AF), proves the critical participation of the PV as a trigger for AF | Contradictory clinical data do not support the use of this end point during AF ablation |


As mentioned, the majority of the studies investigating the role of PVI for the long-term maintenance of sinus rhythm have predominantly enrolled patients with paroxysmal AF; thus far the role of PV triggers in patients with nonparoxysmal AF has not been investigated in a systematic fashion. In addition, few data support the benefit of PVI in paroxysmal patients, also at the very long-term follow-up. However, the authors’ experience suggests that some of the patients will develop non-PV triggers over times that are responsible for arrhythmia recurrence at long term. The clinical experience with PVI in patients with nonparoxysmal AF has consistently shown worse results compared with paroxysmal patients, and PVI alone is largely insufficient to achieve satisfactory long-term arrhythmia-free survival in this cohort. Thus far the approach of most institutions is to provide extensive ablation targeting the LA substrate and areas demonstrating non-PV triggers, either spontaneously or after induction with drug challenges (e.g., isoproterenol) or pacing. However, the amount of additional ablation necessary in these patients to increase the procedural success is still a matter of controversy and goes beyond the scope of this chapter.
Complications of Pulmonary Vein Isolation
Because patients are anticoagulated, the most common complications are vascular (hematomas, pseudoaneurysm, and arteriovenous fistulas). These can be easily prevented using real-time ultrasound when obtaining central venous access.
Hemodynamic and temperature monitoring during the ablation procedure allows early detection and avoidance of the most serious complications of PVI: cardiac tamponade and atrial-esophageal fistula. A blood pressure drop, both sudden and gradual, should be evaluated carefully. A vagal response to ablation near the ganglionic plexi may result in transient sudden hypotension, usually associated with bradycardia (sinus arrest or atrioventricular block). If there is no immediate recovery, cardiac tamponade should be assumed until proven otherwise. Suspicion should be higher after a difficult transseptal puncture, a steam pop, or inadvertent application of excess pressure in the LAA or the roof of the LA. To determine early diagnosis of cardiac tamponade, it is important not to delay pericardiocentesis. A quick assessment to check for the presence of pericardial effusion can be easily and accurately performed with ICE ( ). If this is not available, fluoroscopy usually demonstrates a reduction in the excursion of the cardiac silhouette, better appreciated in the LAO view. Of note, especially after extensive ablation, it is not uncommon to detect mild pericardial effusion without hemodynamic compromise following the procedure. Most of these asymptomatic effusions can be managed conservatively.
PVI involves extensive burning near the esophagus, and atrial-esophageal fistulas can present in the weeks following ablation. Although exceedingly rare, they are frequently fatal; therefore prevention and early diagnosis/treatment (surgical repair) are important to reduce mortality. During ablation, temperature monitoring as well as limitation of the duration of energy delivery when ablating in the posterior aspect of the LA (down to the CS) is vital to avoid excessive heating of the esophagus. A short course of prophylactic proton pump inhibitors and sucralfate can be prescribed. During follow-up, patient and physician awareness is the key. Patients should be instructed to immediately contact the electrophysiology service if fever, chest pain, neurologic symptoms, dysphagia, hematemesis, or melena occur.
Thermal injury can also result in phrenic nerve (PN) injury. This can be right sided when ablating in the right superior PV or performing isolation of the superior vena cava (SVC), or left sided when ablating inside the LAA. To prevent PN injury, it is important to avoid ablation inside the PVs or LAA, and to perform PN mapping when isolating the SVC. Patients with PN paralysis are usually asymptomatic, but they can present with dyspnea on exertion. There is no treatment, but the condition is usually transient, so observation is all that is needed. Dyspnea, with or without cough or hemoptysis, is also a presenting symptom of severe PV stenosis, which is a rare occurrence after true PVI, because RF energy is not delivered ostial or inside the PVs. PV stenosis is diagnosed with CT imaging, and if severe or symptomatic might require intervention (venoplasty and stenting).
To reduce periprocedural thromboembolic events, the procedure should be performed under uninterrupted oral anticoagulation and heparin administered transseptal access with goal ACT 350 seconds or more. To prevent air embolism, transseptal sheaths should be continuously flushed with 2 to 4 mL per minute of heparinized-saline using pressure bags set at 300 to 400 mm Hg.
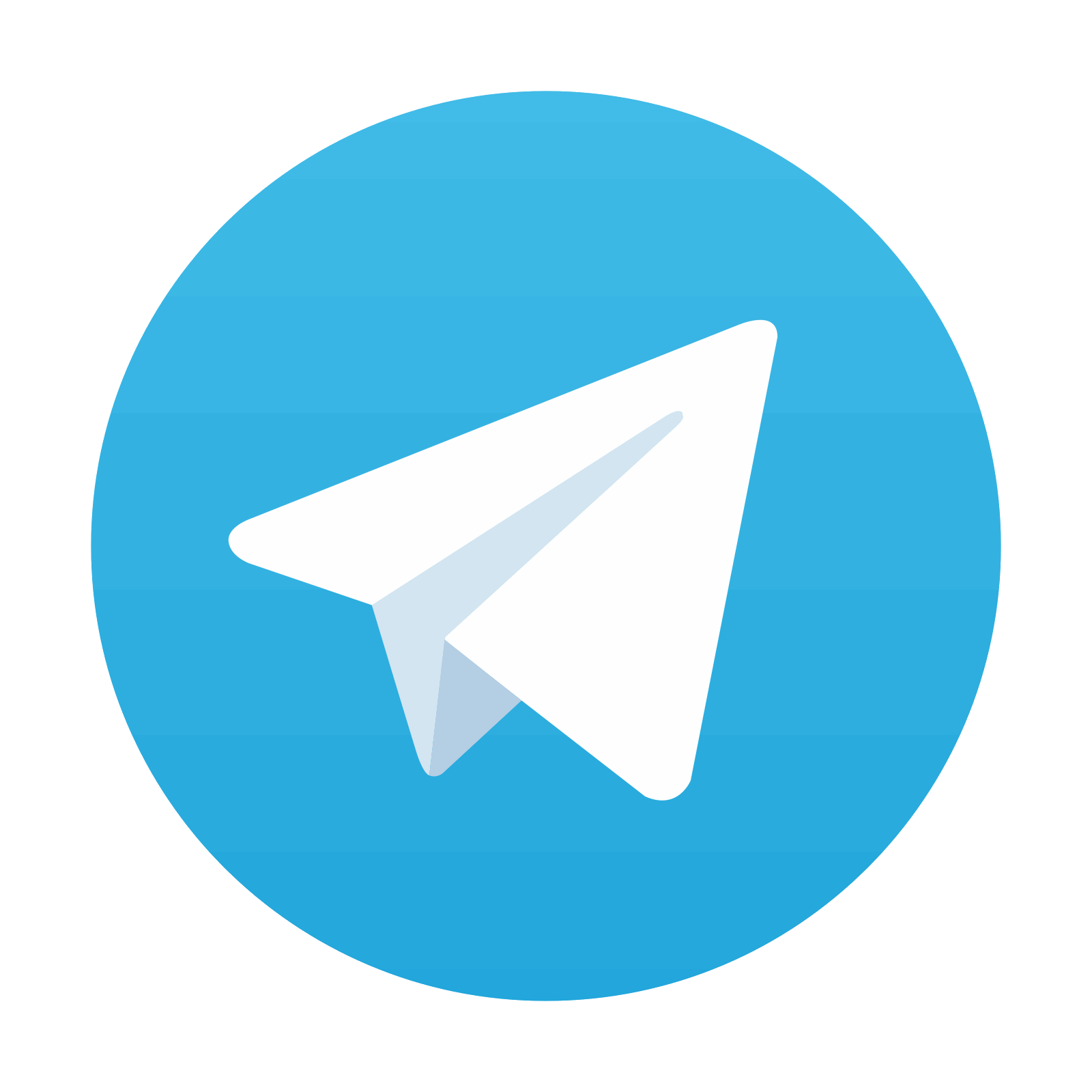
Stay updated, free articles. Join our Telegram channel

Full access? Get Clinical Tree
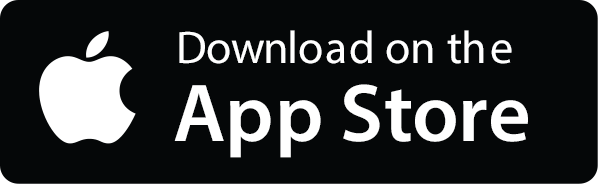
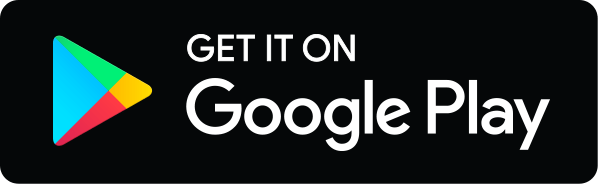